What Is The Smallest Thing In The World
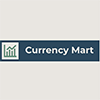
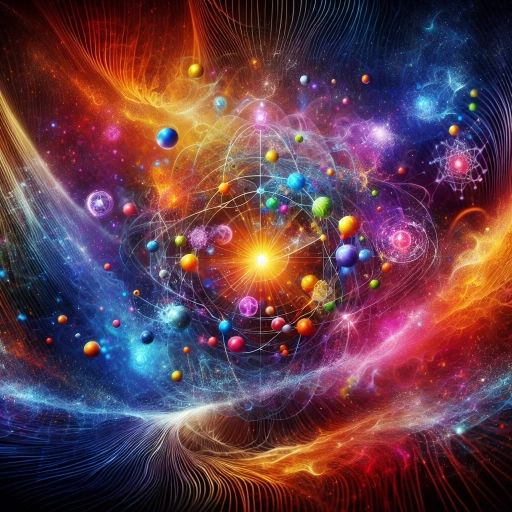
In the vast expanse of our universe, the concept of "smallest" is a fascinating and complex topic that has intrigued scientists and philosophers for centuries. As we delve into the microscopic and quantum realms, we encounter entities that challenge our conventional understanding of size and scale. This article aims to explore what constitutes the smallest thing in the world, navigating through three key areas: understanding the concept of "smallest," exploring the quantum realm, and examining real-world applications and implications. We begin by dissecting the notion of "smallest," a term that is both relative and absolute, depending on the context. This foundational understanding sets the stage for our journey into the quantum realm, where particles like electrons and quarks redefine our perception of minuteness. Finally, we will discuss how these tiny entities have significant real-world applications and implications, influencing fields from technology to medicine. Let us start by **Understanding the Concept of "Smallest"** to lay the groundwork for this intriguing exploration.
Understanding the Concept of "Smallest"
Understanding the concept of "smallest" is a multifaceted exploration that delves into various dimensions of size and scale, historical perspectives, and modern scientific definitions. At its core, the notion of "smallest" challenges our conventional understanding of measurement and perception. To grasp this concept fully, it is essential to define size and scale, which serves as the foundational framework for comprehending what constitutes "small." This involves examining the units and methods used to measure objects and phenomena, from the microscopic to the macroscopic. Additionally, historical perspectives on smallness offer insights into how different cultures and eras have perceived and utilized the concept of smallness, often reflecting broader societal values and technological capabilities. Finally, modern scientific definitions, particularly in fields like physics and biology, provide precise and quantifiable understandings of what constitutes the smallest entities in our universe. By starting with a clear definition of size and scale, we can better navigate these diverse perspectives and gain a deeper appreciation for the complexity and significance of the concept of "smallest." Therefore, let us begin by defining size and scale, the bedrock upon which our understanding of smallness is built.
Defining Size and Scale
Defining size and scale is crucial when delving into the concept of the smallest things in the world. Size refers to the physical dimensions or magnitude of an object, while scale pertains to the relative size or proportion of objects in comparison to each other. Understanding these concepts is essential for grasping the vast range of sizes that exist, from the infinitesimally small to the astronomically large. At the smallest end of the spectrum, we encounter particles that are almost incomprehensibly tiny. For instance, atoms, which are the building blocks of matter, measure about 1 to 3 angstroms (Å) in diameter, with 1 Å being equal to 0.1 nanometers (nm). Within these atoms, protons and neutrons reside in the nucleus, while electrons orbit around it. The size of these subatomic particles is even more minuscule; protons and neutrons have diameters on the order of femtometers (fm), where 1 fm equals 10^-15 meters. To put these sizes into perspective, consider that if an atom were the size of a basketball, a proton would be about the size of a pea. This analogy highlights the enormous scale difference between atomic and subatomic levels. Moving further down the scale, we enter the realm of quantum mechanics, where particles like quarks and leptons exist. These fundamental particles are among the smallest known entities in the universe and are measured in terms of their energy rather than physical size due to their incredibly small dimensions. The concept of scale becomes particularly relevant when comparing these microscopic sizes to everyday objects. For example, if you were to line up atoms side by side, it would take approximately 100 million atoms to span just one centimeter. This underscores how tiny individual atoms are and how they collectively form larger structures that we can see and interact with. Moreover, understanding size and scale helps us appreciate technological advancements that allow us to manipulate and study these tiny entities. Tools like scanning tunneling microscopes can visualize individual atoms on surfaces, while particle accelerators can accelerate subatomic particles to nearly the speed of light to study their properties. In conclusion, defining size and scale provides a framework for comprehending the vast hierarchy of sizes in our universe. From atoms to subatomic particles, each level offers insights into the fundamental nature of matter and energy. This understanding not only deepens our knowledge but also inspires further exploration into what constitutes "the smallest thing" in various scientific contexts. By recognizing these dimensions and proportions, we gain a richer appreciation for both the minuscule and the massive scales that define our world.
