What Is Turgor Pressure
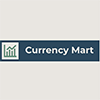
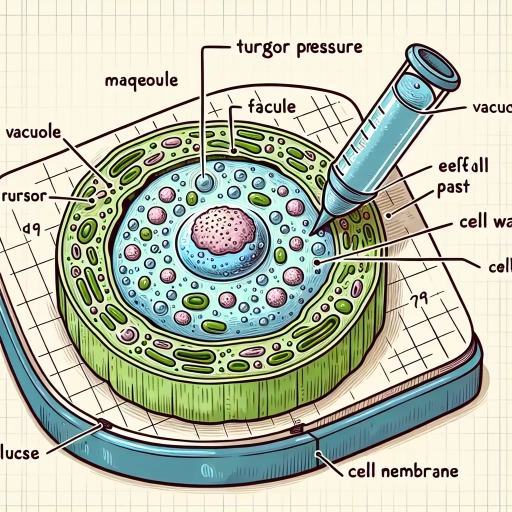
Turgor pressure is a fundamental concept in plant biology that plays a crucial role in the structure, function, and overall health of plants. This pressure, which arises from the movement of water into plant cells, is essential for maintaining the rigidity and shape of plant tissues. In this article, we will delve into the intricacies of turgor pressure, starting with its definition and basic principles. We will explore how turgor pressure works at a mechanistic level, including the processes that drive water uptake and the cellular structures involved. Additionally, we will examine the significant impact of turgor pressure on plant function, from supporting plant growth and development to influencing interactions with the environment. By understanding these aspects, readers will gain a comprehensive insight into the vital role turgor pressure plays in the life of plants. Let us begin by defining and understanding the basics of turgor pressure.
Understanding Turgor Pressure: Definition and Basics
Turgor pressure is a fundamental concept in plant biology, playing a crucial role in the structure, function, and growth of plants. At its core, turgor pressure is the water pressure exerted against the cell wall of a plant cell, which is essential for maintaining the cell's shape and rigidity. This article delves into the intricacies of turgor pressure, starting with a clear definition that clarifies its scientific basis. We will then explore its role in plant cell structure, highlighting how turgor pressure supports the cell wall and contributes to the plant's overall architecture. Finally, we will discuss the importance of turgor pressure in plant growth, revealing how it influences various physiological processes such as cell expansion and plant development. By understanding these aspects, readers will gain a comprehensive insight into the significance of turgor pressure, ultimately leading to a deeper appreciation of its role in plant biology. Understanding Turgor Pressure: Definition and Basics is essential for anyone interested in the fascinating world of plant physiology.
1. Definition of Turgor Pressure
**Understanding Turgor Pressure: Definition and Basics** Turgor pressure is a fundamental concept in plant physiology, playing a crucial role in the structural integrity and function of plant cells. **Definition of Turgor Pressure:** Turgor pressure is the hydrostatic pressure exerted by water within a plant cell against the cell wall, resulting from the osmotic movement of water into the cell. This pressure is generated when water enters the cell through osmosis, driven by the difference in solute concentrations between the cell's cytoplasm and the surrounding environment. As water flows into the cell, it increases the volume of the cell, causing the cell membrane to press against the rigid cell wall. This resistance from the cell wall prevents further expansion and results in turgor pressure. The process begins with the selective permeability of the cell membrane, which allows water to pass through while restricting the movement of solutes. When a plant cell is placed in a hypotonic solution (a solution with lower solute concentration than the cell's cytoplasm), water rushes into the cell to equalize solute concentrations. This influx of water increases the internal pressure until it is balanced by the resistance of the cell wall. In isotonic solutions (where solute concentrations are equal inside and outside the cell), there is no net movement of water, and thus no significant turgor pressure is generated. Conversely, in hypertonic solutions (where the solute concentration is higher outside the cell), water leaves the cell, reducing turgor pressure and potentially leading to cell shrinkage or plasmolysis. Turgor pressure is essential for maintaining the rigidity and shape of plant tissues. It supports the plant's structure, allowing it to stand upright and resist external forces such as wind and gravity. Additionally, turgor pressure plays a critical role in plant growth and development by driving cell expansion during cell division and elongation. For instance, during seed germination, turgor pressure helps to break through the seed coat, facilitating the emergence of the radicle and cotyledon. In summary, turgor pressure is a vital force that arises from the osmotic influx of water into plant cells, pressing against the cell wall and providing structural support. Understanding this concept is crucial for appreciating how plants maintain their form and function in various environmental conditions. By regulating turgor pressure, plants can adapt to changes in their surroundings, ensuring optimal growth and survival. This fundamental principle underscores the intricate mechanisms that govern plant physiology and highlights the dynamic interplay between cellular components and environmental factors.
