What Is A Solute
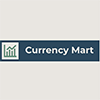
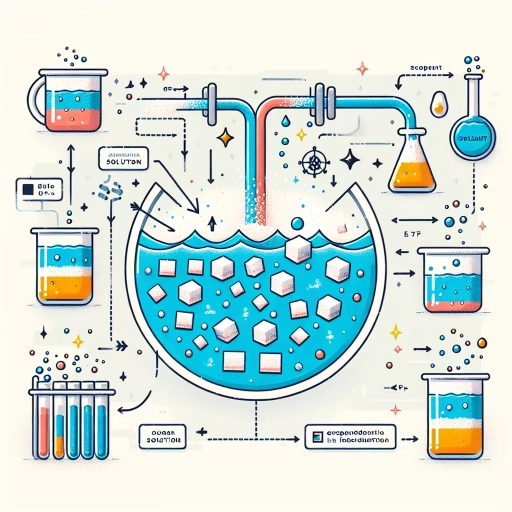
In the realm of chemistry, the concept of a solute is fundamental to understanding the nature of solutions. A solute is a substance that dissolves in a solvent to form a solution, but its significance extends far beyond this simple definition. To fully grasp the role and importance of solutes, it is essential to delve into their definition and concept, explore the various types and classifications, and examine their behavior and interactions within solutions. This article will provide a comprehensive overview, starting with the **Definition and Concept of a Solute**, where we will explore what constitutes a solute and its place within the broader context of solution chemistry. From there, we will move on to **Types and Classification of Solutes**, discussing how solutes can be categorized based on their properties and behaviors. Finally, we will analyze **Behavior and Interactions of Solutes in Solutions**, shedding light on how solutes interact with solvents and other solutes to form complex systems. By understanding these aspects, readers will gain a deeper insight into the critical role solutes play in chemistry and everyday life. Let us begin by defining what a solute is and exploring its foundational concept.
Definition and Concept of a Solute
In the realm of chemistry, understanding the concept of a solute is fundamental to grasping the intricacies of solutions and mixtures. A solute, by definition, is a substance that is dissolved in another substance, known as the solvent, to form a solution. This concept is pivotal in various scientific and everyday contexts. To delve into the essence of solutes, it is crucial to explore their chemical composition and properties, which determine their solubility and interactions within a solution. Additionally, examining the role of solutes in solutions and mixtures reveals their significance in maintaining equilibrium and influencing the overall characteristics of the mixture. Finally, identifying examples of common solutes helps illustrate their practical applications and ubiquity in our environment. By understanding these aspects, we can gain a comprehensive insight into the definition and concept of a solute, which is essential for both theoretical and practical applications in chemistry. This article will explore these key areas to provide a thorough understanding of what constitutes a solute and its importance in chemical contexts.
Chemical Composition and Properties
The chemical composition and properties of a solute are fundamental aspects that define its behavior and interactions within a solution. A solute, by definition, is a substance that is dissolved in a solvent to form a homogeneous mixture. The chemical composition of a solute can vary widely, ranging from simple ions and molecules to complex organic compounds. For instance, sodium chloride (NaCl) is a common solute composed of sodium and chloride ions, while glucose (C6H12O6) is a molecular solute consisting of carbon, hydrogen, and oxygen atoms. The properties of a solute are influenced by its chemical structure and the nature of its interactions with the solvent. One key property is solubility, which is the maximum amount of solute that can dissolve in a given amount of solvent at a particular temperature. Solubility is determined by factors such as the polarity of the solute and solvent molecules, the presence of hydrogen bonding, and the temperature of the solution. For example, ionic solutes like sodium chloride are highly soluble in polar solvents like water due to the strong electrostatic interactions between ions and water molecules. Another important property is the molar mass of the solute, which affects its colligative properties such as boiling-point elevation and freezing-point depression. These properties are critical in understanding how the presence of a solute alters the physical behavior of a solution compared to the pure solvent. Additionally, the chemical reactivity of a solute can influence its stability and potential reactions within the solution. For instance, some solutes may undergo hydrolysis or oxidation reactions when dissolved in water, leading to changes in their chemical composition over time. The physical properties of a solute also play a significant role in its behavior within a solution. These include density, viscosity, and surface tension, which can be altered by the presence of dissolved substances. For example, adding a solute like sucrose to water increases the solution's density and viscosity due to the increased number of particles in the solution. Understanding the chemical composition and properties of a solute is essential for predicting its behavior in various applications, from pharmaceutical formulations to industrial processes. In pharmaceuticals, knowing the solubility and stability of drug molecules is crucial for ensuring their efficacy and safety. In industrial processes, controlling the concentration and properties of solutes can optimize product quality and efficiency. Therefore, a thorough grasp of these aspects is vital for both theoretical understanding and practical applications involving solutes and solutions.
