What Is Qst
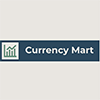
Quantum Simulation Technology (QST) is a revolutionary field that leverages the principles of quantum mechanics to simulate complex systems, offering unprecedented insights into the behavior of matter at the atomic and subatomic level. This cutting-edge technology has the potential to transform various scientific disciplines and industrial sectors by providing accurate and efficient simulations that were previously unattainable with classical computing. In this article, we will delve into the multifaceted world of QST, starting with **Understanding the Basics of QST**, where we will explore the fundamental concepts and mechanisms that underpin this technology. We will then examine **Applications and Uses of QST**, highlighting how it is being utilized across diverse fields such as chemistry, materials science, and pharmaceuticals. Finally, we will look ahead to **Future Prospects and Innovations in QST**, discussing the exciting advancements and potential breakthroughs that are on the horizon. By grasping these core aspects, readers will gain a comprehensive understanding of QST's current state and its promising future. Let us begin by understanding the basics of QST.
Understanding the Basics of QST
Understanding the Basics of QST is a foundational step for anyone delving into the realm of quantum science and technology. This complex and fascinating field has its roots in the early 20th century, with pioneers like Max Planck and Albert Einstein laying the groundwork. To grasp QST, it is essential to explore its **Definition and Origins**, which delve into the fundamental principles that distinguish quantum mechanics from classical physics. Additionally, understanding the **Key Components and Structure** of QST is crucial, as it involves comprehending concepts such as superposition, entanglement, and wave-particle duality. Furthermore, appreciating the **Historical Context and Evolution** of QST provides insight into how these principles have developed over time, from theoretical breakthroughs to practical applications. By examining these aspects, readers can gain a comprehensive understanding of the basics of QST, setting the stage for deeper exploration and application in this rapidly advancing field. This article will guide you through these essential elements, ensuring a solid foundation in Understanding the Basics of QST.
Definition and Origins
**Understanding the Basics of QST: Definition and Origins** Quantum State Tomography (QST) is a fundamental technique in quantum information science that allows for the complete characterization of quantum states. At its core, QST involves reconstructing the density matrix of a quantum system from a set of measurement outcomes. This process is crucial because it provides a detailed understanding of the quantum state's properties, which are essential for various applications in quantum computing, quantum communication, and quantum metrology. The origins of QST can be traced back to the early days of quantum mechanics when physicists began exploring ways to describe and measure quantum systems. The concept gained significant traction in the 1990s with the advent of quantum information theory. Researchers like David Finkelstein and others laid the groundwork by proposing methods to reconstruct quantum states from measurement data. One of the key milestones was the introduction of the "quantum state tomography" term itself, which encapsulated the idea of systematically measuring and reconstructing quantum states. The mathematical underpinnings of QST are rooted in linear algebra and statistical estimation. The density matrix, a central object in quantum mechanics, represents the state of a quantum system. By performing a series of measurements on multiple copies of the system, one can gather enough data to reconstruct this matrix. This involves solving an inverse problem where the measured outcomes are used to estimate the parameters of the density matrix. Various algorithms and techniques have been developed to optimize this process, including maximum likelihood estimation and Bayesian methods. The importance of QST extends beyond theoretical curiosity; it has practical implications for verifying the performance of quantum devices. For instance, in quantum computing, QST is used to validate the operation of quantum gates and ensure that quantum bits (qubits) are correctly prepared and manipulated. In quantum communication, QST helps in assessing the fidelity of quantum channels, which is vital for secure communication protocols like quantum key distribution. In summary, Quantum State Tomography is a powerful tool that enables the comprehensive characterization of quantum states. Its origins are deeply intertwined with the evolution of quantum information science, and its applications are pivotal in advancing our understanding and control over quantum systems. As research in quantum technologies continues to advance, QST remains an indispensable technique for ensuring the accuracy and reliability of these systems.