2. Role in Plant Cell Structure
In the intricate world of plant cell structure, turgor pressure plays a pivotal role, serving as the driving force behind many of the plant's essential functions. At its core, turgor pressure is the hydrostatic pressure exerted by water within the cell against the cell wall. This pressure is crucial for maintaining the rigidity and shape of plant cells, which in turn supports the overall structure and posture of the plant. The cell wall, composed primarily of cellulose, hemicellulose, and pectin, acts as a rigid yet flexible barrier that resists the inward pull of turgor pressure. This resistance allows the cell to maintain its shape and provides mechanical support to the plant, enabling it to stand upright and withstand environmental stresses such as wind and gravity. The vacuole, a large organelle found in plant cells, is key to generating turgor pressure. It stores water and solutes, which are absorbed through osmosis, leading to an increase in water potential inside the cell. As water rushes into the vacuole, it presses against the cell membrane and then against the cell wall, creating turgor pressure. This pressure is vital for various physiological processes in plants. For instance, it facilitates the transport of water and nutrients through the xylem by creating a positive pressure gradient that helps drive the ascent of sap from roots to leaves. Turgor pressure also plays a critical role in stomatal movement; guard cells surrounding stomata adjust their turgor pressure to control gas exchange and transpiration, thereby regulating photosynthesis and water loss. Moreover, turgor pressure is essential for plant growth and development. During cell expansion, turgor pressure helps to stretch the cell wall, allowing the cell to grow in size. This process is tightly regulated by hormonal signals and the synthesis of new cell wall components. In addition, turgor pressure contributes to the mechanical properties of plant tissues, influencing their texture and firmness. In summary, turgor pressure is a fundamental component of plant cell structure and function. It provides structural support, facilitates nutrient transport, regulates stomatal movement, and drives cell growth. Understanding turgor pressure is essential for appreciating how plants maintain their integrity and perform their vital functions in a dynamic environment. This concept underscores the intricate interplay between cellular components and the external environment, highlighting the remarkable adaptability and resilience of plants.
3. Importance in Plant Growth
Turgor pressure plays a pivotal role in plant growth, serving as a fundamental mechanism that supports the structural integrity and physiological functions of plants. At its core, turgor pressure is the hydrostatic pressure exerted by water within plant cells, particularly in the vacuoles. This pressure is crucial for maintaining the rigidity of plant tissues, allowing plants to stand upright and resist external forces such as wind and gravity. Without sufficient turgor pressure, plants would wilt and lose their structural support, hindering their ability to photosynthesize efficiently. Moreover, turgor pressure is essential for cell expansion during plant growth. As cells grow, they must increase in size while maintaining their shape. Turgor pressure provides the necessary force to drive cell wall expansion by pushing against the cell wall from the inside. This process is tightly regulated by the balance of water uptake and loss, ensuring that cells expand uniformly and healthily. In addition, turgor pressure influences stomatal movement, which is critical for gas exchange and transpiration. Stomata, the small pores on leaf surfaces, open and close based on changes in turgor pressure within guard cells surrounding them. This regulation allows plants to optimize their water use and carbon dioxide uptake, directly impacting photosynthesis and overall plant productivity. Furthermore, turgor pressure has a significant impact on plant responses to environmental stresses. For instance, during drought conditions, plants often experience reduced turgor pressure due to water scarcity. This reduction triggers a series of physiological responses aimed at conserving water, such as closing stomata to minimize transpiration and producing stress hormones like abscisic acid. Conversely, under optimal water conditions, high turgor pressure can enhance nutrient uptake from the soil by increasing root turgor, which helps in absorbing essential minerals and nutrients necessary for growth. In summary, turgor pressure is indispensable for plant growth as it provides structural support, drives cell expansion, regulates stomatal movement, and mediates responses to environmental stresses. Understanding turgor pressure is therefore vital for appreciating the intricate mechanisms that govern plant physiology and for developing strategies to enhance plant health and productivity in various agricultural and horticultural contexts. By recognizing the importance of turgor pressure in these processes, researchers and practitioners can better manage plant growth conditions to optimize yield and resilience in diverse environments.