Role in Solutions and Mixtures
In the realm of chemistry, the role of solutes in solutions and mixtures is pivotal, as it underpins the fundamental principles of how substances interact and combine. A solute, by definition, is a substance that is dissolved in another substance known as the solvent, forming a solution. This interaction is crucial because it allows for the creation of homogeneous mixtures where the solute particles are evenly distributed throughout the solvent. The role of a solute can be multifaceted. For instance, in a solution, the solute can alter the physical properties of the solvent, such as its boiling point, freezing point, and viscosity. This phenomenon is exemplified by the boiling-point elevation and freezing-point depression, where the presence of a solute changes these critical temperatures. Additionally, solutes can affect the osmotic pressure of a solution, which is vital in biological systems where cells maintain their structure and function through osmotic balance. In mixtures, solutes play a key role in determining the overall composition and behavior. For example, in a mixture like air, which is primarily composed of nitrogen and oxygen gases, trace amounts of other gases such as carbon dioxide and water vapor act as solutes. These solutes can influence the mixture's properties, such as its density and reactivity. Similarly, in solid solutions like alloys, the solute atoms or molecules distribute within the solvent lattice, enhancing mechanical properties like strength and corrosion resistance. Moreover, solutes are essential in various industrial and biological processes. In pharmaceuticals, solutes are often dissolved in solvents to create medications that can be administered effectively. In agriculture, fertilizers act as solutes in water to provide essential nutrients to plants. In biological systems, solutes such as glucose and amino acids are dissolved in blood plasma and other bodily fluids, facilitating their transport and utilization by cells. The ability of a substance to act as a solute also depends on its chemical compatibility with the solvent. The principle of "like dissolves like" suggests that polar solutes dissolve best in polar solvents, while nonpolar solutes dissolve best in nonpolar solvents. This principle guides the selection of appropriate solvents for various applications, ensuring that the desired properties of the solution or mixture are achieved. In conclusion, the role of solutes in solutions and mixtures is fundamental to understanding how different substances interact and combine. By influencing physical properties, determining mixture compositions, and facilitating various industrial and biological processes, solutes play a critical role in chemistry and its applications. Their behavior and interactions with solvents form the basis of many scientific and practical endeavors, highlighting their importance in both theoretical and applied contexts.