Historical Perspectives on Smallness
Historical perspectives on smallness reveal a fascinating evolution in human understanding and perception of the concept. From ancient philosophies to modern scientific discoveries, the notion of "small" has been redefined and refined over time. In ancient Greece, philosophers like Democritus proposed the existence of atoms, which were considered the smallest indivisible units of matter. This early atomic theory laid the groundwork for later scientific inquiries into the nature of smallness. During the Renaissance, the invention of the microscope by Antonie van Leeuwenhoek in the 17th century opened up a new world of microscopic life, revealing organisms and structures that were previously invisible to the naked eye. This discovery not only expanded our understanding of biological diversity but also challenged traditional views on what constitutes "small." The microscopic realm introduced a new dimension to the concept, highlighting that even within seemingly empty spaces, there existed complex ecosystems and structures. The 19th and 20th centuries saw significant advancements in physics, particularly with the development of quantum mechanics. Scientists like Max Planck and Niels Bohr delved into the atomic and subatomic levels, uncovering particles such as electrons, protons, and neutrons. These discoveries further miniaturized our understanding of smallness, revealing that even atoms themselves are composed of smaller entities. The concept of wave-particle duality introduced by Louis de Broglie added another layer of complexity, showing that particles could exhibit both wave-like and particle-like behavior depending on how they were observed. In recent decades, nanotechnology has emerged as a field that manipulates matter on an atomic or molecular scale. This has led to innovations in materials science, electronics, and medicine, among other areas. The ability to engineer materials at the nanoscale has pushed our understanding of smallness into new territories, enabling the creation of ultra-fine structures with unique properties. Throughout history, each new discovery has not only refined our definition of "small" but also expanded our capabilities to interact with and manipulate tiny entities. From philosophical speculations about indivisible atoms to the precise engineering of nanoparticles, human curiosity about smallness has driven scientific progress and transformed our understanding of the world around us. This journey underscores that the concept of "smallest" is not static but rather a dynamic frontier that continues to evolve as our tools and knowledge advance. As we delve deeper into the microscopic and subatomic realms, we are constantly reminded that there may always be something even smaller waiting to be discovered.
Modern Scientific Definitions
In the realm of modern scientific definitions, the concept of "smallest" is intricately tied to advancements in various fields such as physics, chemistry, and biology. At the heart of this inquiry lies the quest to understand the fundamental building blocks of our universe. In physics, the smallest entities are often considered to be elementary particles like quarks and leptons, which are the constituents of atoms and cannot be further divided into simpler forms. These particles are governed by the principles of quantum mechanics, a branch of physics that explains the behavior of matter and energy at an atomic and subatomic level. Here, concepts like wave-particle duality and the Heisenberg Uncertainty Principle come into play, highlighting the complex nature of these tiny entities. Chemistry also delves into the realm of the smallest through the study of atoms and molecules. The atom, once thought to be indivisible, is now known to consist of protons, neutrons, and electrons. Protons and neutrons reside in the nucleus, while electrons orbit around it in defined energy levels or shells. The interactions between these atoms form molecules, which are the basic units of chemical compounds. Understanding these interactions is crucial for grasping chemical reactions and the properties of materials. Biology, on the other hand, explores the smallest units of life—cells. Cells are the fundamental units of living organisms and contain genetic material in the form of DNA, which encodes all the information necessary for life processes. Within cells, there are organelles such as mitochondria and ribosomes that perform specific functions essential for cellular survival and reproduction. The intersection of these disciplines often leads to fascinating discoveries about what constitutes "smallest." For instance, nanotechnology—a field that bridges physics, chemistry, and biology—deals with materials and devices on a nanoscale (typically between 1-100 nanometers). Here, scientists manipulate matter at an atomic or molecular level to create new materials with unique properties, further blurring the lines between what is considered "small" in different scientific contexts. Moreover, recent advancements in microscopy have allowed scientists to visualize and study structures at unprecedented scales. Techniques like scanning tunneling microscopy (STM) and atomic force microscopy (AFM) enable researchers to observe individual atoms on surfaces, providing insights into surface chemistry and material science. In conclusion, modern scientific definitions of "smallest" are multifaceted and span across various disciplines. From the elementary particles in physics to the cells in biology and the nanoscale materials in nanotechnology, each field contributes a unique perspective on what constitutes the smallest entity. These definitions not only reflect our current understanding but also drive further research into the intricate details of our universe, continually refining our comprehension of the smallest things in the world.
