What Is An Mga
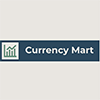
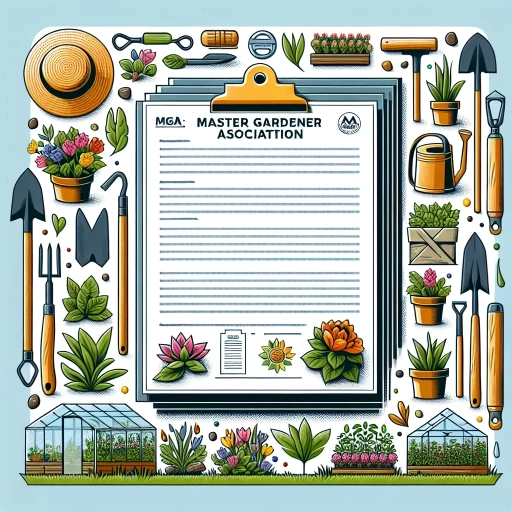
In the realm of advanced technology, the term "MGA" stands out as a significant concept, particularly in fields such as engineering, electronics, and telecommunications. An MGA, or Monolithic Microwave Integrated Circuit (MMIC) Amplifier, is a sophisticated device that plays a crucial role in modern communication systems. To fully understand the importance and functionality of an MGA, it is essential to delve into its definition and origins, explore its technical aspects and functionality, and examine its various applications and real-world use cases. This article will provide a comprehensive overview of MGAs, starting with their definition and origins, which will set the stage for a deeper exploration of their technical intricacies and practical applications. By understanding where MGAs come from, how they work, and how they are used, readers will gain a thorough appreciation for the impact these devices have on contemporary technology. Let us begin by defining and tracing the origins of MGAs.
Definition and Origins of MGA
The concept of MGA, or Managed General Agency, is a crucial component in the insurance industry, facilitating the distribution and underwriting of insurance policies. To fully understand the significance of MGA, it is essential to delve into its definition and origins. This article will explore the historical context of MGA development, highlighting the key events and factors that led to its emergence. Additionally, we will examine the key components and features that define an MGA, including its role in risk assessment and policy issuance. Finally, we will discuss the evolution of MGA over time, tracing how it has adapted to changing market conditions and technological advancements. By understanding these aspects, readers will gain a comprehensive insight into the world of MGAs. Let us begin by examining the historical context of MGA development, which sets the stage for understanding its current role and future trajectory.
Historical Context of MGA Development
The historical context of MGA (Managed Graphics Acceleration) development is deeply intertwined with the evolution of computer graphics and the need for efficient, high-performance rendering. In the early 1980s, as personal computers began to gain widespread use, there was a growing demand for improved graphical capabilities. This period saw the emergence of specialized graphics processing units (GPUs) designed to offload complex graphics computations from the central processing unit (CPU), thereby enhancing overall system performance. One of the pivotal moments in MGA's development was the introduction of the IBM PS/2 line in 1987, which included the MGA (Micro Channel Graphics Adapter) card. This adapter was designed to take advantage of IBM's Micro Channel architecture, providing a significant boost in graphics performance compared to earlier standards like CGA (Color Graphics Adapter) and EGA (Enhanced Graphics Adapter). The MGA card supported higher resolutions and more colors, making it a crucial step towards modern graphical standards. The late 1980s and early 1990s saw further advancements with the introduction of VGA (Video Graphics Array) and later SVGA (Super Video Graphics Array), which continued to push the boundaries of what was possible in terms of resolution and color depth. However, these standards were still limited by their reliance on shared system memory and lack of dedicated graphics processing power. The true catalyst for modern MGA development came with the advent of dedicated GPUs from companies like NVIDIA and ATI (now AMD). These GPUs not only accelerated 2D and 3D graphics but also introduced programmable shaders, transforming the landscape of computer graphics. By the late 1990s, GPUs had become indispensable components in gaming PCs and workstations, driving innovation in fields such as gaming, video editing, and scientific visualization. In parallel, advancements in APIs like DirectX and OpenGL facilitated better communication between software applications and hardware accelerators. These APIs standardized how developers could leverage GPU capabilities, leading to more efficient and powerful graphics rendering across various platforms. Today, MGA continues to evolve alongside advancements in GPU technology. Modern GPUs are capable of handling complex tasks such as ray tracing, artificial intelligence-enhanced rendering, and virtual reality applications. The historical context of MGA development underscores a continuous quest for improved performance, efficiency, and innovation in computer graphics, reflecting broader technological trends and user demands over several decades. This evolution has transformed not just how we interact with computers but also how we experience visual content across various industries.