Examples of Common Solutes
In the context of solutions, solutes are substances that dissolve in a solvent to form a homogeneous mixture. Understanding common examples of solutes is crucial for grasping the concept of solutes and their role in various scientific and everyday applications. One of the most familiar solutes is **sugar (sucrose)**, which dissolves readily in water to create a sweet solution. Another common example is **salt (sodium chloride)**, often used to season food and preserve it by inhibiting bacterial growth. These substances are soluble in water due to their polar nature, allowing them to interact with water molecules effectively. **Gases** can also act as solutes, particularly in liquids. For instance, **oxygen** dissolves in water, which is essential for aquatic life. Similarly, **carbon dioxide** dissolves in water to form carbonic acid, a process that occurs naturally in the atmosphere and oceans. These gas solutes play critical roles in environmental chemistry and biological processes. In addition to these simple substances, more complex molecules like **proteins** and **polymers** can also serve as solutes. Proteins, such as those found in blood plasma, dissolve in the aqueous environment of the body and perform vital functions like enzyme activity and transport of nutrients. Polymers, including synthetic materials like polyethylene glycol (PEG), are used in pharmaceuticals and biomedical applications due to their solubility in water and biocompatibility. Furthermore, **minerals** and **vitamins** are essential solutes in biological systems. Minerals such as calcium and potassium ions are crucial for maintaining proper cellular function and nerve transmission. Vitamins, which are organic compounds necessary for various metabolic processes, dissolve in bodily fluids to be transported to where they are needed. In industrial contexts, solutes like **dyes** and **pigments** are dissolved in solvents to create colored solutions used in textiles, paints, and other products. Similarly, **medicinal compounds** are often dissolved in solvents to create pharmaceutical solutions that can be administered orally or intravenously. Understanding these diverse examples of solutes highlights their ubiquity and importance across various fields. From the simplest sugars and salts to complex proteins and polymers, solutes play a vital role in both natural and synthetic systems, underscoring the significance of the concept of a solute in chemistry and beyond.
Types and Classification of Solutes
In the realm of chemistry, solutes play a crucial role in understanding various chemical reactions and processes. A solute is a substance that is dissolved in a solvent to form a solution. However, not all solutes are created equal; they can be classified into different types based on their properties and behaviors. This article delves into the diverse classifications of solutes, exploring key distinctions such as electrolytes versus non-electrolytes, organic versus inorganic solutes, and polar versus non-polar solutes. Each of these classifications provides valuable insights into how solutes interact with their solvents and other substances, influencing the overall characteristics of the solution. By understanding these different types of solutes, we can better grasp the fundamental principles of solution chemistry. This knowledge is essential for a wide range of applications, from pharmaceuticals to environmental science. As we explore these classifications in detail, we will transition seamlessly into a deeper examination of the definition and concept of a solute, providing a comprehensive understanding of this critical chemical component.
Electrolytes vs. Non-Electrolytes
In the realm of solutes, a crucial distinction lies between electrolytes and non-electrolytes, each playing unique roles in various chemical and biological processes. **Electrolytes** are substances that, when dissolved in a solvent, typically water, dissociate into ions. This dissociation enables them to conduct electricity, a property that is vital in numerous physiological and industrial contexts. For instance, sodium chloride (NaCl), commonly known as table salt, is an electrolyte because it breaks down into sodium (Na+) and chloride (Cl-) ions in aqueous solution. Other examples include potassium nitrate (KNO3) and calcium chloride (CaCl2). The ability of electrolytes to conduct electricity is fundamental in biological systems; for example, nerve impulses rely on the movement of ions across cell membranes, and electrolyte imbalances can lead to serious health issues such as muscle weakness or cardiac arrhythmias. On the other hand, **non-electrolytes** are solutes that do not dissociate into ions when dissolved in a solvent. These substances remain as molecules and do not contribute to the electrical conductivity of the solution. Examples of non-electrolytes include sugar (sucrose), urea, and ethanol. Unlike electrolytes, non-electrolytes do not participate in ionic reactions and are generally less reactive in aqueous solutions. However, they still play critical roles; for instance, sugars are essential energy sources for cells, while urea is a key component in the body's waste removal process. The classification of solutes into electrolytes and non-electrolytes is not merely academic; it has practical implications across various fields. In medicine, understanding whether a substance is an electrolyte or non-electrolyte is crucial for diagnosing and treating conditions related to fluid balance and ion homeostasis. In chemistry, this distinction helps predict the behavior of solutions in different reactions and processes. For example, electrolytes are often used in electrochemical cells to facilitate the flow of electric current, whereas non-electrolytes are commonly used as solvents or reactants in organic synthesis. In summary, the differentiation between electrolytes and non-electrolytes underscores the diverse ways in which solutes interact with their solvents and the broader environment. This distinction is pivotal for understanding both the fundamental principles of chemistry and the intricate mechanisms of biological systems, highlighting the importance of solute classification in various scientific and practical applications.
