What State Of Matter Is Fire
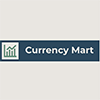
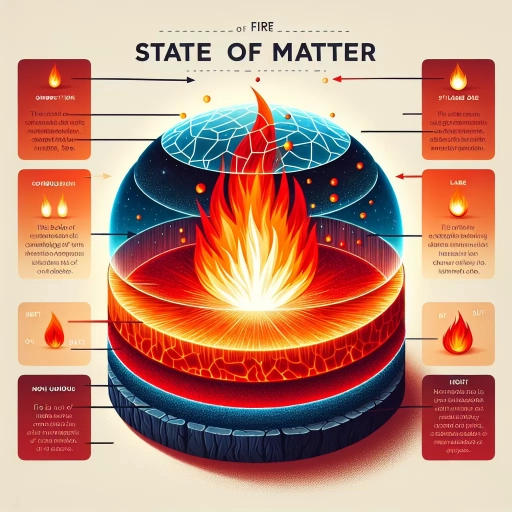
Fire, a phenomenon that has captivated human imagination for centuries, is often misunderstood in terms of its classification as a state of matter. While it is commonly perceived as a distinct entity, the scientific community has delved deeper into its nature to determine whether it fits into the traditional categories of solid, liquid, or gas. This article aims to explore the multifaceted aspects of fire, beginning with an in-depth look at **Understanding the Nature of Fire**, where we will dissect the chemical and physical processes that define it. We will then proceed to **Classifying Fire as a State of Matter**, examining the arguments for and against categorizing fire within the conventional states of matter. Finally, we will delve into **Scientific Perspectives on Fire's State**, where various scientific viewpoints and theories will be discussed to provide a comprehensive understanding. By navigating these perspectives, we can gain a clearer insight into what fire truly is and how it should be classified. Let us start by understanding the fundamental nature of fire.
Understanding the Nature of Fire
Fire, a fundamental element of human existence, has captivated and intimidated us for centuries. Its mesmerizing dance of flames and its ability to both create and destroy have made it a subject of fascination and study. To truly understand the nature of fire, it is essential to delve into its core components and behaviors. This article will explore three critical aspects that define fire: the chemical composition that fuels its existence, the thermal energy and heat transfer mechanisms that sustain it, and the physical properties of flames that characterize its appearance and behavior. By examining these elements, we can gain a deeper insight into what fire is, how it operates, and why it remains such a powerful force in our lives. Understanding the nature of fire is not just about appreciating its beauty or respecting its power; it is about grasping the underlying principles that make it possible. In this article, we will break down these complex concepts to provide a comprehensive understanding of fire's intricate nature.
Chemical Composition of Fire
Fire, often misunderstood as a state of matter, is actually a chemical reaction known as combustion. The chemical composition of fire involves a complex interplay of elements and compounds that facilitate this process. At its core, fire is the result of an exothermic reaction between a fuel source and an oxidizer, typically oxygen from the air. This reaction releases heat, light, and various products of combustion. The fuel can be any combustible material such as wood, gasoline, or propane, each with its own unique chemical structure. For instance, wood is primarily composed of cellulose and lignin, while gasoline is a mixture of hydrocarbons. When these fuels are heated to their ignition temperature, they decompose into volatile gases that react with oxygen in the air. This reaction is known as oxidation and results in the formation of carbon dioxide (CO₂), water vapor (H₂O), and other gases depending on the fuel's composition. The process can be simplified into four stages: ignition, combustion, sustainment, and extinction. Ignition occurs when the fuel reaches its flash point, initiating the chemical reaction. Combustion is the actual burning process where fuel reacts with oxygen to produce heat and light. Sustainment happens as long as there is sufficient fuel and oxygen to continue the reaction. Finally, extinction occurs when one or more of these elements are removed. During combustion, various intermediate species are formed, including free radicals and excited molecules that emit light across different wavelengths, giving fire its characteristic colors. For example, the yellow color often seen in flames is due to incandescent particles of soot and other unburned carbon particles. The blue color at the base of a flame is typically from excited carbon dioxide molecules. Understanding the chemical composition of fire is crucial for managing and controlling it. Firefighters use this knowledge to select appropriate extinguishing agents that can disrupt one or more of the necessary elements for combustion—fuel, oxygen, or heat. Similarly, engineers design fire-resistant materials and systems that can withstand high temperatures and prevent ignition. In summary, fire is not a state of matter but a dynamic chemical process involving complex interactions between fuels and oxidizers. Its composition includes various gases and intermediate species that contribute to its appearance and behavior. Recognizing these components is essential for comprehending how fire works and how it can be managed effectively. This understanding underscores the importance of chemistry in explaining natural phenomena like fire.
