What Is Conventional Current
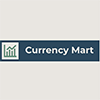
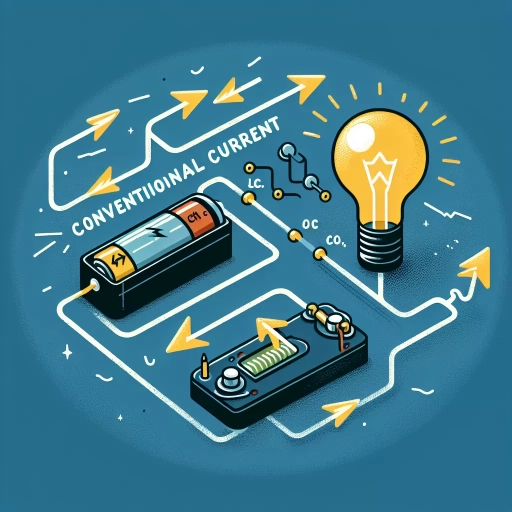
Conventional current is a fundamental concept in the study of electricity, often misunderstood but crucial for understanding how electrical systems operate. This article delves into the essence of conventional current, exploring its definition, practical applications, and the significant implications it has on our daily lives. First, we will delve into **Understanding Conventional Current**, clarifying the historical context and scientific principles behind this concept. Next, we will examine **Applications of Conventional Current**, highlighting how it is utilized in various technologies and industries. Finally, we will discuss **Importance and Implications of Conventional Current**, revealing its impact on safety standards, engineering practices, and the broader societal benefits. By grasping these aspects, readers will gain a comprehensive understanding of conventional current and its pivotal role in modern technology. Let us begin by **Understanding Conventional Current**.
Understanding Conventional Current
Understanding conventional current is a fundamental concept in the study of electricity, and it is crucial to grasp its historical context, definition, and basic principles, as well as how it compares to electron flow. Historically, the concept of conventional current was established before the discovery of electrons, leading to a model where current was thought to flow from positive to negative. This historical context is essential for appreciating why conventional current remains a standard in electrical engineering and physics. The definition and basic principles of conventional current explain how it is used to describe the flow of electric charge in circuits, providing a consistent framework for analyzing and designing electrical systems. Finally, comparing conventional current with electron flow highlights the difference between the theoretical direction of electron movement and the practical, standardized direction used in engineering applications. By exploring these aspects, one can gain a comprehensive understanding of conventional current and its significance in modern electrical science. Let's begin by delving into the historical context of conventional current.
Historical Context of Conventional Current
The historical context of conventional current is deeply intertwined with the early understanding and study of electricity. The concept of conventional current, which defines the direction of electric current flow from positive to negative, was established during a period when the nature of electricity was still being explored. In the 18th and 19th centuries, scientists such as Benjamin Franklin and Alessandro Volta contributed significantly to the field. Franklin proposed that electricity was a form of fluid that flowed through conductors, and he arbitrarily assigned positive and negative charges to this fluid. This early model, though incorrect by modern standards, laid the groundwork for later researchers. One pivotal figure in this narrative is Michael Faraday, who in the 1830s conducted extensive experiments on electricity and formulated the laws of electrolysis. His work helped solidify the idea that electric current was a flow of charge, but he did not specify the direction. It was later scientists like André-Marie Ampère and Georg Ohm who built upon Faraday's findings and developed more precise theories about electric current. The term "conventional current" itself was coined because it followed the direction of positive charge flow as initially proposed by Franklin. However, it was later discovered by J.J. Thomson in 1897 that electric current is actually carried by negatively charged electrons moving in the opposite direction. Despite this discovery, the convention established by Franklin and reinforced by subsequent researchers remained in place due to its widespread adoption and practical utility. This historical context highlights how scientific understanding evolves over time. The persistence of conventional current as a standard in electrical engineering and physics is a testament to the practicality and consistency it brings to the field, even though it does not align with the actual physical movement of charge carriers. This dichotomy between historical convention and modern scientific understanding underscores the importance of historical context in comprehending why certain principles remain integral to our current knowledge of electricity.