Organic vs. Inorganic Solutes
When discussing solutes, it is crucial to differentiate between organic and inorganic solutes, as each category has distinct characteristics and implications in various fields such as chemistry, biology, and environmental science. **Organic solutes** are derived from living organisms or contain carbon-hydrogen bonds, which are fundamental to their structure. These solutes can range from simple sugars and amino acids to complex molecules like proteins and nucleic acids. Organic solutes play vital roles in biological systems; for instance, glucose serves as a primary energy source for cells, while amino acids are the building blocks of proteins. In environmental contexts, organic solutes can influence water quality, with high levels of organic matter potentially leading to eutrophication in aquatic ecosystems. The presence of organic solutes in soil also affects its fertility and microbial activity. On the other hand, **inorganic solutes** are typically derived from mineral sources and lack carbon-hydrogen bonds. Common examples include salts like sodium chloride (NaCl), calcium carbonate (CaCO3), and various ions such as potassium (K+), magnesium (Mg2+), and phosphate (PO43-). Inorganic solutes are essential for numerous biological processes; for example, ions like sodium and potassium are critical for maintaining cellular osmotic balance, while calcium is vital for bone health. In industrial applications, inorganic solutes are used in manufacturing processes, such as the production of detergents and fertilizers. However, excessive levels of certain inorganic solutes can be harmful; for instance, high concentrations of nitrates in water can pose health risks to humans and wildlife. The classification of solutes into organic and inorganic categories is not merely academic; it has practical implications across various disciplines. Understanding the nature of solutes is essential for developing effective strategies in fields like agriculture, where the balance of organic and inorganic nutrients in soil directly impacts crop yield and quality. In medical research, distinguishing between organic and inorganic solutes helps in diagnosing and treating conditions related to electrolyte imbalances or metabolic disorders. Furthermore, in environmental monitoring, identifying the types of solutes present in water bodies or soil helps in assessing pollution levels and implementing appropriate remediation measures. In summary, the distinction between organic and inorganic solutes is fundamental to understanding their roles, behaviors, and impacts in biological systems, industrial processes, and environmental contexts. Recognizing these differences enables scientists, policymakers, and practitioners to make informed decisions that optimize outcomes in diverse fields. Whether it is ensuring the health of ecosystems, improving agricultural productivity, or advancing medical treatments, the classification of solutes into organic and inorganic categories provides a critical framework for addressing complex challenges effectively.
Polar vs. Non-Polar Solutes
When discussing the types and classification of solutes, a crucial distinction lies between polar and non-polar solutes. This differentiation is pivotal because it influences the solute's behavior in various solvents, affecting its solubility, reactivity, and overall interaction with the solvent molecules. **Polar Solutes:** Polar solutes are characterized by their asymmetric distribution of electrons, resulting in a partial positive charge on one end and a partial negative charge on the other. This polarity arises from the difference in electronegativity between the atoms forming the molecule. For instance, water (H₂O) is a polar molecule due to the higher electronegativity of oxygen compared to hydrogen. Polar solutes tend to dissolve well in polar solvents because they can form hydrogen bonds or dipole-dipole interactions with the solvent molecules. These interactions enhance the solute's solubility and stability within the solution. Examples of polar solutes include sugars, amino acids, and many organic compounds containing functional groups like hydroxyl (-OH), carboxyl (-COOH), or amine (-NH₂). **Non-Polar Solutes:** In contrast, non-polar solutes have a symmetrical distribution of electrons, leading to no net dipole moment. These molecules do not exhibit significant partial charges and thus do not form strong interactions with polar solvent molecules. Non-polar solutes are typically hydrophobic and dissolve better in non-polar solvents where London dispersion forces (van der Waals forces) are the primary mode of interaction. Examples of non-polar solutes include hydrocarbons such as methane (CH₄), ethane (C₂H₆), and benzene (C₆H₆). The lack of strong intermolecular forces between non-polar solutes and polar solvents often results in poor solubility in water or other polar solvents. **Implications:** Understanding the polarity of a solute is essential for predicting its behavior in different solvents. For example, in pharmaceutical applications, the polarity of a drug molecule can influence its absorption, distribution, metabolism, and excretion (ADME) properties. Similarly, in chemical synthesis, choosing the appropriate solvent based on the polarity of the reactants and products can significantly impact reaction rates and yields. In summary, the distinction between polar and non-polar solutes is fundamental to understanding their solubility characteristics and interactions with various solvents. This knowledge is crucial for a wide range of scientific and industrial applications, from drug development to chemical engineering. By recognizing whether a solute is polar or non-polar, scientists can better predict its behavior and optimize processes involving solute-solvent interactions.