Thermal Energy and Heat Transfer
Thermal energy and heat transfer are fundamental concepts that underpin our understanding of fire, a complex phenomenon that involves the transformation of chemical energy into thermal energy. Thermal energy, the total internal kinetic and potential energy of the particles in a substance, is a measure of the motion and interaction of these particles. When a substance undergoes combustion, as in the case of fire, chemical bonds are broken and reformed, releasing a significant amount of thermal energy. This process is known as exothermic, meaning it releases heat. Heat transfer, the movement of thermal energy from one body to another due to a temperature difference, plays a crucial role in sustaining fire. There are three primary modes of heat transfer: conduction, convection, and radiation. Conduction occurs through direct contact between particles, allowing heat to spread within a solid or between solids in contact. Convection involves the movement of heated fluids (gases or liquids) that rise and are replaced by cooler fluids, creating a continuous cycle that helps distribute heat. Radiation is the transfer of heat through electromagnetic waves and does not require a medium to propagate. In the context of fire, all three modes of heat transfer are at play. For instance, conduction helps ignite adjacent materials when they come into contact with the hot flames. Convection currents are evident in the rising plumes of smoke and heated air that carry heat away from the fire source, contributing to its spread. Radiation from the fire can also ignite flammable materials at a distance, even if they are not in direct contact with the flames. Understanding these mechanisms is essential for comprehending how fire behaves and how it can be controlled or extinguished. For example, smothering a fire by depriving it of oxygen disrupts the combustion process, while using water to cool the fuel can reduce the temperature below the ignition point, preventing further heat transfer and thus extinguishing the fire. In summary, thermal energy and heat transfer are integral to the nature of fire. The release of thermal energy during combustion fuels the fire, while the various modes of heat transfer facilitate its spread and sustain its intensity. Recognizing these principles not only enhances our understanding of fire but also informs strategies for managing and mitigating its impact.
Physical Properties of Flames
Fire, often misunderstood as a state of matter, is actually a chemical reaction known as combustion. The physical properties of flames are crucial in understanding this complex process. A flame consists of several distinct zones, each with its own set of characteristics. At the base of the flame, there is the **innermost zone**, where fuel and oxygen mix but do not yet react, creating a dark or blue region due to the absence of light emission. Moving outward, the **intermediate zone** is where the actual combustion occurs; here, fuel reacts with oxygen to produce heat and light, typically emitting a yellow or orange color due to incandescence from soot particles. The **outermost zone**, or the tip of the flame, is where complete combustion takes place, characterized by a blue color indicating high temperatures and efficient burning. The **temperature** of a flame varies significantly across these zones. The hottest part of the flame is usually at the tip, where temperatures can reach up to 1800°C (3272°F) in well-oxygenated conditions. This high temperature is a result of the exothermic reaction releasing a substantial amount of energy. The color of the flame also provides insight into its temperature; blue flames are hotter than yellow or orange ones because they indicate more complete combustion and higher energy release. Another important physical property is **luminosity**. Flames emit light due to incandescence and chemiluminescence. Incandescence occurs when soot particles in the intermediate zone heat up and glow, producing the yellow and orange hues. Chemiluminescence, on the other hand, involves the emission of light by excited molecules as they return to their ground state after reacting with oxygen. This process contributes to the blue color seen in hotter parts of the flame. The **shape and size** of a flame can be influenced by factors such as airflow and fuel type. In still air, a flame tends to be teardrop-shaped due to buoyancy effects that cause hot gases to rise. However, in windy conditions or with forced air supply, flames can become elongated or even turbulent. Different fuels also produce flames of varying sizes and shapes; for example, a gas flame might be smaller and more controlled compared to a wood fire. Understanding these physical properties of flames not only enhances our appreciation for the complexity of fire but also has practical applications in fields like engineering, where designing efficient combustion systems is crucial for energy production and safety. By recognizing how different zones within a flame contribute to its overall behavior, we can better manage and utilize fire in various contexts, from industrial processes to everyday life. This knowledge underscores that fire, while often seen as a simple phenomenon, is actually a sophisticated interplay of chemical reactions and physical processes.