Definition and Basic Principles
**Understanding Conventional Current** ### Definition and Basic Principles Conventional current, a fundamental concept in electrical engineering and physics, refers to the flow of electric charge in a circuit. It is defined as the movement of positive charge carriers, such as protons or holes, from a region of higher potential to a region of lower potential. This concept was established by Benjamin Franklin in the 18th century, who arbitrarily assigned positive and negative charges to the two types of electric charges he observed. Despite later discoveries revealing that electrons (negatively charged particles) are the actual charge carriers in most conductors, the convention has been retained for consistency and simplicity. The basic principles of conventional current are rooted in the behavior of electric fields and potentials. In any circuit, conventional current flows from the positive terminal of a power source (such as a battery) through the circuit and back to the negative terminal. This flow is driven by the electric field generated by the potential difference between the terminals. The direction of conventional current is always from higher potential to lower potential, which aligns with the direction of the electric field lines. Key principles include Ohm's Law, which states that current (I) is equal to voltage (V) divided by resistance (R): \( I = \frac{V}{R} \). This law underscores that current is directly proportional to voltage and inversely proportional to resistance. Additionally, Kirchhoff's Laws provide further insights into how currents and voltages behave in complex circuits. The first law states that the sum of currents entering a node is equal to the sum of currents leaving it, while the second law states that the sum of voltage changes around any closed loop is zero. Understanding conventional current is crucial for designing and analyzing electrical circuits, predicting their behavior under various conditions, and ensuring safety and efficiency. It forms the basis for more advanced concepts such as AC and DC circuits, circuit analysis techniques, and electronic device operation. By adhering to this convention, engineers and physicists can communicate effectively and work with a unified framework that simplifies complex electrical phenomena. In summary, conventional current is a well-established concept that describes the flow of positive charge carriers in an electric circuit from higher to lower potential. Its principles are grounded in fundamental laws like Ohm's Law and Kirchhoff's Laws, making it an indispensable tool for understanding and working with electrical systems.
Comparison with Electron Flow
When understanding conventional current, it is crucial to compare it with electron flow to grasp the fundamental principles of electrical current. Conventional current, also known as traditional current, is defined as the flow of positive charge carriers, such as protons or holes in a semiconductor, from the positive terminal to the negative terminal of a circuit. This concept was established before the discovery of electrons and has been retained for consistency and simplicity in circuit analysis. In contrast, electron flow refers to the actual movement of electrons within a conductor. Electrons are negatively charged particles that move from the negative terminal to the positive terminal when a voltage is applied across the circuit. This movement is driven by the electric field created by the potential difference between the terminals. The key difference between conventional current and electron flow lies in their direction. Conventional current flows in the opposite direction to electron flow due to the historical convention of considering positive charge carriers. This distinction is important because it affects how we analyze and describe electrical circuits. For instance, in a simple circuit with a battery and a resistor, conventional current flows from the positive terminal of the battery through the resistor and back to the negative terminal, while electrons actually move in the opposite direction. Despite this difference in direction, both concepts are essential for understanding electrical phenomena. Conventional current simplifies circuit analysis by allowing engineers to use familiar mathematical tools and circuit laws like Ohm's Law and Kirchhoff's Laws without needing to consider the detailed behavior of individual electrons. On the other hand, understanding electron flow provides insight into the physical mechanisms underlying electrical conduction, which is vital for advanced topics such as semiconductor physics and quantum mechanics. In practical applications, engineers often use conventional current for designing and analyzing circuits because it aligns with established notation and conventions in electrical engineering. However, recognizing that electrons are the actual charge carriers helps in understanding phenomena like resistance, where collisions between electrons and atoms within conductors result in energy loss. In summary, while conventional current and electron flow describe different aspects of electrical current, both are indispensable for a comprehensive understanding of electricity. Conventional current offers a simplified framework for circuit analysis, while electron flow reveals the underlying physical processes that govern electrical behavior. By comparing these two concepts, one can appreciate the dual nature of electrical current and apply this knowledge effectively in various fields of electrical engineering.
Applications of Conventional Current
The concept of conventional current, often misunderstood as the flow of electrons, is actually the flow of positive charge carriers. This fundamental principle has far-reaching applications across various fields, particularly in electrical engineering. In the realm of electrical engineering and circuit analysis, understanding conventional current is crucial for designing and analyzing complex circuits. It also plays a pivotal role in the development and operation of electronic devices and systems, where precise control over current flow is essential. Additionally, conventional current is vital in power distribution and transmission systems, ensuring efficient and safe delivery of electrical energy over long distances. By grasping the principles of conventional current, engineers can optimize circuit performance, enhance device functionality, and ensure reliable power supply. This article will delve into these applications, starting with the foundational aspects of electrical engineering and circuit analysis.