Key Components and Structure
Understanding the basics of Quantum Signal Processing (QST) hinges on grasping its key components and structure. At its core, QST involves the manipulation and analysis of quantum signals, which are fundamentally different from their classical counterparts due to the principles of quantum mechanics. The structure of QST can be broken down into several critical elements. First, **quantum states** form the foundation. These states are described by wave functions or density matrices and can exist in superposition and entanglement, allowing for parallel processing and enhanced computational power. **Quantum gates**, analogous to logic gates in classical computing, are the building blocks for quantum algorithms. These gates perform operations on qubits (quantum bits), enabling tasks such as quantum teleportation and superdense coding. Another crucial component is **quantum measurement**, which extracts information from quantum systems. Unlike classical measurements, quantum measurements are probabilistic and can collapse the superposition of states, making them irreversible. **Quantum error correction** is also essential due to the fragile nature of quantum states; techniques like quantum error correction codes help mitigate decoherence and maintain the integrity of quantum information. The **quantum circuit model** provides a framework for designing and implementing quantum algorithms. This model consists of a sequence of quantum gates applied to qubits, allowing for the systematic construction of complex quantum operations. **Quantum algorithms**, such as Shor's algorithm for factorization and Grover's algorithm for search, leverage these components to solve problems exponentially faster than their classical counterparts in certain cases. Furthermore, **quantum communication protocols** like quantum key distribution (QKD) utilize the principles of QST to ensure secure communication. These protocols exploit the no-cloning theorem and entanglement to detect eavesdropping, thereby guaranteeing the confidentiality of transmitted information. In addition, **quantum control and calibration** are vital for maintaining the coherence of quantum systems. Advanced techniques such as dynamical decoupling and pulse shaping help mitigate noise and ensure precise control over quantum operations. Lastly, **quantum information theory** provides the theoretical underpinnings for understanding the limits and capabilities of QST. It encompasses concepts like quantum entropy, mutual information, and channel capacity, which are crucial for optimizing quantum communication and computation. By understanding these key components and their interplay within the structure of QST, one can appreciate the profound implications and potential applications of quantum signal processing in fields ranging from cryptography to materials science. This foundational knowledge is essential for advancing research and development in this rapidly evolving field.
Historical Context and Evolution
Understanding the Basics of QST begins with a deep dive into its historical context and evolution. Quantum Science and Technology (QST) has its roots in the early 20th century, when groundbreaking discoveries in quantum mechanics by pioneers like Max Planck, Albert Einstein, and Niels Bohr laid the foundation for what would become a revolutionary field. Initially, these principles were applied in theoretical physics to explain phenomena at the atomic and subatomic levels. However, as technology advanced, the practical applications of quantum mechanics began to emerge. The post-World War II era saw significant investments in scientific research, leading to the development of quantum electronics and the invention of the transistor by John Bardeen, Walter Brattain, and William Shockley in 1947. This innovation marked a pivotal moment in the evolution of QST, as it enabled the miniaturization of electronic devices and paved the way for modern computing. In the 1960s and 1970s, quantum optics and laser technology became prominent areas of research. The invention of the laser by Theodore Maiman in 1960 opened up new avenues for studying light-matter interactions and led to advancements in fields such as spectroscopy and interferometry. These developments were crucial for understanding quantum phenomena in controlled environments. The 1980s witnessed a surge in interest in quantum computing, sparked by Paul Benioff's proposal for a quantum Turing machine in 1982 and David Deutsch's formulation of the quantum algorithm in 1985. This period also saw the introduction of quantum cryptography by Charles Bennett and Gilles Brassard, which provided a secure method for encrypting data using quantum mechanics. In recent decades, QST has continued to evolve rapidly. Advances in materials science have led to the development of superconducting qubits and topological quantum computing. The advent of quantum simulation, pioneered by researchers like Seth Lloyd and Peter Shor, has allowed scientists to study complex quantum systems that are intractable with classical computers. Furthermore, breakthroughs in quantum error correction and quantum algorithms have brought us closer to practical applications of quantum computing. Today, QST encompasses a broad spectrum of disciplines including quantum computing, quantum communication, quantum simulation, and quantum metrology. Governments and private companies are investing heavily in QST research, recognizing its potential to solve complex problems in fields such as medicine, finance, and climate modeling. As we continue to explore the frontiers of quantum science and technology, it is clear that understanding its historical context and evolution is essential for appreciating its current state and future possibilities. This historical perspective not only highlights the achievements of past scientists but also underscores the collaborative and interdisciplinary nature of QST, which continues to drive innovation and push the boundaries of human knowledge.
Applications and Uses of QST
Quantum Sensing Technology (QST) has emerged as a revolutionary field, leveraging the unique properties of quantum mechanics to enhance precision and accuracy in various domains. This cutting-edge technology has far-reaching implications across multiple sectors, making it a cornerstone for innovation. In industrial and commercial applications, QST is transforming industries such as navigation, timing, and materials science by offering unprecedented levels of sensitivity and reliability. Scientific research and development also benefit significantly from QST, enabling breakthroughs in fields like magnetometry, spectroscopy, and quantum computing. Moreover, everyday life and consumer products are being influenced by QST, with potential applications in healthcare, environmental monitoring, and consumer electronics. As we delve into the diverse applications and uses of QST, it becomes clear that understanding the basics of this technology is crucial for appreciating its full potential. Therefore, this article will explore these three key areas in detail, providing a comprehensive overview that transitions seamlessly into understanding the fundamentals of Quantum Sensing Technology.