Exploring the Quantum Realm
Exploring the Quantum Realm is a journey into the heart of matter and energy, where the rules of classical physics no longer apply. This realm, governed by the principles of quantum mechanics, reveals a world of subatomic particles, quantum fields, and forces that shape our understanding of reality. At its core, quantum mechanics introduces us to a new paradigm where particles can exist in multiple states simultaneously and where probability rather than determinism reigns. Delving deeper, we encounter subatomic particles such as quarks and leptons, which are the building blocks of all matter and play a crucial role in understanding the structure of atoms and beyond. Furthermore, quantum fields and forces provide the framework for how these particles interact, influencing everything from the behavior of electrons in atoms to the dynamics of cosmic phenomena. As we embark on this exploration, our first step will be to delve into the foundational principles of quantum mechanics, setting the stage for a comprehensive understanding of this fascinating realm. Therefore, let us begin with an **Introduction to Quantum Mechanics**, where we will uncover the fundamental concepts that underpin our journey into the quantum world.
Introduction to Quantum Mechanics
**Introduction to Quantum Mechanics** Quantum mechanics, a fundamental branch of physics, delves into the behavior of matter and energy at the smallest scales, revealing a realm vastly different from the classical world we experience. This discipline emerged in the early 20th century as scientists sought to explain phenomena that classical physics could not. Key figures such as Max Planck, Albert Einstein, Niels Bohr, Louis de Broglie, Erwin Schrödinger, and Werner Heisenberg laid the groundwork for this new understanding. At its core, quantum mechanics posits that particles like electrons and photons exhibit both wave-like and particle-like properties, a concept known as wave-particle duality. The Heisenberg Uncertainty Principle further complicates our ability to measure these particles precisely, suggesting that certain properties, such as position and momentum, cannot be known simultaneously with infinite precision. Quantum mechanics also introduces the concept of superposition, where a quantum system can exist in multiple states simultaneously until observed, and entanglement, where the state of one particle is instantaneously affected by the state of another, regardless of distance. These principles are encapsulated in the Schrödinger equation, which predicts the time-evolution of a quantum system. Quantum mechanics has been instrumental in explaining phenomena such as the photoelectric effect, the Compton effect, and the structure of atoms and molecules. Its applications are diverse and profound, underpinning technologies like transistors, lasers, and magnetic resonance imaging (MRI). As we continue to explore the quantum realm, we uncover new insights into the nature of reality itself, pushing the boundaries of what we thought was possible and opening doors to future innovations that could revolutionize various fields from computing to medicine. This journey into the quantum world not only deepens our understanding of the smallest things in the universe but also challenges our intuitive perceptions of space, time, and causality.