Behavior and Interactions of Solutes in Solutions
In the intricate world of chemistry, the behavior and interactions of solutes in solutions are fundamental concepts that underpin a wide range of phenomena. Understanding these dynamics is crucial for grasping various chemical processes and their applications. This article delves into three key aspects that define the behavior of solutes: solubility and solubility limits, colligative properties and their effects, and chemical reactions involving solutes. Solubility and solubility limits explore how much of a substance can dissolve in a solvent, influencing the formation of solutions. Colligative properties, such as boiling point elevation and freezing point depression, reveal how the presence of solutes affects the physical properties of solutions. Additionally, chemical reactions involving solutes highlight the role of dissolved substances in driving chemical transformations. By examining these facets, we gain a comprehensive understanding of how solutes interact within solutions. This knowledge is rooted in the definition and concept of a solute, which forms the foundation for understanding these complex interactions.
Solubility and Solubility Limits
Solubility and solubility limits are fundamental concepts in understanding the behavior and interactions of solutes in solutions. Solubility refers to the maximum amount of a substance (solute) that can dissolve in a given amount of solvent at a particular temperature and pressure. This property is crucial because it determines how much of a solute can be dissolved before it starts to precipitate out of the solution. The solubility limit, often expressed in terms of concentration (e.g., grams per 100 milliliters of solvent), varies significantly among different substances and is influenced by several factors including temperature, pressure, and the nature of both the solute and solvent. For instance, most salts and sugars exhibit increased solubility with rising temperatures, meaning that more of these substances can dissolve in a solvent as the temperature increases. Conversely, gases generally become less soluble in liquids as temperature rises. Pressure also plays a role, particularly for gases; an increase in pressure can significantly enhance the solubility of gases in liquids according to Henry's Law. The chemical properties of both the solute and solvent are equally important; polar solvents like water tend to dissolve polar solutes well due to their ability to form hydrogen bonds or other dipole-dipole interactions. Understanding solubility limits is essential in various fields such as chemistry, pharmaceuticals, and environmental science. In chemistry labs, knowing the solubility limits helps in preparing solutions accurately without causing precipitation or supersaturation. In pharmaceuticals, it is critical for formulating drugs that must be soluble enough to be effective yet stable over time. In environmental science, solubility affects how pollutants interact with water bodies and soil. Moreover, solubility can be affected by the presence of other solutes in what is known as the "common ion effect" or "salting out." For example, adding a common ion to a solution can decrease the solubility of another solute that shares that ion. This principle is often used in industrial processes to separate mixtures of salts or other compounds. In summary, solubility and solubility limits are key parameters that dictate how solutes interact within solutions. These properties are influenced by multiple factors including temperature, pressure, and chemical compatibility between solutes and solvents. Understanding these concepts is vital for various scientific applications and industrial processes where precise control over solution composition is necessary. By grasping these principles, scientists can better predict and manipulate the behavior of solutes in solutions to achieve desired outcomes across different fields of study.