Classifying Fire as a State of Matter
Fire, often misunderstood as merely a chemical reaction, is a complex phenomenon that challenges traditional classifications of states of matter. While it exhibits characteristics distinct from solids, liquids, and gases, fire also shares some intriguing similarities with these states. This article delves into the multifaceted nature of fire, comparing its properties with those of solid, liquid, and gas states to highlight both the parallels and the divergences. Additionally, we explore the concept of plasma, the fourth state of matter, and how fire's behavior aligns with or deviates from this category. By examining fire's unique characteristics—such as its ability to sustain itself through continuous chemical reactions and its dynamic, ever-changing form—we aim to provide a comprehensive understanding of what constitutes this enigmatic state. Through this analysis, we will transition to a deeper exploration of the nature of fire, shedding light on its fundamental properties and why it stands apart yet remains connected to the traditional states of matter. Understanding the nature of fire is crucial for appreciating its role in various scientific and everyday contexts, and this article seeks to illuminate this fascinating subject.
Comparison with Solid, Liquid, and Gas States
When considering the classification of fire as a state of matter, it is essential to compare and contrast it with the traditional solid, liquid, and gas states. Unlike solids, which maintain a fixed shape and volume due to the rigid structure of their particles, fire does not possess a definite shape or volume. Instead, fire spreads and changes shape based on the availability of fuel and oxygen, much like a gas. However, unlike gases, which are composed of molecules that are widely spaced and free to move in any direction, fire is not a substance but rather a chemical reaction involving the rapid oxidation of fuel sources. This process releases heat, light, and various products of combustion. In contrast to liquids, which take the shape of their container but retain their volume, fire does not adhere to these characteristics. Liquids are made up of particles that are close together but still able to move past one another, allowing for fluidity. Fire, on the other hand, is more akin to a dynamic process than a static state; it involves the continuous interaction between fuel molecules and oxygen molecules in the air. This interaction results in the release of energy in the form of heat and light, which are not properties inherent to any single state of matter but rather outcomes of the combustion process. Moreover, while solids, liquids, and gases can be transformed from one state to another through changes in temperature or pressure (e.g., melting ice from solid to liquid), fire cannot be transformed into another state because it is not a substance that exists independently. Instead, fire ceases to exist when the fuel is depleted or when oxygen is removed from the environment. This distinction highlights that fire is fundamentally different from the traditional states of matter; it is an event or process rather than a material entity. In summary, while fire shares some superficial similarities with gases in terms of its ability to spread and change shape, it does not fit neatly into any of the traditional categories of solid, liquid, or gas. Its nature as a chemical reaction rather than a physical substance sets it apart from these states of matter. Therefore, classifying fire as a state of matter would require a broader definition that encompasses dynamic processes and chemical reactions rather than just physical properties alone.
Plasma: The Fourth State of Matter
Plasma, often referred to as the fourth state of matter, is a unique and fascinating phase that differs significantly from solid, liquid, and gas. Unlike these traditional states, plasma is characterized by the presence of ions and free electrons, which are not bound to any particular atom or molecule. This ionized gas state is achieved when a gas is heated to extremely high temperatures, typically above 10,000 Kelvin, causing the atoms to lose their electrons and form a collection of charged particles. In this state, the plasma behaves more like a fluid than a gas due to its high energy and mobility. The ions and electrons in plasma are highly responsive to electromagnetic fields, making it an excellent conductor of electricity. This property is crucial in various technological applications such as plasma TVs, fluorescent lights, and even some types of medical treatments. The existence of plasma is not confined to laboratory settings; it is abundant in nature. Stars, including our Sun, are massive balls of plasma where nuclear fusion reactions occur. Lightning is another example of natural plasma, formed when electrical discharges ionize the air molecules in the atmosphere. Even the aurora borealis (northern lights) and aurora australis (southern lights) are manifestations of plasma interacting with Earth's magnetic field. Understanding plasma is essential for advancing various fields such as astrophysics, materials science, and engineering. For instance, plasma etching is a critical process in semiconductor manufacturing, allowing for the precise removal of material layers. Additionally, plasma research has led to significant advancements in fusion energy, which holds promise as a clean and sustainable energy source. When considering whether fire can be classified as a state of matter, understanding plasma becomes particularly relevant. Fire involves chemical reactions that release heat and light, often producing ionized gases that can be considered plasma. However, fire itself is more complex and dynamic than a single state of matter; it encompasses elements of both gas and plasma depending on the conditions. In summary, plasma represents a distinct state of matter with unique properties that set it apart from solids, liquids, and gases. Its presence in both natural phenomena and technological applications underscores its importance across various scientific disciplines. As we delve into the classification of fire as a state of matter, recognizing the role of plasma within these processes provides a deeper understanding of the intricate mechanisms at play.