Subatomic Particles: Quarks and Leptons
In the quantum realm, the smallest building blocks of matter are subatomic particles, specifically quarks and leptons. These fundamental particles are the cornerstone of the Standard Model of particle physics, which describes the behavior and interactions of all known subatomic particles. Quarks, named by physicist Murray Gell-Mann after a line in James Joyce's novel "Finnegans Wake," come in six flavors: up, down, charm, strange, top, and bottom. Each flavor has three different colors—red, green, and blue—leading to a total of 18 different quarks. Quarks are never found alone in nature but are always bound together with other quarks or antiquarks to form composite particles called hadrons. The most familiar hadrons are protons and neutrons, which make up atomic nuclei; protons consist of two up quarks and one down quark, while neutrons consist of two down quarks and one up quark. Leptons, on the other hand, are a class of particles that do not participate in the strong nuclear force and thus do not form hadrons. There are six types of leptons: electron, muon, tau, and their corresponding neutrinos (electron neutrino, muon neutrino, and tau neutrino). Unlike quarks, leptons can exist as free particles and are not confined within hadrons. The electron is the most well-known lepton and is a key component of atoms, orbiting the nucleus in energy levels or shells. Neutrinos are intriguing because they have very small masses and interact via the weak nuclear force, making them extremely difficult to detect directly. The interactions between quarks and leptons are mediated by fundamental forces described by the Standard Model. Quarks interact via the strong nuclear force, which is mediated by particles called gluons. Leptons interact primarily through the weak nuclear force, which is mediated by W and Z bosons, and through electromagnetism, mediated by photons. Understanding these interactions has been crucial for advancing our knowledge of particle physics and has led to numerous groundbreaking discoveries, including the Higgs boson—a particle responsible for giving other particles mass. Exploring these subatomic particles has opened up new avenues for research in fields such as cosmology, materials science, and medical imaging. For instance, understanding quark behavior helps scientists study the early universe's conditions during the Big Bang when quarks were first formed. In materials science, research on quark-gluon plasma—a state of matter where quarks are deconfined—provides insights into extreme conditions found in stars and other celestial bodies. In medicine, techniques like positron emission tomography (PET) scans rely on the annihilation of electrons and positrons (the antiparticle of electrons), which are leptons. In conclusion, quarks and leptons represent the smallest known units of matter that make up everything around us. Their unique properties and interactions govern the behavior of all physical phenomena at the most fundamental level. As scientists continue to explore these particles through experiments at high-energy colliders like the Large Hadron Collider (LHC), they uncover more about the intricate workings of the quantum realm and push the boundaries of human understanding ever further. This ongoing quest for knowledge not only deepens our comprehension of the universe but also drives technological innovation and scientific progress across various disciplines.
Quantum Fields and Forces
In the vast and intricate landscape of the quantum realm, quantum fields and forces play a pivotal role in understanding the fundamental nature of reality. These fields are not just abstract concepts but tangible, albeit invisible, entities that permeate space and time. At their core, quantum fields represent the quantized states of particles and their interactions, encapsulating the essence of quantum mechanics. For instance, the electromagnetic field is a quantum field that mediates the force between charged particles, while the Higgs field is responsible for imparting mass to fundamental particles. The concept of quantum fields is deeply intertwined with the theory of quantum field theory (QFT), which provides a framework for describing how particles interact at the smallest scales. QFT posits that particles such as electrons and photons are excitations of underlying fields, much like how waves are excitations of the ocean's surface. This perspective revolutionizes our understanding of particle interactions, transforming them from point-like collisions to continuous field dynamics. One of the most compelling aspects of quantum fields is their role in mediating fundamental forces. The Standard Model of particle physics, a cornerstone of modern physics, describes three of the four fundamental forces—electromagnetism, the weak nuclear force, and the strong nuclear force—through the lens of quantum fields. For example, photons are the quanta of the electromagnetic field, gluons mediate the strong nuclear force, and W and Z bosons facilitate the weak nuclear force. These force-carrying particles, or gauge bosons, arise from the symmetries of their respective fields and are crucial for explaining phenomena ranging from atomic stability to nuclear reactions. Moreover, quantum fields exhibit unique properties that distinguish them from classical fields. Quantum fluctuations, for instance, introduce inherent uncertainties and temporary particle-antiparticle pairs that pop in and out of existence even in a vacuum. These fluctuations have profound implications for our understanding of space-time itself and have been experimentally verified through phenomena such as the Casimir effect. The study of quantum fields also extends into cosmology and the early universe. The Higgs field, discovered in 2012 at CERN, is believed to have played a crucial role in the symmetry-breaking processes that occurred during the universe's first fraction of a second. This field's presence explains why some particles acquired mass while others remained massless, a differentiation that underpins much of modern particle physics. In conclusion, quantum fields and forces are not merely theoretical constructs but are integral components of our current understanding of the universe. They bridge the gap between the microscopic world of particles and the macroscopic world we experience, offering insights into the deepest mysteries of existence. As we continue to explore the quantum realm, delving deeper into these fields and forces will undoubtedly reveal more secrets about the smallest things in the world and the very fabric of reality itself.