Key Components and Features
Key components and features of a Master Games Agreement (MGA) are crucial for understanding its role and significance in the gaming industry. An MGA typically includes several essential elements that define the terms and conditions under which a game is developed, published, and distributed. 1. **Scope of Work**: This section outlines the specific responsibilities of each party involved, including the developer's obligations to create the game and the publisher's duties to market and distribute it. 2. **Intellectual Property Rights**: The MGA clearly defines who owns the intellectual property (IP) rights to the game, including characters, storylines, and other creative assets. This is vital for ensuring that both parties understand their rights and limitations. 3. **Development Milestones**: The agreement specifies key milestones that the developer must meet during the game's development process. These milestones often trigger payments or other obligations. 4. **Payment Terms**: This component details how payments will be made, including any advances, royalties, and other financial arrangements. It may also include provisions for bonuses or penalties based on performance metrics. 5. **Quality Standards**: To ensure that the final product meets industry standards, the MGA may include quality benchmarks that the game must adhere to before it is considered complete. 6. **Testing and Approval**: The agreement often outlines a process for testing and approving the game at various stages of development. This ensures that both parties are satisfied with the game's quality before its release. 7. **Marketing and Distribution**: This section covers how the game will be marketed and distributed, including responsibilities for advertising, packaging, and distribution channels. 8. **Warranties and Representations**: Both parties typically make certain warranties and representations about their capabilities and the game itself, such as ensuring that the game does not infringe on third-party rights. 9. **Termination Clauses**: The MGA includes provisions for terminating the agreement under specific conditions, such as failure to meet milestones or breach of contract. 10. **Dispute Resolution**: To handle any disputes that may arise, the agreement often includes mechanisms for resolving conflicts, such as arbitration or mediation. 11. **Confidentiality**: Given the sensitive nature of game development, MGAs often include confidentiality clauses to protect proprietary information shared between parties. 12. **Licensing Agreements**: If third-party licenses are involved (e.g., for music or characters), these agreements will be referenced within the MGA to ensure compliance with all licensing terms. Understanding these key components and features is essential for both game developers and publishers to navigate the complex landscape of game development successfully while protecting their interests and ensuring a mutually beneficial partnership.
Evolution Over Time
Evolution over time is a fundamental concept that underpins the development and diversification of life on Earth. It is the process by which species change through successive generations, driven by genetic variations, environmental pressures, and natural selection. This gradual transformation can lead to the emergence of new traits, the adaptation to changing environments, and ultimately, the formation of new species. In the context of the Definition and Origins of MGA (Molecular Graphics Algorithm), understanding evolution is crucial because it highlights the dynamic nature of biological systems. MGA, which is used in computational biology to visualize and analyze molecular structures, must account for the evolutionary changes that occur in proteins and other biomolecules over time. These changes can affect the structure, function, and interactions of molecules, making it essential for MGA to incorporate evolutionary data to provide accurate and meaningful insights. The evolutionary process begins with genetic variation, which arises from mutations, gene flow, and genetic recombination. These variations can result in differences in traits among individuals within a population. Environmental factors such as climate, predation, and competition then act on these variations through natural selection, favoring individuals with traits that enhance their survival and reproductive success. Over many generations, this selective pressure can lead to the accumulation of beneficial traits, driving evolutionary change. For instance, antibiotic resistance in bacteria is a clear example of evolution in action. When exposed to antibiotics, susceptible bacteria die off, leaving behind resistant strains that have genetic mutations conferring survival advantages. These resistant bacteria then reproduce, passing on their resistant genes to their offspring, thereby spreading resistance through the population. In addition to natural selection, other mechanisms like genetic drift and gene flow also contribute to evolutionary change. Genetic drift involves random changes in allele frequencies within a population, which can lead to the loss or fixation of alleles. Gene flow, on the other hand, refers to the movement of genes from one population to another, which can introduce new genetic variations and increase genetic diversity. Understanding these evolutionary processes is vital for developing effective MGAs. By integrating evolutionary information into molecular graphics algorithms, researchers can better predict protein structure and function, identify conserved regions across different species, and design drugs that target specific molecular interactions. This integration enhances the accuracy and utility of MGA outputs, making them more relevant for applications in fields such as drug discovery and personalized medicine. In summary, evolution over time is a cornerstone of biological science that shapes the diversity of life on Earth. Its principles are essential for understanding how biological systems change and adapt, which in turn informs the development of sophisticated tools like MGA. By acknowledging and incorporating evolutionary dynamics into computational models, scientists can gain deeper insights into molecular biology and develop more effective solutions for various biomedical challenges.