Fire's Unique Characteristics
Fire, often misunderstood as a state of matter itself, is actually a chemical reaction known as combustion. This process involves the rapid oxidation of fuel sources, typically in the presence of oxygen, which releases heat, light, and various products of combustion. One of the unique characteristics of fire is its ability to sustain itself through a continuous cycle of fuel consumption and heat generation. Unlike solid, liquid, or gas states of matter, fire does not have a fixed shape or volume; instead, it spreads and adapts based on the availability of fuel and oxygen. The visual and thermal properties of fire are also distinct. The flames produced by combustion can range in color from yellow to blue, depending on the temperature and chemical composition of the burning material. For instance, higher temperatures tend to produce blue flames, while lower temperatures yield yellow or orange hues. Additionally, fire emits light across a wide spectrum, making it visible even in low-light conditions. The heat generated by fire can be intense, capable of melting metals and causing significant structural damage. Another characteristic that sets fire apart is its dynamic nature. Unlike static states of matter, fire is inherently dynamic and can change rapidly in response to environmental factors such as wind, humidity, and the presence of combustible materials. This dynamism makes fire both powerful and unpredictable, requiring careful management to control its spread and intensity. From a scientific perspective, classifying fire as a state of matter would be inaccurate because it does not possess the defining attributes of solid, liquid, or gas states. Instead, fire is best understood as a complex chemical reaction that involves the interaction of various substances under specific conditions. This understanding highlights the importance of recognizing fire's unique characteristics and treating it as a distinct phenomenon rather than attempting to categorize it within traditional states of matter. In conclusion, while fire exhibits some properties that might seem akin to those of solid, liquid, or gas states—such as occupying space and producing physical effects—it remains fundamentally different due to its nature as a chemical reaction. Recognizing these unique characteristics not only aids in understanding fire's behavior but also underscores why it should be considered separately from traditional states of matter. This nuanced view is crucial for developing effective strategies in fields such as firefighting, engineering, and environmental science.
Scientific Perspectives on Fire's State
Fire, a fundamental element in human history, has long been a subject of fascination and study. From ancient myths to modern scientific inquiry, our understanding of fire has evolved significantly over time. This article delves into the scientific perspectives on fire's state, exploring three key areas that have shaped our current knowledge. First, we examine the historical understanding and misconceptions about fire, highlighting how early theories often blurred the lines between myth and science. Next, we discuss the modern scientific consensus, which has clarified fire's chemical and physical properties through rigorous research. Finally, we delve into experimental evidence and observations that have further refined our comprehension of fire's behavior and characteristics. By navigating these historical, theoretical, and empirical dimensions, we aim to provide a comprehensive overview that ultimately leads to a deeper understanding of the nature of fire.
Historical Understanding and Misconceptions
Historical understanding and misconceptions about fire's state of matter have evolved significantly over time, reflecting the gradual refinement of scientific knowledge. In ancient times, fire was often considered a fundamental element, alongside earth, water, and air, as proposed by philosophers such as Aristotle. This elemental view persisted well into the Middle Ages, where fire was seen as a distinct substance with its own properties rather than a process or reaction. The concept of fire as an element was deeply ingrained in alchemical theories and early scientific thought. However, with the advent of modern chemistry in the 17th and 18th centuries, scientists began to challenge these traditional views. Antoine Lavoisier's discovery of oxygen in 1778 marked a pivotal moment in understanding combustion processes. Lavoisier demonstrated that fire is not an element but rather a chemical reaction involving the rapid oxidation of fuel sources. This breakthrough led to a more nuanced understanding of fire as a dynamic process rather than a static substance. Despite these advancements, misconceptions about fire's nature persisted in popular culture and even in some educational contexts. For instance, many people still refer to fire as if it were a tangible object or substance rather than recognizing it as a complex series of chemical reactions involving heat, light, and gases. This misunderstanding can be attributed to the intuitive yet misleading appearance of flames, which seem to have a life of their own. From a scientific perspective, fire is best described as a self-sustaining exothermic reaction that involves the oxidation of fuel sources. This process releases heat and light, creating the visible flames we associate with fire. The state of matter in this context is not fire itself but rather the gases and particles involved in the combustion process—primarily hot gases like carbon dioxide, water vapor, and unburned particles. Understanding these scientific perspectives on fire is crucial for dispelling historical misconceptions and fostering a more accurate comprehension of this fundamental phenomenon. By recognizing fire as a chemical reaction rather than an element or substance, we can better appreciate its role in various natural and industrial processes. This nuanced understanding also underscores the importance of continued scientific inquiry in refining our knowledge and correcting long-standing misconceptions about the natural world. Ultimately, the evolution from ancient elemental theories to modern chemical explanations highlights the dynamic nature of scientific knowledge and its capacity to illuminate even the most seemingly familiar phenomena.