Electrical Engineering and Circuit Analysis
Electrical Engineering and Circuit Analysis are foundational disciplines that underpin the understanding and application of conventional current. Electrical engineering, a field that deals with the study and application of electricity, electronics, and electromagnetism, relies heavily on circuit analysis to design, build, and maintain electrical systems. Circuit analysis involves the mathematical and theoretical methods used to determine the behavior of electrical circuits, which are crucial for ensuring that these systems operate safely and efficiently. In the context of conventional current, circuit analysis plays a pivotal role in understanding how current flows through a circuit. Conventional current is defined as the flow of positive charge, which is opposite to the actual flow of electrons but simplifies many calculations and analyses. By using conventional current, engineers can predict voltage drops, current flows, and power consumption in complex circuits. This is achieved through various techniques such as Kirchhoff's laws, Thevenin's theorem, and Norton's theorem, which provide systematic approaches to solving circuit problems. The applications of conventional current in circuit analysis are diverse and widespread. For instance, in power systems engineering, conventional current is used to analyze transmission lines and distribution networks to ensure reliable and efficient power delivery. In electronic design, it helps engineers to develop and troubleshoot electronic circuits, from simple amplifiers to complex digital systems. Additionally, in control systems engineering, understanding conventional current flow is essential for designing feedback loops and control mechanisms that stabilize and optimize system performance. Moreover, the use of conventional current simplifies many educational and practical aspects of electrical engineering. It allows students and practitioners to focus on the fundamental principles without getting bogged down by the complexities of electron flow. This simplification enables quicker problem-solving and better visualization of circuit behavior, making it an indispensable tool in both academic curricula and professional practice. In summary, electrical engineering and circuit analysis are intricately linked with the concept of conventional current. By leveraging conventional current, engineers can perform detailed analyses of electrical circuits, ensuring that systems are designed to meet specific requirements and operate within safe limits. The widespread adoption of conventional current in various fields underscores its importance as a fundamental concept in electrical engineering education and practice.
Electronic Devices and Systems
Electronic devices and systems are integral components of modern life, leveraging the principles of conventional current to function efficiently. Conventional current, which flows from positive to negative, is a fundamental concept in understanding how these devices operate. In electronic devices such as smartphones, laptops, and televisions, conventional current plays a crucial role in powering the circuits that enable communication, computation, and entertainment. For instance, in a smartphone, conventional current flows through the battery to the processor, memory, and display, allowing users to make calls, send messages, and access the internet. Similarly, in laptops, conventional current powers the CPU, RAM, and storage devices, facilitating tasks like word processing and data analysis. In electronic systems like home appliances and automotive electronics, conventional current ensures reliable operation. For example, in a refrigerator, conventional current powers the compressor motor and control circuits that maintain optimal temperature settings. In vehicles, electronic systems such as anti-lock braking systems (ABS) and traction control systems (TCS) rely on conventional current to enhance safety and performance. These systems use sensors and actuators that are controlled by electronic control units (ECUs), which process data and make decisions based on the flow of conventional current. Moreover, industrial automation and control systems heavily depend on conventional current. Programmable logic controllers (PLCs) and distributed control systems (DCS) use conventional current to monitor and control industrial processes such as manufacturing lines and power plants. These systems ensure precise control over machinery and processes, optimizing efficiency and safety. In medical devices like ECG machines and MRI scanners, conventional current is essential for accurate diagnostics. These devices use sophisticated electronic circuits that rely on the flow of conventional current to capture and analyze physiological signals or generate high-resolution images. Furthermore, renewable energy systems such as solar panels and wind turbines convert natural energy into electrical energy using conventional current principles. Inverters in these systems convert DC power generated by solar panels or wind turbines into AC power that can be fed into the grid or used locally. In summary, electronic devices and systems across various sectors—consumer electronics, automotive, industrial automation, medical diagnostics, and renewable energy—rely on the concept of conventional current to operate effectively. Understanding conventional current is pivotal for designing, maintaining, and optimizing these systems to meet the demands of modern technology.