Technical Aspects and Functionality
When delving into the technical aspects and functionality of a system, it is crucial to consider several key elements that collectively contribute to its overall performance and usability. First, the architectural design and structure form the foundation upon which all other components are built, influencing scalability, maintainability, and efficiency. Second, performance metrics and benchmarks provide quantifiable measures to evaluate the system's speed, reliability, and capacity, allowing for continuous improvement. Third, integration with other technologies ensures seamless interoperability and enhances the system's versatility. By examining these facets, developers can create robust, high-performing systems that meet diverse user needs. This article will explore each of these critical areas in depth, starting with the architectural design and structure, which sets the stage for a well-engineered and effective system.
Architectural Design and Structure
Architectural design and structure are the cornerstone of any building, ensuring both aesthetic appeal and functional integrity. When it comes to technical aspects and functionality, several key elements come into play. **Load-bearing capacity** is crucial, as it determines how much weight a structure can support without compromising its stability. This involves careful consideration of materials such as steel, concrete, and wood, each with its own strengths and limitations. For instance, steel is renowned for its high tensile strength and durability, making it ideal for skyscrapers and large-scale constructions. **Structural systems** also play a vital role in maintaining the integrity of a building. These include beams, columns, and foundations that work together to distribute loads evenly. The choice of structural system—whether it be a simple beam-column system or a more complex truss system—depends on the building's intended use and environmental conditions. For example, buildings in seismic zones require specialized structural designs that can withstand earthquakes, such as seismic-resistant frames. **Building codes and regulations** are another critical aspect of architectural design. These standards ensure that structures meet minimum safety requirements and are designed to withstand various environmental factors like wind, rain, and extreme temperatures. Compliance with these codes not only protects occupants but also helps in maintaining the longevity of the building. In addition to structural considerations, **sustainability** has become a significant focus in modern architectural design. Architects now incorporate green building techniques and materials to reduce the environmental footprint of their creations. This includes using recycled materials, optimizing energy efficiency through smart building technologies, and integrating natural ventilation systems. **Functionality** is equally important as it directly impacts the usability and comfort of a building. This involves designing spaces that are efficient, accessible, and meet the needs of their intended users. For example, in office buildings, open floor plans can enhance collaboration among employees while also allowing for flexible workspace arrangements. In residential buildings, functional design might include features like smart home automation systems that enhance convenience and energy efficiency. Lastly, **innovative technologies** are revolutionizing architectural design by offering new possibilities for both form and function. Advances in computer-aided design (CAD) software allow architects to create complex models with precision and speed. Additionally, technologies like 3D printing are enabling the creation of intricate structures that would be impossible to build using traditional methods. In summary, architectural design and structure are intertwined with technical aspects such as load-bearing capacity, structural systems, compliance with building codes, sustainability considerations, functional design principles, and the integration of innovative technologies. Each of these elements must be carefully considered to ensure that a building not only stands the test of time but also serves its purpose effectively.