Industrial and Commercial Applications
Industrial and commercial applications of Quantum Simulation Technology (QST) are revolutionizing various sectors by offering unprecedented insights and efficiencies. In the realm of materials science, QST enables the precise simulation of material properties at the atomic level, allowing for the design of new materials with tailored characteristics such as enhanced strength, conductivity, or thermal resistance. This capability is particularly valuable in industries like aerospace, where lightweight yet robust materials are crucial for reducing fuel consumption and increasing safety. In the pharmaceutical industry, QST facilitates the simulation of complex molecular interactions, accelerating drug discovery and development. By accurately modeling the behavior of molecules, researchers can predict the efficacy and potential side effects of new compounds more reliably than ever before. This not only speeds up the drug development process but also reduces the need for costly and time-consuming physical trials. The energy sector also benefits significantly from QST. Simulations can optimize the performance of solar cells and fuel cells by predicting how different materials will interact under various conditions. Additionally, QST can help in the design of more efficient energy storage systems such as batteries, which is critical for widespread adoption of renewable energy sources. In manufacturing, QST can be used to simulate and optimize production processes. For instance, it can predict how different parameters will affect the quality of products in real-time, allowing for immediate adjustments to ensure consistent output. This real-time optimization can lead to significant reductions in waste and improvements in product quality. Furthermore, QST has profound implications for logistics and supply chain management. By simulating complex systems and predicting potential bottlenecks or disruptions, companies can make more informed decisions about inventory management, transportation routes, and resource allocation. This predictive capability helps in minimizing delays and maximizing operational efficiency. In the field of finance, QST can be applied to simulate complex financial models and predict market behaviors with greater accuracy. This allows financial institutions to better manage risk, optimize investment strategies, and make more informed decisions about asset allocation. Overall, the industrial and commercial applications of QST are vast and transformative. By leveraging quantum simulation capabilities, businesses across various sectors can achieve significant advancements in efficiency, innovation, and profitability. As QST continues to evolve, its potential to drive technological breakthroughs and economic growth will only continue to expand.
Scientific Research and Development
Scientific research and development (R&D) are the cornerstone of advancing our understanding and application of Quantum Science and Technology (QST). This rigorous process involves systematic investigation and experimentation to develop new or improved products, processes, and services. In the context of QST, R&D is crucial for unlocking the full potential of quantum mechanics, which promises revolutionary changes in various fields. At the forefront of QST R&D are researchers from diverse disciplines, including physics, engineering, computer science, and materials science. These scientists engage in cutting-edge experiments and simulations to explore phenomena such as superposition, entanglement, and quantum interference. The insights gained from these studies are then translated into practical applications, such as quantum computing, quantum cryptography, and quantum sensing. Quantum computing, for instance, leverages quantum bits (qubits) to perform calculations exponentially faster than classical computers for certain problems. This has significant implications for fields like cryptography, optimization problems, and drug discovery. Researchers are actively developing quantum algorithms and hardware to overcome current limitations and achieve scalability. Another critical area is quantum cryptography, which exploits the principles of quantum mechanics to create unbreakable encryption methods. Quantum key distribution (QKD) protocols ensure secure communication by detecting any attempt to eavesdrop on the transmission. This technology is being integrated into secure communication networks to protect sensitive information. Quantum sensing is another promising application where highly sensitive quantum systems are used to measure physical parameters such as magnetic fields, temperature, and pressure with unprecedented precision. This has far-reaching implications for navigation, materials science, and medical imaging. The R&D process in QST also involves significant collaboration between academia, industry, and government. Funding agencies and private investors support research initiatives that aim to bridge the gap between theoretical advancements and practical implementations. Additionally, international collaborations facilitate the sharing of resources, expertise, and knowledge to accelerate progress. In conclusion, scientific research and development are essential for harnessing the power of QST. Through continuous innovation and experimentation, scientists are transforming theoretical concepts into tangible technologies that promise to revolutionize various sectors. As R&D continues to advance, we can expect significant breakthroughs that will shape the future of technology and society.