Power Distribution and Transmission
Power distribution and transmission are critical components of the electrical grid, ensuring that electricity generated at power plants is efficiently delivered to consumers. The process begins with power generation, where electrical energy is produced through various methods such as thermal, nuclear, or renewable sources. This generated power is then transmitted over long distances via high-voltage transmission lines, which are designed to minimize energy loss due to resistance. The high voltage is necessary to reduce the current flowing through the lines, thereby reducing heat loss and increasing efficiency. Once the electricity reaches a substation near the consumption area, it is transformed to lower voltages suitable for distribution. Distribution lines, often seen as overhead power lines or underground cables, carry this lower-voltage electricity to residential, commercial, and industrial areas. The distribution network includes transformers that step down the voltage further to levels safe for household use. The efficiency of power distribution and transmission systems is enhanced by advanced technologies such as smart grids, which integrate information and communication technologies to optimize energy distribution. Smart grids enable real-time monitoring and control of the grid, allowing for better management of energy supply and demand, reducing power outages, and improving overall reliability. In addition, advancements in materials science have led to the development of more efficient transmission lines and substations. For instance, high-temperature superconductors can significantly reduce energy losses during transmission. Furthermore, renewable energy sources like solar and wind power are increasingly being integrated into the grid, necessitating flexible and adaptive distribution systems. The applications of conventional current in power distribution and transmission are multifaceted. Conventional current, which flows from positive to negative, is the standard for electrical engineering and design. It simplifies the understanding and analysis of electrical circuits, making it easier to design and maintain complex power distribution networks. The use of conventional current ensures consistency across different components of the electrical grid, facilitating easier troubleshooting and maintenance. Moreover, conventional current is essential for the operation of protective devices such as circuit breakers and fuses. These devices rely on the predictable flow of current to interrupt power supply in case of faults or overloads, thereby preventing damage to equipment and ensuring safety. The widespread adoption of conventional current also facilitates international standards and interoperability among different electrical systems, making it easier to integrate new technologies into existing grids. In summary, power distribution and transmission are intricate processes that rely heavily on the principles of conventional current. By understanding and applying these principles, engineers can design more efficient, reliable, and safe electrical grids that meet the increasing demands of modern society. The integration of advanced technologies and adherence to conventional current standards are crucial for maintaining the integrity and performance of the electrical grid.
Importance and Implications of Conventional Current
The concept of conventional current is a cornerstone in the field of electrical engineering, playing a pivotal role in the functioning and development of modern electrical systems. Understanding its importance and implications is crucial for several reasons. First, standardization in electrical systems ensures that all components and devices operate harmoniously, reducing the risk of malfunctions and enhancing overall system reliability. Second, safety and efficiency considerations are paramount; adhering to conventional current standards helps prevent electrical hazards and optimizes energy transmission. Lastly, the impact on technological advancements cannot be overstated, as consistent current flow enables the development of more sophisticated and efficient technologies. By exploring these aspects, we can appreciate the significance of conventional current in maintaining uniformity and driving innovation. Let us begin by examining how standardization in electrical systems has been instrumental in shaping the modern electrical landscape.
Standardization in Electrical Systems
Standardization in electrical systems is a cornerstone of modern electrical engineering, ensuring safety, efficiency, and interoperability across various devices and systems. This standardization is particularly crucial when considering the concept of conventional current, which defines the direction of electric current flow as from positive to negative. The importance of standardization becomes evident in several key areas. Firstly, **safety** is significantly enhanced through standardized practices. Uniform standards for wiring, circuit design, and component specifications reduce the risk of electrical shocks, fires, and other hazards. For instance, the use of standardized color codes for wires (such as red for positive and black for negative) helps technicians and engineers quickly identify potential dangers and avoid mistakes. Secondly, **interoperability** is greatly improved with standardized electrical systems. Components from different manufacturers can be seamlessly integrated into a single system without compatibility issues. This interoperability facilitates the development of complex electrical networks and ensures that devices from various sources can work together efficiently. For example, standardized connectors and sockets enable the easy connection of appliances to power sources regardless of their origin. Thirdly, **efficiency** is optimized through standardized practices. Standardized components and systems allow for easier maintenance and troubleshooting. Technicians can rely on familiar configurations and protocols, reducing the time and effort required to diagnose and repair issues. Additionally, standardized systems enable better resource allocation and planning, as engineers can predict performance and behavior based on established norms. Fourthly, **economical benefits** are substantial. Standardization reduces production costs by allowing mass production of components that meet specific standards. This economies-of-scale approach makes electrical devices more affordable for consumers. Furthermore, standardized systems reduce the need for custom solutions, which are often more expensive and time-consuming to develop. Lastly, **regulatory compliance** is simplified with standardized electrical systems. Governments and regulatory bodies can enforce uniform standards, ensuring that all electrical installations meet minimum safety and performance criteria. This compliance helps in preventing electrical accidents and ensures that all electrical work is done to a high standard. In summary, standardization in electrical systems is vital for ensuring safety, interoperability, efficiency, economic benefits, and regulatory compliance. These aspects are particularly relevant when discussing conventional current, as they underscore the importance of a unified approach to understanding and working with electric current. By adhering to standardized practices, we can build more reliable, efficient, and safe electrical systems that support our daily lives and technological advancements.