Performance Metrics and Benchmarks
Performance metrics and benchmarks are crucial components in evaluating the effectiveness and efficiency of any system, including an MGA (Managed General Agency). These metrics serve as quantifiable measures to assess performance against predefined standards or benchmarks, enabling stakeholders to make informed decisions. Here are some key aspects: 1. **Response Time**: This metric measures how quickly a system responds to user inputs. For an MGA, it could be the time taken to process insurance claims or generate policy quotes. 2. **Throughput**: This indicates the volume of work that can be processed within a given timeframe. In an MGA context, it might refer to the number of policies issued or claims settled per day. 3. **Accuracy**: This measures the correctness of the output. For instance, the accuracy of underwriting decisions or the precision of premium calculations. 4. **Uptime and Downtime**: These metrics track the availability of the system. High uptime ensures that the system is accessible when needed, while minimizing downtime reduces operational disruptions. 5. **Customer Satisfaction**: Often measured through surveys or feedback forms, this metric gauges how well the system meets user expectations and needs. Benchmarks, on the other hand, provide a baseline for comparison. These can be industry standards, internal targets, or historical data. By comparing performance metrics against these benchmarks, an MGA can identify areas for improvement and optimize its operations. For example: - **Industry Benchmarks**: Comparing response times with industry averages can help an MGA determine if its systems are performing at par with competitors. - **Internal Targets**: Setting internal benchmarks for throughput can help an MGA measure its progress towards operational goals. - **Historical Data**: Comparing current performance metrics with past data allows an MGA to track trends and improvements over time. Incorporating these metrics and benchmarks into the technical aspects and functionality of an MGA ensures that the system is not only functional but also efficient and effective in meeting its objectives. This approach fosters continuous improvement by providing clear, data-driven insights that guide strategic decisions and operational enhancements.
Integration with Other Technologies
Integration with other technologies is a crucial aspect of the functionality and technical aspects of an MGA (Managing General Agent). An MGA operates as a intermediary between insurance carriers and policyholders, and its ability to integrate seamlessly with various technologies enhances its efficiency, scalability, and overall performance. Here’s how integration plays a vital role: 1. **APIs and Data Exchange**: MGAs leverage Application Programming Interfaces (APIs) to facilitate data exchange between different systems. This allows for real-time updates, automated underwriting processes, and streamlined claims handling. APIs enable MGAs to connect with insurance carriers' systems, policy administration systems, and other third-party services such as credit scoring agencies or medical records databases. 2. **CRM Systems**: Integration with Customer Relationship Management (CRM) systems helps MGAs manage client interactions more effectively. By syncing data from CRM systems, MGAs can track client history, preferences, and communication records, ensuring personalized service and improved customer satisfaction. 3. **Policy Administration Systems**: These systems are essential for managing the lifecycle of insurance policies. Integration here ensures that policy issuance, renewals, cancellations, and amendments are processed efficiently without manual intervention. This reduces errors and enhances the speed of service delivery. 4. **Claims Management Software**: Seamless integration with claims management software allows MGAs to handle claims more efficiently. Automated workflows for claims processing, documentation, and communication with adjusters and policyholders streamline the entire claims process. 5. **Analytics and Reporting Tools**: Advanced analytics tools integrated into an MGA's system provide valuable insights into market trends, risk assessment, and operational performance. These insights help in making informed decisions regarding underwriting policies, pricing strategies, and resource allocation. 6. **Cloud Computing**: Cloud integration offers scalability, flexibility, and cost savings. MGAs can scale their operations quickly without significant capital expenditures on hardware or infrastructure. Cloud-based solutions also ensure high availability and disaster recovery capabilities. 7. **Cybersecurity Measures**: Integration with robust cybersecurity measures is critical to protect sensitive client data. MGAs must integrate advanced security protocols such as encryption, firewalls, and intrusion detection systems to safeguard against cyber threats. 8. **Compliance Software**: Ensuring compliance with regulatory requirements is paramount for MGAs. Integration with compliance software helps in monitoring and adhering to industry standards, reducing the risk of non-compliance penalties. In summary, the integration of an MGA with various technologies not only enhances its operational efficiency but also improves customer experience through faster service delivery and better decision-making capabilities. By leveraging APIs, CRM systems, policy administration systems, claims management software, analytics tools, cloud computing solutions, cybersecurity measures, and compliance software, MGAs can position themselves as agile and reliable partners in the insurance ecosystem. This holistic approach to technology integration underscores the technical sophistication and functional capabilities that define a modern MGA.