The Mechanism of Turgor Pressure: How It Works
Turgor pressure is a fundamental concept in plant physiology, playing a crucial role in maintaining the structural integrity and function of plant cells. This pressure, generated by the movement of water into plant cells, is essential for plant growth, support, and overall health. To fully comprehend the mechanism of turgor pressure, it is necessary to delve into three key components: water uptake and osmosis, the interaction between cell walls and membranes, and the regulation by stomata and hormones. Water uptake and osmosis explain how water enters plant cells, creating the initial pressure that keeps them turgid. The cell wall and membrane interaction highlights the structural elements that contain this pressure, ensuring the cell maintains its shape without bursting. Finally, the regulation by stomata and hormones reveals how plants dynamically adjust turgor pressure in response to environmental conditions. Understanding these mechanisms is vital for grasping the intricate processes that govern plant life. By exploring these aspects in detail, we can gain a deeper insight into the complex interplay that sustains turgor pressure, ultimately leading to a comprehensive understanding of this critical physiological process. Transitioning from here, we will delve into the basics of turgor pressure, defining what it is and how it operates within the plant cell. **Understanding Turgor Pressure: Definition and Basics**.
1. Water Uptake and Osmosis
**Water Uptake and Osmosis** Water uptake and osmosis are fundamental processes that underpin the mechanism of turgor pressure in plants. Turgor pressure, the force that keeps plant cells rigid and upright, is primarily driven by the movement of water into the cell through a process known as osmosis. Osmosis is the passive transport of water molecules from an area of high concentration to an area of low concentration across a semipermeable membrane, which in plant cells is the cell membrane. This movement is crucial because it allows cells to maintain their structural integrity and support the plant's overall architecture. In plant cells, water uptake occurs when the cell's cytoplasm has a higher solute concentration than the surrounding environment. This creates an osmotic gradient where water molecules naturally flow into the cell to dilute the solutes. The cell wall, which is rigid but permeable, provides structural support while allowing water to pass through. As water enters the cell, it increases the hydrostatic pressure inside the cell, pushing against the cell wall. This pressure is what we refer to as turgor pressure. The process of osmosis is tightly regulated by the cell's ability to control solute concentrations. For instance, plants can adjust their solute levels by synthesizing or breaking down sugars, salts, and other substances. This regulation ensures that the osmotic balance is maintained, preventing excessive water uptake that could lead to cell bursting (lysis) or insufficient uptake that would cause the cell to shrink (plasmolysis). Additionally, specialized structures such as root hairs and stomata play critical roles in facilitating water uptake. Root hairs increase the surface area for absorption from the soil, while stomata on leaves regulate transpiration—the process by which water is lost through evaporation—ensuring that the plant maintains an optimal balance of water intake and loss. In summary, water uptake and osmosis are essential components of turgor pressure in plants. By controlling solute concentrations and facilitating the movement of water across cell membranes, plants are able to maintain the necessary turgor pressure to support their growth and structural integrity. This intricate balance underscores the remarkable adaptability and resilience of plant life, enabling them to thrive in a wide range of environments. Understanding these mechanisms provides valuable insights into how plants function at a cellular level and highlights the importance of water in sustaining life on Earth.