Safety and Efficiency Considerations
When discussing the importance and implications of conventional current, it is crucial to delve into safety and efficiency considerations. Conventional current, which flows from positive to negative, is a fundamental concept in electrical engineering and has significant implications for both safety and efficiency in various applications. **Safety Considerations:** 1. **Proper Wiring:** Understanding conventional current helps in ensuring proper wiring practices. Incorrect wiring can lead to short circuits, electrical shocks, or even fires. By adhering to conventional current flow, electricians can avoid such hazards. 2. **Device Protection:** Many electrical devices are designed with conventional current flow in mind. Misunderstanding this concept can result in device malfunction or damage, which may pose safety risks. 3. **Grounding:** Proper grounding techniques rely on the understanding of conventional current flow. Incorrect grounding can lead to unsafe conditions where electrical shocks are more likely. **Efficiency Considerations:** 1. **Optimal Circuit Design:** Knowing how conventional current flows allows engineers to design circuits that are more efficient. This includes minimizing resistance and maximizing power transfer. 2. **Energy Loss Reduction:** Efficient circuit design based on conventional current principles helps reduce energy losses due to heat dissipation and other factors. 3. **Component Selection:** Choosing components that are compatible with conventional current flow ensures optimal performance and longevity of electrical systems. **Practical Applications:** 1. **Power Distribution Systems:** In power distribution systems, understanding conventional current is essential for designing safe and efficient networks that supply electricity to homes and industries. 2. **Electronic Devices:** Electronic devices such as computers, smartphones, and televisions rely on efficient circuit designs based on conventional current principles to function optimally. 3. **Industrial Automation:** Industrial automation systems, which include control circuits and motor drives, depend heavily on accurate understanding and application of conventional current for safe and efficient operation. In summary, recognizing the flow of conventional current is pivotal for ensuring both safety and efficiency in electrical systems. It guides proper wiring practices, device protection, and grounding techniques while enabling the design of optimal circuits that minimize energy losses and maximize performance. These considerations are fundamental across various applications from residential power distribution to complex industrial automation systems. By adhering to these principles, engineers and technicians can create safer, more reliable, and more efficient electrical systems.
Impact on Technological Advancements
The impact of technological advancements on conventional current is multifaceted and profound, driving significant changes in how we understand, utilize, and manage electrical currents. At the heart of these advancements lies the evolution of materials science, which has led to the development of more efficient conductors and insulators. For instance, the introduction of superconducting materials has enabled the creation of high-performance electrical systems that can carry current with minimal resistance, thereby reducing energy losses and enhancing overall system efficiency. This technological leap has far-reaching implications for power transmission and distribution, allowing for more reliable and cost-effective delivery of electricity over long distances. Moreover, advancements in semiconductor technology have revolutionized the field of electronics by enabling the development of sophisticated control systems and power electronics. These advancements allow for precise control over electrical currents, facilitating the widespread adoption of technologies such as variable frequency drives and power factor correction devices. These technologies not only improve the efficiency of electrical systems but also enhance their reliability and longevity. The integration of digital technologies, particularly in the realm of smart grids and IoT (Internet of Things), has transformed the way conventional current is monitored, managed, and optimized. Real-time data analytics and machine learning algorithms enable utilities to predict energy demand more accurately, detect anomalies in the grid, and implement predictive maintenance strategies. This level of intelligence in electrical systems ensures better resource allocation, reduces the likelihood of power outages, and supports the integration of renewable energy sources into the grid. Furthermore, advancements in battery technology have made it possible to store electrical energy more efficiently, which is crucial for stabilizing the grid when there are fluctuations in supply and demand. Advanced battery systems can absorb excess energy during periods of low demand and release it during peak hours, thereby smoothing out the flow of conventional current and ensuring a stable power supply. In addition to these technical improvements, technological advancements have also led to significant safety enhancements. Modern circuit breakers and protective relays are equipped with advanced sensors and algorithms that can detect faults in real-time, preventing electrical accidents and reducing downtime. These safety features are particularly important in industrial settings where high currents are involved. Lastly, the environmental impact of these technological advancements should not be overlooked. By improving the efficiency of electrical systems and enabling better integration of renewable energy sources, we can reduce our reliance on fossil fuels and lower greenhouse gas emissions. This not only contributes to a more sustainable future but also aligns with global efforts to mitigate climate change. In summary, technological advancements have profoundly impacted the understanding, application, and management of conventional current. From more efficient materials and sophisticated control systems to smart grid technologies and advanced safety features, these innovations have transformed the electrical industry, enhancing efficiency, reliability, and sustainability. As technology continues to evolve, we can expect even more significant improvements in how we harness and utilize electrical energy.