Applications and Real-World Use Cases
The applications and real-world use cases of advanced technologies are vast and multifaceted, touching various aspects of our lives. This article delves into three key areas: Industrial and Commercial Uses, Research and Development Applications, and Future Trends and Potential Innovations. In the industrial and commercial sectors, these technologies are revolutionizing production processes, enhancing efficiency, and driving innovation. For instance, automation and AI are transforming manufacturing by optimizing workflows and reducing costs. In research and development, these technologies are facilitating groundbreaking discoveries and advancements in fields such as medicine, energy, and materials science. Looking ahead, future trends and potential innovations promise even more transformative impacts, from sustainable solutions to cutting-edge consumer products. By exploring these areas, we gain a comprehensive understanding of how these technologies are shaping our present and future. Let us begin by examining the significant role these advancements play in Industrial and Commercial Uses.
Industrial and Commercial Uses
Industrial and commercial uses of Magnesium Alloys (MgAs) are diverse and significant, leveraging their unique properties such as high strength-to-weight ratio, excellent corrosion resistance, and good thermal conductivity. In the aerospace industry, MgAs are used in aircraft components due to their lightweight nature, which enhances fuel efficiency and reduces overall weight. For instance, magnesium alloys are found in engine mounts, gearboxes, and other structural parts where weight reduction is critical without compromising on strength. In the automotive sector, MgAs are utilized in vehicle parts like engine blocks, gearboxes, and steering components to improve fuel efficiency and reduce emissions. The automotive industry also benefits from magnesium's ability to absorb vibrations, making it ideal for use in dashboards and other interior components. Additionally, magnesium alloys are used in die-casting processes to produce complex shapes with high precision, which is particularly advantageous for producing parts such as transmission cases and engine blocks. The electronics industry also relies heavily on MgAs due to their excellent thermal conductivity and electromagnetic shielding properties. For example, magnesium alloys are used in laptop casings and other electronic devices where heat dissipation is crucial. Furthermore, their non-magnetic nature makes them suitable for use in applications requiring minimal interference with electromagnetic fields. In construction and architecture, magnesium alloys find application in building materials due to their fire-resistant properties and ability to withstand extreme temperatures. This makes them ideal for use in fireproofing systems and structural components that require high durability. Moreover, MgAs have medical applications due to their biocompatibility and ability to degrade at a controlled rate within the body. This property makes them suitable for use in biodegradable implants such as bone screws and plates that can dissolve over time as the body heals. Lastly, magnesium alloys are also used in sports equipment like bicycle frames and golf clubs where lightweight yet strong materials are essential for performance enhancement. Their corrosion resistance also makes them suitable for marine applications such as boat propellers and other marine hardware. Overall, the versatility of magnesium alloys across various industries underscores their importance in modern manufacturing and engineering practices. Their unique combination of physical properties makes them an indispensable material for a wide range of industrial and commercial applications.