Everyday Life and Consumer Products
In the realm of everyday life, consumer products play a pivotal role in enhancing convenience, comfort, and efficiency. These products, often overlooked but always present, are the unsung heroes that make our daily routines smoother and more enjoyable. From the moment we wake up to the time we go to bed, we interact with a myriad of consumer goods that are designed to simplify tasks and improve our quality of life. For instance, kitchen appliances like refrigerators, microwaves, and dishwashers save us time and effort in meal preparation and cleanup. Personal care items such as toothbrushes, shampoos, and skincare products help maintain hygiene and appearance. Home cleaning tools like vacuum cleaners and laundry machines ensure our living spaces remain tidy and clean. The integration of advanced technologies into these consumer products has significantly elevated their performance and user experience. For example, smart home devices like thermostats and lighting systems can be controlled remotely through smartphones, allowing for greater energy efficiency and convenience. Wearable technology such as fitness trackers and smartwatches monitor health metrics in real-time, providing valuable insights that can guide healthier lifestyles. Even something as mundane as a coffee maker can now be programmed to brew coffee at a specific time each day, ensuring a fresh cup is ready when you need it. Moreover, the development of these consumer products is often driven by innovative materials and technologies. Quantum Science and Technology (QST), for instance, has the potential to revolutionize various aspects of consumer product design. QST can lead to breakthroughs in energy storage through more efficient batteries, enabling longer-lasting power for portable devices. It can also enhance the performance of electronic components, making them faster, smaller, and more reliable. Additionally, QST could pave the way for new materials with unique properties that could be used in everything from durable cookware to advanced textiles. The applications of QST in consumer products are vast and promising. Imagine refrigerators that use quantum-inspired cooling systems to be more energy-efficient or smartphones with quantum-enhanced processors that offer unparalleled speed and security. These advancements not only improve the functionality of consumer goods but also contribute to a more sustainable future by reducing energy consumption and waste. In conclusion, everyday life is intricately woven with consumer products that make our lives easier, more comfortable, and more enjoyable. As technology continues to evolve, particularly with the advent of Quantum Science and Technology, we can expect even more innovative solutions that will further transform our daily experiences. The integration of QST into consumer products holds immense potential for creating smarter, greener, and more efficient living environments, underscoring its critical role in shaping the future of everyday life.
Future Prospects and Innovations in QST
As we stand at the forefront of a new era in Quantum Science and Technology (QST), the landscape is abuzz with excitement and anticipation. The future prospects and innovations in QST are poised to revolutionize various fields, from computing and cryptography to materials science and medicine. This article delves into the emerging technologies and trends that are shaping the QST landscape, highlighting advancements such as quantum computing, quantum communication networks, and quantum simulation. We also explore potential breakthroughs and discoveries that could transform our understanding of quantum mechanics and its applications. However, these advancements come with their own set of challenges and opportunities ahead, requiring careful navigation to fully harness their potential. By understanding these dynamics, we can better appreciate the transformative power of QST. To fully grasp these concepts, it is essential to start by understanding the basics of QST, which will be discussed in detail later in this article.
Emerging Technologies and Trends
In the realm of Quantum Science and Technology (QST), emerging technologies and trends are revolutionizing the landscape with unprecedented potential. At the forefront is the development of quantum computing, which promises to solve complex problems that are currently intractable for classical computers. Quantum processors, such as those being developed by companies like Google and IBM, leverage quantum bits (qubits) to perform calculations exponentially faster than their classical counterparts. This advancement holds significant implications for fields like cryptography, drug discovery, and climate modeling. Another pivotal trend is the integration of quantum communication systems, particularly quantum key distribution (QKD). QKD enables secure data transmission by exploiting the principles of quantum mechanics to encode and decode messages in a way that any attempt to intercept would be detectable. This technology is crucial for safeguarding sensitive information in industries such as finance and defense. Advances in quantum sensing are also transforming various sectors. Quantum sensors, which utilize the unique properties of quantum systems to measure physical parameters with extraordinary precision, are being applied in navigation, geophysics, and materials science. For instance, quantum magnetometers can detect subtle changes in magnetic fields, enhancing the accuracy of GPS systems and mineral exploration. Furthermore, the field of quantum simulation is gaining momentum. Quantum simulators can mimic the behavior of complex quantum systems, allowing researchers to study phenomena that are difficult or impossible to replicate classically. This capability is driving breakthroughs in understanding chemical reactions, material properties, and even the behavior of subatomic particles. The convergence of QST with other technologies like artificial intelligence (AI) and machine learning (ML) is opening new avenues for innovation. Quantum machine learning algorithms can process vast amounts of data more efficiently than classical algorithms, potentially leading to significant advancements in areas such as pattern recognition and predictive analytics. Additionally, the development of quantum-resistant cryptography is becoming increasingly important as the advent of powerful quantum computers threatens to compromise current encryption methods. Researchers are working on post-quantum cryptographic protocols that can withstand attacks from both classical and quantum computers, ensuring the long-term security of digital communications. In summary, the future prospects of QST are illuminated by these emerging technologies and trends. As these innovations continue to evolve, they promise to reshape industries, enhance security, and unlock new scientific discoveries. The integration of quantum technologies into various fields will undoubtedly drive significant progress, making QST a cornerstone of future technological advancements.