2. Cell Wall and Membrane Interaction
In the intricate mechanism of turgor pressure, the interaction between the cell wall and the cell membrane plays a crucial role. Turgor pressure is the force exerted by water against the cell wall, which is essential for maintaining the structural integrity and rigidity of plant cells. This interaction is fundamentally based on the unique properties of both the cell wall and the cell membrane. The cell wall, primarily composed of cellulose, hemicellulose, and pectin, acts as a rigid yet flexible scaffold that provides mechanical support to the cell. It is this rigidity that allows the cell to withstand the internal pressure generated by water uptake. On the other hand, the cell membrane, a semi-permeable lipid bilayer, regulates the movement of water and solutes in and out of the cell through osmosis. When water enters the cell due to osmotic forces, it increases the internal pressure against the cell wall. The interaction between these two structures is dynamic and highly coordinated. As water enters the cell, it fills the vacuole, causing it to expand. This expansion increases turgor pressure, which is then resisted by the cell wall. The cell wall's elasticity allows it to stretch slightly under this pressure but not to the point of rupture. This balance ensures that the cell maintains its shape and turgidity, which is vital for plant growth and function. Moreover, this interaction is not just passive; it involves active cellular processes. For instance, plants can adjust their turgor pressure by regulating ion transport across the cell membrane. Potassium ions (K+) play a significant role in this process. When K+ ions are pumped into the cell, they increase the osmotic potential, drawing more water into the cell and thus increasing turgor pressure. Conversely, when K+ ions are pumped out, water leaves the cell, reducing turgor pressure. This delicate balance between the cell wall's mechanical strength and the cell membrane's osmotic regulation is what allows plants to maintain their structural integrity and respond to environmental changes. For example, during drought conditions, plants can reduce turgor pressure by losing water, which helps them conserve water and survive until better conditions return. In summary, the interaction between the cell wall and the cell membrane is central to the mechanism of turgor pressure. This interaction ensures that plant cells can maintain their shape, support their structure, and adapt to changing environmental conditions, all of which are essential for plant survival and growth. Understanding this complex relationship provides valuable insights into how plants function at a cellular level and highlights the remarkable adaptability of plant physiology.
3. Regulation by Stomata and Hormones
Regulation by stomata and hormones is a critical component in the mechanism of turgor pressure, ensuring that plants maintain optimal water balance and structural integrity. Stomata, small pores found on the surfaces of leaves, play a pivotal role in this process. These stomata are controlled by guard cells, which swell or shrink based on the plant's water status. When the plant is well-hydrated, guard cells absorb water and swell, causing the stomata to open and allowing for gas exchange—primarily carbon dioxide uptake and oxygen release. Conversely, during drought or high transpiration rates, guard cells lose water and shrink, closing the stomata to conserve water. This dynamic regulation helps maintain turgor pressure within the plant cells by controlling water loss through transpiration. Hormones also play a significant role in regulating turgor pressure. Abscisic acid (ABA) is a key hormone involved in this process. During drought conditions, ABA levels increase, triggering a signaling cascade that leads to the closure of stomata. This hormone acts on guard cells to reduce their turgor pressure, thereby preventing excessive water loss. On the other hand, hormones like auxins and cytokinins can influence cell growth and division, indirectly affecting turgor pressure by altering cell wall properties and water uptake capabilities. For instance, auxins promote cell elongation by increasing cell wall extensibility, which can enhance water uptake and turgor pressure in growing tissues. The interplay between stomatal regulation and hormonal signaling ensures that plants can adapt to varying environmental conditions while maintaining structural integrity. For example, during the day when light is abundant and photosynthesis is active, stomata open to allow CO2 uptake, but as light intensity decreases or water becomes scarce, ABA signals the closure of stomata to conserve water. This balanced approach allows plants to optimize their growth and survival under diverse conditions. In summary, the regulation of turgor pressure by stomata and hormones is a sophisticated mechanism that enables plants to manage their water status efficiently. By controlling stomatal aperture and influencing cell growth, these regulatory systems help plants maintain optimal turgor pressure, ensuring proper functioning of physiological processes such as photosynthesis and nutrient transport. This intricate balance underscores the remarkable adaptability of plants to their environment, highlighting the complex interplay between structural, physiological, and hormonal factors that sustain plant life.