Real-World Applications and Implications
In the realm of modern science, the understanding and manipulation of matter at the nanoscale have opened up a plethora of real-world applications and implications. This field, known as nanotechnology, has the potential to revolutionize various aspects of our lives. The article delves into three critical areas that highlight the significance of nanotechnology: its diverse applications, the intricate workings of biological systems at the microscale, and the broader societal impact of understanding smallness. By exploring **Nanotechnology and Its Applications**, we uncover how advancements in this field are transforming industries such as medicine, energy, and materials science. Additionally, **Biological Systems at the Microscale** reveals the intricate mechanisms that govern life at the cellular and molecular levels, providing insights into disease prevention and treatment. Finally, **Societal Impact of Understanding Smallness** examines how this newfound knowledge influences our daily lives, from environmental sustainability to economic development. As we transition into the specifics of **Nanotechnology and Its Applications**, it becomes clear that the future of innovation is deeply rooted in the manipulation and understanding of matter at its smallest scale.
Nanotechnology and Its Applications
Nanotechnology, the manipulation of matter on an atomic, molecular, and supramolecular scale, has revolutionized various fields with its vast array of applications. At the heart of nanotechnology lies the ability to engineer materials and devices at the nanoscale, typically defined as 1 to 100 nanometers. This precision allows for the creation of materials with unique properties that are not observed at larger scales. In real-world applications, nanotechnology has made significant impacts in medicine, electronics, energy, and environmental science. In medicine, nanotechnology has enabled the development of targeted drug delivery systems. Nanoparticles can be engineered to carry drugs directly to diseased cells, reducing side effects and improving treatment efficacy. For instance, nanoparticles coated with specific antibodies can target cancer cells, delivering chemotherapy directly to the tumor site while sparing healthy tissue. Additionally, nanotechnology has led to advancements in diagnostic tools, such as biosensors that can detect biomarkers for diseases at an early stage, facilitating timely interventions. In the realm of electronics, nanotechnology has driven the miniaturization of devices. Transistors, the building blocks of modern electronics, have been scaled down to nanoscale dimensions, enabling the production of smaller, faster, and more efficient electronic devices. This has been crucial for the development of smartphones, laptops, and other consumer electronics that we use daily. Furthermore, nanomaterials like graphene and carbon nanotubes are being explored for their exceptional electrical conductivity and mechanical strength, promising even more powerful and durable electronic components in the future. The energy sector also benefits significantly from nanotechnology. Solar cells and fuel cells have seen improvements in efficiency due to the use of nanostructured materials. For example, nanostructured solar cells can enhance light absorption and electron transport, leading to higher energy conversion rates. Similarly, nanocatalysts in fuel cells can increase the reaction rate and efficiency of chemical reactions, making these devices more viable for widespread adoption. Environmental applications of nanotechnology include water purification and pollution remediation. Nanofiltration membranes can remove contaminants from water at the molecular level, providing clean drinking water in areas where it is scarce. Additionally, nanoparticles can be designed to break down pollutants in soil and groundwater, offering a promising solution for environmental cleanup efforts. The implications of these advancements are profound. Nanotechnology has the potential to address some of the world's most pressing challenges, such as healthcare disparities, energy shortages, and environmental degradation. However, it also raises ethical and safety concerns. The handling and disposal of nanoparticles require careful consideration to avoid unintended environmental impacts or health risks. Regulatory frameworks and public awareness campaigns are essential to ensure that the benefits of nanotechnology are realized while minimizing its risks. In conclusion, nanotechnology's influence extends across multiple disciplines, transforming how we approach problems in medicine, electronics, energy, and environmental science. As research continues to push the boundaries of what is possible at the nanoscale, we can expect even more innovative solutions to emerge, further integrating nanotechnology into our daily lives and shaping a more sustainable future.