Modern Scientific Consensus
Modern scientific consensus on the state of matter of fire is rooted in a deep understanding of thermodynamics, chemistry, and physics. Fire, often perceived as a distinct state of matter, is actually a complex process involving chemical reactions and thermal energy. According to contemporary scientific perspectives, fire is not classified as one of the traditional states of matter—solid, liquid, or gas—but rather as a manifestation of rapid oxidation reactions, typically involving hydrocarbons and oxygen. These reactions release heat and light, creating the visible flames and warmth associated with fire. The consensus among scientists is that fire is a dynamic, nonequilibrium process rather than a static state. It involves the breakdown of molecules into simpler compounds, releasing energy in the form of heat and light. This process is known as combustion, and it requires three essential elements: fuel (the substance being burned), oxygen (or another oxidizer), and heat (to initiate and sustain the reaction). The products of combustion include carbon dioxide, water vapor, and other gases, which are released into the atmosphere. From a thermodynamic standpoint, fire represents a significant increase in entropy due to the conversion of chemical energy into thermal energy. This process is irreversible under normal conditions, aligning with the second law of thermodynamics. The visible flames are primarily composed of hot gases and soot particles that emit light as they cool down. Chemically, fire involves exothermic reactions where fuel molecules react with oxygen to form carbon dioxide and water. These reactions are highly energetic and release substantial amounts of heat, which in turn sustain the combustion process. The color and intensity of flames can vary based on the temperature and composition of the burning material, reflecting different wavelengths of light emitted by excited atoms or molecules. In summary, modern scientific consensus views fire not as a distinct state of matter but as a dynamic chemical and thermal process. This understanding underscores the importance of considering fire within the context of chemical reactions and thermodynamic principles rather than categorizing it alongside solids, liquids, and gases. This nuanced perspective enhances our comprehension of fire's behavior and its role in various natural and industrial processes.
Experimental Evidence and Observations
Experimental evidence and observations play a crucial role in understanding the state of matter that fire represents. From a scientific perspective, fire is not considered a state of matter in the traditional sense, which includes solid, liquid, and gas. Instead, it is a chemical reaction known as combustion. This process involves the rapid oxidation of fuel sources, typically organic materials, in the presence of oxygen. Observations from experiments highlight several key characteristics that distinguish fire from other states of matter. For instance, when a substance undergoes combustion, it releases heat and light, which are indicative of the exothermic nature of the reaction. This is evident in the visible flames and warmth emitted by fires. Additionally, the products of combustion, such as carbon dioxide and water vapor, are gases that disperse into the atmosphere, further supporting the notion that fire is not a distinct state but rather a transformative process. Experimental setups have been designed to study various aspects of fire behavior. For example, controlled laboratory experiments using combustion chambers allow scientists to measure the rates of reaction, temperatures achieved during combustion, and the composition of exhaust gases. These studies provide valuable insights into the kinetics and thermodynamics of fire, reinforcing its classification as a chemical reaction rather than a state of matter. Moreover, observations from real-world scenarios, such as wildfires or industrial fires, corroborate laboratory findings. The dynamic behavior of fires in these contexts—such as spreading patterns, flame heights, and smoke plumes—can be explained by principles of chemistry and physics rather than properties unique to a specific state of matter. This empirical evidence underscores that fire's characteristics are derived from the interactions between reactants and environmental conditions rather than inherent properties of a distinct state. In conclusion, experimental evidence and observations collectively support the scientific perspective that fire is not a state of matter but a complex chemical reaction involving combustion. By understanding fire through this lens, researchers can better predict its behavior, develop strategies for control and prevention, and enhance our overall comprehension of this multifaceted phenomenon. This nuanced understanding is essential for advancing both theoretical knowledge and practical applications related to fire science.