Impact of Turgor Pressure on Plant Function and Environment
Turgor pressure, the internal pressure exerted by water within plant cells, plays a pivotal role in the functioning and survival of plants. This critical physiological process influences various aspects of plant biology, from the mechanical movements of leaves to the intricate balance of water relations and the plant's ability to adapt to environmental conditions. In this article, we will delve into the multifaceted impact of turgor pressure on plant function and its interaction with the environment. We will explore how turgor pressure affects leaf movement and photosynthesis, enabling plants to optimize their exposure to sunlight and CO2. Additionally, we will examine its influence on plant water relations and drought tolerance, highlighting the mechanisms that allow plants to thrive under water-stressed conditions. Finally, we will discuss how turgor pressure interacts with environmental factors such as temperature and light, illustrating its dynamic role in plant-environment interactions. By understanding these complex relationships, we can gain a deeper appreciation for the essential role turgor pressure plays in plant biology, which will be further elucidated by transitioning to a detailed explanation of **Understanding Turgor Pressure: Definition and Basics**.
1. Effects on Leaf Movement and Photosynthesis
Turgor pressure, the internal fluid pressure within plant cells, plays a crucial role in various aspects of plant function, particularly in leaf movement and photosynthesis. This pressure is essential for maintaining the structural integrity of leaves and facilitating their movements in response to environmental cues. For instance, in plants like the sensitive plant (Mimosa pudica), turgor pressure changes allow the leaves to fold up when touched or disturbed, a phenomenon known as thigmonasty. This adaptive response helps protect the plant from potential herbivores and mechanical damage. Similarly, in plants such as the prayer plant (Maranta leuconeura), turgor pressure adjustments enable the leaves to move towards or away from light sources, optimizing their exposure for photosynthesis. Photosynthesis itself is also influenced by turgor pressure. The process requires an adequate supply of water and nutrients, which are transported through the xylem and phloem under the influence of turgor pressure. When turgor pressure is optimal, stomata (small pores on the leaf surface) can open and close efficiently, regulating gas exchange and water loss. This precise control allows plants to maximize carbon dioxide uptake while minimizing water loss, thereby optimizing photosynthetic efficiency. Conversely, under conditions of drought or water stress, reduced turgor pressure can lead to stomatal closure, limiting CO2 availability and thus impairing photosynthesis. Moreover, turgor pressure affects the overall shape and orientation of leaves, which in turn impacts light interception and distribution within the leaf canopy. Leaves with optimal turgor pressure maintain their natural curvature and positioning, ensuring that they capture sunlight effectively. This is particularly important in dense plant communities where competition for light is intense. In addition, the rigidity provided by turgor pressure helps leaves withstand wind and other mechanical stresses without significant deformation or damage. In summary, turgor pressure is a vital component of plant physiology that directly influences leaf movement and photosynthetic processes. By regulating cell volume and maintaining structural integrity, turgor pressure enables plants to adapt to their environment dynamically while optimizing their ability to capture light and perform photosynthesis efficiently. This intricate balance underscores the critical role of turgor pressure in supporting the overall health and productivity of plants.