Potential Breakthroughs and Discoveries
In the realm of Quantum Science and Technology (QST), potential breakthroughs and discoveries are poised to revolutionize various fields, from computing and communication to medicine and materials science. One of the most promising areas is quantum computing, where advancements in quantum algorithms and error correction could lead to exponential increases in computational power. This could solve complex problems that are currently intractable, such as optimizing large-scale systems, simulating molecular interactions for drug discovery, and cracking complex encryption codes. Another significant breakthrough could come from quantum communication, particularly in the development of secure quantum networks. These networks, leveraging quantum entanglement and superposition, would enable virtually unbreakable encryption methods, safeguarding sensitive information against cyber threats. Furthermore, quantum sensing technologies are on the cusp of transforming precision measurement. Quantum sensors, capable of detecting minute changes in magnetic fields, temperature, and other physical parameters, could enhance navigation systems, improve medical imaging techniques like MRI, and even aid in the discovery of new mineral resources. The field of quantum materials science is also ripe for innovation, with researchers exploring new properties of superconductors, superfluids, and topological insulators. These materials hold the potential to create ultra-efficient energy storage devices, high-speed transportation systems, and advanced electronic components. Additionally, quantum-inspired technologies are emerging as a bridge between classical and quantum systems. These innovations, such as quantum annealing and machine learning algorithms inspired by quantum mechanics, can solve optimization problems more efficiently than traditional methods. They are being applied in logistics, finance, and artificial intelligence to optimize processes and make more accurate predictions. The intersection of QST with other disciplines like biology and chemistry is also yielding exciting prospects. Quantum biology, for instance, seeks to understand how quantum phenomena influence biological processes at the molecular level. This could lead to new insights into photosynthesis, enzyme catalysis, and even the origins of life itself. Similarly, quantum chemistry is advancing our understanding of chemical reactions and molecular interactions, which could accelerate the development of new drugs and materials. Overall, the future of QST is marked by a plethora of potential breakthroughs and discoveries that promise to transform industries and improve our daily lives. As researchers continue to push the boundaries of what is possible with quantum science, we can expect significant advancements in computing power, communication security, precision measurement, and material science, among other areas. These innovations will not only solve some of humanity's most pressing challenges but also open up new avenues for scientific exploration and technological innovation.
Challenges and Opportunities Ahead
As we delve into the future prospects and innovations in Quantum Science and Technology (QST), it is imperative to acknowledge both the challenges and opportunities that lie ahead. One of the primary challenges is the inherent complexity of quantum systems, which demands sophisticated control and precision to maintain coherence and avoid decoherence. This complexity necessitates significant advancements in materials science, nanotechnology, and cryogenic engineering to develop robust and scalable quantum hardware. Additionally, the need for highly specialized expertise poses a significant barrier to entry, requiring substantial investment in education and training programs to cultivate a skilled workforce capable of driving innovation in this field. Despite these challenges, QST presents numerous opportunities that could revolutionize various sectors. For instance, quantum computing has the potential to solve complex problems that are currently intractable with classical computers, leading to breakthroughs in fields such as medicine, finance, and climate modeling. Quantum cryptography offers unparalleled security for data transmission, which could transform the way we protect sensitive information. Furthermore, quantum sensing technologies promise enhanced precision in navigation, spectroscopy, and imaging, opening up new avenues for scientific research and industrial applications. The integration of QST with other emerging technologies like artificial intelligence and the Internet of Things (IoT) could also yield synergistic benefits. For example, quantum machine learning algorithms could enhance AI models by providing more accurate predictions and faster processing times. Similarly, quantum-secured communication networks could safeguard IoT devices from cyber threats, ensuring the integrity of data exchanged between interconnected devices. Moreover, the development of QST is not just about technological advancements but also about fostering economic growth and societal impact. Governments and private investors are increasingly recognizing the potential of QST to drive innovation and competitiveness, leading to substantial funding initiatives aimed at accelerating research and development. This investment could lead to the creation of new industries, jobs, and opportunities for economic development. In conclusion, while the path forward for QST is fraught with technical and educational challenges, the potential rewards are substantial. By addressing these challenges through collaborative research efforts, strategic investments, and comprehensive educational programs, we can unlock the full potential of quantum science and technology. As we navigate this complex landscape, it is clear that QST holds the promise of transforming various aspects of our lives and contributing significantly to future scientific and technological advancements.