Research and Development Applications
Research and Development (R&D) applications are pivotal in driving innovation and solving real-world problems across various industries. In the context of applications and real-world use cases, R&D plays a crucial role in transforming theoretical concepts into practical solutions. Here, we delve into the significant impact of R&D in several key areas. **Technological Advancements:** R&D is the backbone of technological progress, enabling the development of new products, processes, and services. For instance, in the tech industry, R&D has led to breakthroughs such as artificial intelligence (AI), blockchain technology, and the Internet of Things (IoT). These innovations have revolutionized how businesses operate and how consumers interact with technology. **Healthcare:** In healthcare, R&D is instrumental in discovering new treatments and medications. Pharmaceutical companies invest heavily in R&D to develop drugs that combat diseases like cancer, HIV, and COVID-19. Additionally, medical device manufacturers rely on R&D to create advanced diagnostic tools and therapeutic equipment, improving patient outcomes and quality of life. **Sustainability:** As the world grapples with environmental challenges, R&D in sustainability has become increasingly important. Researchers are developing renewable energy sources like solar and wind power, as well as more efficient energy storage solutions such as advanced batteries. This work is crucial for reducing carbon footprints and mitigating climate change. **Manufacturing:** In manufacturing, R&D focuses on improving production processes and materials. This includes the development of advanced materials like nanomaterials and smart materials, which enhance product performance and durability. Moreover, R&D in manufacturing automation has led to the adoption of Industry 4.0 technologies, such as robotics and machine learning, which optimize production efficiency and reduce costs. **Agriculture:** Agricultural R&D aims to increase crop yields, improve food quality, and enhance agricultural sustainability. Scientists are working on genetically modified crops that are more resilient to pests and environmental stressors. Precision agriculture, another area of focus, uses data analytics and IoT to optimize farming practices, reducing waste and increasing productivity. **Transportation:** The transportation sector benefits significantly from R&D, particularly in the development of electric vehicles (EVs) and autonomous driving technologies. EVs are becoming more mainstream due to advancements in battery technology, while autonomous vehicles are being tested for safety and efficiency. These innovations promise to transform urban mobility and reduce emissions. In summary, R&D applications are essential for driving innovation across multiple sectors. By investing in R&D, organizations can develop new technologies, improve existing processes, and address pressing global challenges. The real-world use cases of R&D underscore its critical role in shaping the future of various industries and improving the quality of life for individuals worldwide.
Future Trends and Potential Innovations
As we delve into the future trends and potential innovations in the realm of Microbial Genome Assembly (MGA), several exciting developments come to the forefront. One of the most promising areas is the integration of advanced computational tools and artificial intelligence (AI) to enhance assembly accuracy and speed. AI algorithms can help in identifying and correcting errors more efficiently, leading to more reliable and complete genome assemblies. Additionally, the rise of long-read sequencing technologies, such as those offered by PacBio and Oxford Nanopore, will continue to play a crucial role in overcoming the limitations of short-read data, enabling better resolution of repetitive regions and complex genomic structures. Another significant trend is the increasing focus on single-cell genomics, which allows for the assembly of individual microbial genomes from mixed communities. This approach will revolutionize our understanding of microbial diversity and function within ecosystems, enabling precise tracking of specific strains and their roles in various environments. Furthermore, advancements in metagenomic assembly will allow researchers to reconstruct genomes directly from environmental samples without the need for culturing, opening up new avenues for discovering novel microbial species and their potential applications. The integration of MGA with other 'omics' technologies, such as transcriptomics and proteomics, will also become more prevalent. This multi-omics approach will provide a holistic view of microbial function, allowing scientists to correlate genomic data with gene expression and protein activity. This comprehensive understanding will be pivotal in fields like biotechnology, where engineered microbes are used for biofuel production, bioremediation, and the development of novel therapeutics. Moreover, the application of MGA in personalized medicine is expected to grow. By analyzing the genomic content of an individual's microbiome, healthcare providers can tailor treatments to address specific microbial imbalances or pathogenic infections more effectively. This personalized approach could lead to breakthroughs in treating chronic diseases influenced by the microbiome, such as inflammatory bowel disease and mental health disorders. In agriculture, MGA will play a critical role in improving crop yields and disease resistance by understanding the genomic makeup of beneficial soil microbes and plant pathogens. This knowledge can be used to develop targeted biofertilizers and biopesticides, reducing the reliance on chemical inputs and promoting sustainable farming practices. Lastly, the ethical and regulatory frameworks surrounding MGA are likely to evolve as its applications expand. Ensuring data privacy, addressing biosafety concerns, and establishing standards for genomic data sharing will be essential to harnessing the full potential of MGA while mitigating potential risks. In summary, the future of MGA is marked by technological advancements, interdisciplinary integration, and diverse real-world applications. As these trends continue to unfold, we can expect significant contributions to various fields, from biotechnology and personalized medicine to agriculture and environmental science, ultimately driving innovation and improving human well-being.