2. Influence on Plant Water Relations and Drought Tolerance
Turgor pressure, the internal water pressure within plant cells, plays a pivotal role in influencing plant water relations and enhancing drought tolerance. This pressure is crucial for maintaining cell shape and rigidity, which in turn supports the overall structure of the plant. When turgor pressure is optimal, plants can efficiently transport water and nutrients through the xylem, ensuring proper hydration and nutrient distribution. However, under drought conditions, turgor pressure can significantly decline due to reduced water availability. This decrease can lead to wilting and impaired photosynthesis, as stomata close to conserve water, thereby reducing gas exchange and limiting CO2 uptake. Despite these challenges, some plants have evolved mechanisms to maintain or recover turgor pressure during drought. For instance, certain species can produce osmolytes—compounds that help retain water within cells by lowering the osmotic potential. This allows plants to maintain turgor pressure even when soil water is scarce. Additionally, plants may adjust their root architecture to explore deeper soil layers for water or develop more efficient stomatal control to minimize water loss. These adaptations not only help plants survive during drought but also enable them to recover quickly once water becomes available again. The influence of turgor pressure on plant water relations is also evident in the regulation of stomatal aperture. Stomata, the small pores on leaf surfaces responsible for gas exchange, are highly sensitive to changes in turgor pressure. When turgor pressure drops due to drought, stomata close to prevent further water loss, a response mediated by abscisic acid (ABA), a hormone that plays a central role in drought stress signaling. This closure reduces transpiration but also limits CO2 uptake, highlighting the delicate balance between water conservation and photosynthetic activity. In summary, turgor pressure is a critical factor in plant water relations and drought tolerance. Its maintenance or recovery during drought is essential for sustaining plant function and ensuring survival. Through various physiological and morphological adaptations, plants can mitigate the negative impacts of reduced turgor pressure, thereby enhancing their resilience to water stress. Understanding these mechanisms provides valuable insights into how plants cope with environmental challenges and underscores the importance of turgor pressure in plant-environment interactions.
3. Interaction with Environmental Factors like Temperature and Light
Interaction with environmental factors such as temperature and light plays a crucial role in the dynamics of turgor pressure within plants. Turgor pressure, the internal pressure exerted by water against the cell walls of plant cells, is fundamentally influenced by these external conditions. Temperature affects the rate of metabolic processes, including photosynthesis and respiration, which in turn impact the balance of water and solutes within plant cells. At optimal temperatures, plants can efficiently regulate their water uptake and loss, maintaining a stable turgor pressure that supports cell growth and structural integrity. However, extreme temperatures can disrupt this balance: high temperatures may lead to increased transpiration rates, causing water loss and reduced turgor pressure, while low temperatures can slow down metabolic activities, potentially leading to water accumulation and increased turgor pressure. Light is another critical environmental factor that influences turgor pressure. Photosynthesis, which occurs in the presence of light, drives the production of sugars and other organic compounds that contribute to osmotic potential within plant cells. During daylight hours, increased photosynthetic activity enhances the uptake of carbon dioxide and release of oxygen, which indirectly supports the maintenance of turgor pressure by regulating solute concentrations. Additionally, light exposure triggers stomatal opening, allowing for gas exchange and influencing transpiration rates. This interplay between light-induced photosynthesis and stomatal regulation ensures that plants can adapt their turgor pressure to optimize growth and survival under varying light conditions. The interaction between temperature and light also has synergistic effects on turgor pressure. For instance, high light intensities combined with optimal temperatures can maximize photosynthetic rates, enhancing solute production and maintaining robust turgor pressure. Conversely, low light conditions coupled with extreme temperatures can severely impair photosynthesis and disrupt water relations, leading to fluctuations in turgor pressure that may compromise plant function. Understanding these interactions is essential for appreciating how environmental factors modulate turgor pressure and, by extension, impact overall plant health and productivity. By recognizing these dynamics, researchers and agricultural practitioners can develop strategies to optimize plant growth under diverse environmental conditions, ultimately enhancing crop resilience and yield.