Colligative Properties and Their Effects
Colligative properties are a set of physical properties of solutions that depend on the concentration of solute particles, rather than their identity. These properties include boiling point elevation, freezing point depression, osmotic pressure, and vapor pressure lowering. Understanding these colligative properties is crucial for grasping the behavior and interactions of solutes in solutions. When a solute is dissolved in a solvent, it alters the boiling and freezing points of the solution. The boiling point elevation occurs because the solute particles interfere with the formation of hydrogen bonds between solvent molecules, making it more difficult for the solvent to boil. Conversely, the freezing point depression happens as solute particles disrupt the crystalline structure of the solvent, requiring lower temperatures for solidification to occur. These changes are directly proportional to the molality of the solution, which is the number of moles of solute per kilogram of solvent. Osmotic pressure is another significant colligative property, particularly relevant in biological systems. It is the pressure required to prevent the flow of solvent molecules through a semipermeable membrane into a solution with higher solute concentration. This phenomenon is critical in maintaining cellular balance and function, as cells rely on osmotic pressure to regulate fluid and ion exchange. Vapor pressure lowering is observed when a non-volatile solute is added to a solvent. The presence of solute particles reduces the rate at which solvent molecules escape into the vapor phase, resulting in a lower vapor pressure compared to the pure solvent. This effect is essential in various industrial processes, such as the production of antifreeze solutions and the preservation of food items. The effects of colligative properties are far-reaching and influence various aspects of solution behavior. For instance, in pharmaceutical applications, understanding these properties helps in formulating drugs that can be effectively administered and absorbed by the body. In environmental science, colligative properties play a role in understanding how pollutants affect aquatic ecosystems. Additionally, in food science, these properties are crucial for preserving food quality and safety by controlling factors like freezing temperatures and moisture levels. In summary, colligative properties provide valuable insights into how solutes interact with solvents at a molecular level, affecting key physical characteristics of solutions. By understanding these properties, scientists and engineers can design and optimize solutions for a wide range of applications, from medical treatments to industrial processes, highlighting the importance of solute behavior in solution chemistry.
Chemical Reactions Involving Solutes
Chemical reactions involving solutes are fundamental processes that underpin various phenomena in solutions. When a solute dissolves in a solvent, it disperses into individual atoms, molecules, or ions, which then interact with the solvent and other solutes present. These interactions can lead to a wide range of chemical reactions, including precipitation, acid-base reactions, oxidation-reduction (redox) reactions, and complexation. **Precipitation Reactions** occur when two solutions containing ions are mixed, resulting in the formation of an insoluble solid. For example, when a solution of silver nitrate (AgNO₃) is mixed with a solution of sodium chloride (NaCl), silver chloride (AgCl) precipitates out because it is insoluble in water. This type of reaction is crucial in analytical chemistry for identifying and separating ions. **Acid-Base Reactions** involve the transfer of protons (H⁺ ions) between solutes. In aqueous solutions, acids donate H⁺ ions while bases accept them. For instance, hydrochloric acid (HCl) reacts with sodium hydroxide (NaOH) to form water and sodium chloride. These reactions are vital in understanding pH regulation in biological systems and industrial processes. **Oxidation-Reduction (Redox) Reactions** involve the transfer of electrons between solutes. In these reactions, one species loses electrons (oxidized) while another gains electrons (reduced). A classic example is the reaction between potassium permanganate (KMnO₄) and iron(II) sulfate (FeSO₄), where permanganate ions oxidize iron(II) ions to iron(III) ions. Redox reactions are essential in energy storage devices like batteries and in biological processes such as cellular respiration. **Complexation Reactions** involve the formation of coordination compounds where a central metal ion or atom is surrounded by ligands. For example, when ammonia (NH₃) is added to a solution of copper(II) sulfate (CuSO₄), it forms a blue-colored complex [Cu(NH₃)₄]²⁺. These reactions are significant in analytical chemistry for detecting metal ions and in biological systems where metal ions play critical roles. Understanding these chemical reactions involving solutes is crucial for various applications across different fields. In chemistry labs, these reactions help in identifying and quantifying substances. In industrial processes, they are used to synthesize new materials and control reaction conditions. In biological systems, these reactions underpin metabolic pathways and cellular functions. Therefore, studying the behavior and interactions of solutes in solutions provides a comprehensive insight into the dynamic world of chemical reactions that shape our understanding of chemistry and its applications.