Biological Systems at the Microscale
At the microscale, biological systems reveal intricate mechanisms that underpin life itself. These systems, often invisible to the naked eye, are composed of cells, the fundamental units of life, and their interactions with each other and their environment. Within cells, organelles such as mitochondria, ribosomes, and lysosomes perform specialized functions essential for cellular survival and function. For instance, mitochondria generate energy through cellular respiration, while ribosomes synthesize proteins from genetic blueprints encoded in DNA. This microscopic world is also home to complex networks of proteins, lipids, and carbohydrates that form the cell membrane, regulating what enters and leaves the cell. The study of these biological systems at the microscale has profound real-world applications and implications. In medicine, understanding cellular processes helps in diagnosing and treating diseases. For example, cancer research focuses on how cancer cells differ from normal cells in terms of growth, division, and metabolism. Targeted therapies can then be developed to exploit these differences, leading to more effective treatments with fewer side effects. Additionally, insights into microbial biology have revolutionized fields like public health and agriculture. Antibiotics and vaccines, which rely on our understanding of bacterial and viral biology at the microscale, have significantly reduced mortality rates from infectious diseases. In agriculture, knowledge of plant cell biology has led to the development of genetically modified crops that are more resilient to environmental stresses and pests. This not only increases food production but also reduces the need for pesticides and other chemicals that can harm ecosystems. Furthermore, advances in biotechnology have enabled the creation of biofuels from microbial fermentation processes, offering a sustainable alternative to fossil fuels. The implications extend beyond these practical applications; they also influence our broader understanding of life and its origins. The study of extremophiles—organisms that thrive in extreme environments—provides clues about how life might have emerged on Earth and whether it could exist elsewhere in the universe. Moreover, research into synthetic biology aims to engineer new biological systems or modify existing ones to perform novel functions, potentially solving some of humanity's most pressing challenges such as climate change and resource depletion. In conclusion, the microscopic realm of biological systems is a vibrant tapestry of intricate mechanisms and interactions that underpin all living organisms. By delving into this world, scientists uncover not only the fundamental principles of life but also innovative solutions to real-world problems. As our understanding deepens, so too do the possibilities for improving human health, enhancing food security, and addressing global environmental issues—all testament to the profound impact of exploring life at its smallest scales.
Societal Impact of Understanding Smallness
Understanding the concept of smallness has profound societal impacts that extend far beyond the realm of scientific curiosity. At its core, grasping the smallest units of matter and energy opens doors to innovative technologies, improves our daily lives, and reshapes various industries. For instance, advancements in nanotechnology, which deals with materials on a nanoscale (typically 1-100 nanometers), have led to breakthroughs in healthcare. Nanoparticles are being used to deliver targeted cancer treatments, reducing side effects and increasing the efficacy of chemotherapy. This precision medicine not only enhances patient outcomes but also reduces healthcare costs by minimizing the need for prolonged hospital stays and follow-up treatments. In the field of electronics, understanding smallness has enabled the development of smaller, more efficient devices. The miniaturization of transistors and other components has led to the creation of smartphones, laptops, and other portable electronics that are integral to modern life. These devices have revolutionized communication, education, and commerce, bridging geographical gaps and fostering global connectivity. Moreover, the energy sector benefits from nanomaterials that enhance the efficiency of solar cells and batteries, contributing to sustainable energy solutions and mitigating climate change. The impact of smallness is also evident in environmental science. Nanofiltration technologies are being employed to purify water, making clean drinking water accessible to communities worldwide. This not only improves public health but also addresses one of the most pressing global challenges—water scarcity. Additionally, nanomaterials are used in pollution control, helping to clean up contaminated soil and water by breaking down toxic substances into harmless components. In agriculture, understanding smallness has led to the development of precision farming techniques. Nanoparticles can be used as fertilizers and pesticides, ensuring that these chemicals are delivered directly to the plants, reducing waste and environmental impact. This targeted approach enhances crop yields while minimizing the use of harmful chemicals, contributing to sustainable agricultural practices. Furthermore, the societal impact of understanding smallness extends into the realm of policy and governance. As technologies derived from nanoscience become more prevalent, there is a growing need for regulatory frameworks that ensure their safe and ethical use. Governments and international organizations are developing guidelines to manage the risks associated with nanomaterials, protecting both human health and the environment. In conclusion, the societal impact of understanding smallness is multifaceted and far-reaching. From healthcare and electronics to environmental science and agriculture, the implications are profound. As we continue to explore and harness the power of the smallest things in the world, we are not only advancing scientific knowledge but also creating a better future for humanity. This understanding drives innovation, improves quality of life, and addresses some of the most critical challenges facing our world today.