What Is Melting
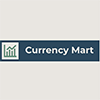
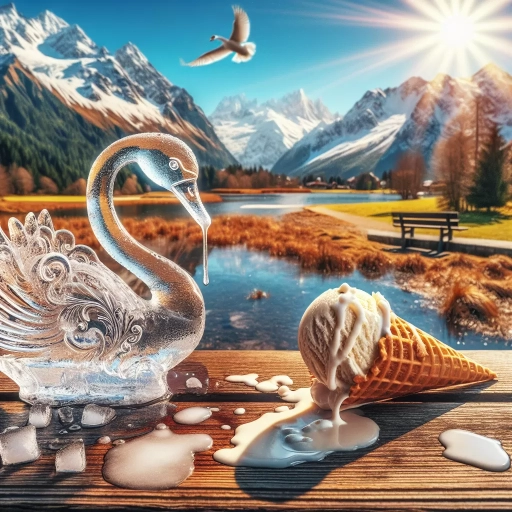
Melting, a fundamental process in both everyday life and scientific inquiry, is a phenomenon that transforms solids into liquids. This transition is not just a simple change of state but involves intricate physical and chemical mechanisms. To fully grasp the concept of melting, it is essential to delve into its underlying principles, explore its physical and chemical aspects, and examine its practical applications. In this article, we will first **Understand the Concept of Melting**, clarifying the definitions and basic principles that govern this process. We will then delve into the **Physical and Chemical Aspects of Melting**, discussing the thermodynamic and molecular changes that occur during melting. Finally, we will explore **Practical Applications and Examples of Melting**, highlighting how this process is utilized in various fields such as engineering, cooking, and environmental science. By understanding these facets, we can appreciate the complexity and importance of melting in our world. Let us begin by **Understanding the Concept of Melting**.
Understanding the Concept of Melting
Understanding the concept of melting is a fundamental aspect of physics and chemistry, offering insights into the behavior of materials under varying conditions. This article delves into the intricacies of melting, providing a comprehensive overview through three key sections. First, we explore the **Definition and Scientific Explanation** of melting, dissecting the molecular changes that occur as a substance transitions from solid to liquid. This foundational understanding sets the stage for a deeper analysis. Next, we examine **Types of Melting Processes**, highlighting the different mechanisms by which materials melt, including phase transitions and the role of external factors. Finally, we discuss **Key Factors Influencing Melting Points**, shedding light on how temperature, pressure, and purity affect the melting behavior of substances. By grasping these concepts, readers will gain a holistic view of melting and its significance in various scientific and practical contexts. Let us begin by defining and scientifically explaining what melting entails, laying the groundwork for a detailed exploration of this critical physical phenomenon.
Definition and Scientific Explanation
**Understanding the Concept of Melting** Melting, a fundamental process in the physical sciences, is the transition of a substance from the solid to the liquid phase. This phenomenon occurs when the temperature of the solid reaches its melting point, a specific temperature at which the solid and liquid phases of a substance coexist in equilibrium. The scientific explanation behind melting lies in the realm of thermodynamics and molecular dynamics. At the molecular level, solids are characterized by closely packed molecules arranged in a structured lattice. These molecules are held together by strong intermolecular forces such as ionic bonds, covalent bonds, or van der Waals forces. As energy is added to the system, typically in the form of heat, the molecules begin to vibrate more vigorously. When the temperature reaches the melting point, these vibrations become so intense that they overcome the intermolecular forces holding the lattice structure together. Consequently, the rigid structure breaks down, and the molecules gain enough kinetic energy to move freely past one another, transitioning into a liquid state. The melting process is also influenced by pressure; however, for most substances, the effect of pressure on the melting point is relatively minor compared to temperature. This is why melting points are generally defined at standard atmospheric pressure. The concept of latent heat of fusion is crucial here; it is the amount of energy required to change a unit mass of a substance from solid to liquid at its melting point without changing its temperature. This energy input does not increase the temperature but rather goes into breaking down the solid's lattice structure. Understanding melting is essential in various fields such as chemistry, physics, and engineering. For instance, in materials science, knowing the melting points of different materials helps in selecting appropriate materials for specific applications. In chemistry, melting points are used as a criterion for identifying substances and determining their purity. Additionally, in environmental science, understanding how melting affects ice caps and glaciers is critical for studying climate change. In summary, melting is a complex yet fascinating process that involves the transition from a solid to a liquid state due to increased thermal energy. It is governed by thermodynamic principles and influenced by molecular interactions and external conditions such as temperature and pressure. Grasping this concept not only enhances our understanding of physical sciences but also has practical implications across diverse scientific disciplines.
Types of Melting Processes
Melting, a fundamental phase transition in which a solid transforms into a liquid, encompasses various processes that are crucial in both natural and industrial contexts. Understanding these different types of melting processes is essential for grasping the complexities of this phenomenon. **1. Isothermal Melting:** Isothermal melting occurs when the temperature remains constant throughout the melting process. This type of melting is often observed in pure substances where the solid and liquid phases coexist at a specific temperature, known as the melting point. For instance, ice melts at 0°C (32°F) under standard atmospheric pressure, and this process happens isothermally. **2. Non-Isothermal Melting:** Non-isothermal melting, on the other hand, involves a change in temperature during the melting process. This is common in mixtures or impure substances where the melting point range is broader. For example, alloys or solutions may melt over a range of temperatures rather than at a single point. **3. Equilibrium Melting:** Equilibrium melting occurs when the solid and liquid phases are in thermodynamic equilibrium. This means that the rates of melting and freezing are equal, and there is no net change in the amount of solid or liquid present. This equilibrium is often seen in systems where there is sufficient time for the phases to adjust to the new conditions. **4. Nonequilibrium Melting:** Nonequilibrium melting happens when the system does not have enough time to reach equilibrium. This can occur under rapid heating conditions or in systems where nucleation sites for crystallization are limited. Nonequilibrium melting can lead to superheating or supercooling, where the solid remains solid above its melting point or the liquid remains liquid below its freezing point. **5. Phase Change Materials (PCMs) Melting:** Phase Change Materials (PCMs) are substances that absorb and release thermal energy by melting and freezing at a nearly constant temperature. These materials are used in various applications such as thermal energy storage systems, building insulation, and even clothing designed to regulate body temperature. **6. Microwave Melting:** Microwave melting involves the use of microwave energy to heat materials directly within their molecular structure. This method is particularly useful for melting metals and other conductive materials efficiently and uniformly. It is commonly used in industrial processes such as metal casting and sintering. **7. Laser Melting:** Laser melting uses focused laser beams to heat specific areas of a material precisely. This technique is widely used in additive manufacturing (3D printing) and surface treatment processes where high precision and localized heating are required. Understanding these different types of melting processes provides a comprehensive view of how materials behave under various conditions, which is crucial for both scientific research and industrial applications. Each type of melting process has its unique characteristics and applications, making it essential to recognize and differentiate between them to optimize processes and achieve desired outcomes. By delving into these specifics, one can better appreciate the intricacies involved in the concept of melting and its significance across diverse fields.
Key Factors Influencing Melting Points
Understanding the concept of melting is deeply intertwined with several key factors that influence the melting points of substances. The melting point, defined as the temperature at which a solid transforms into a liquid, is not a constant property but rather a dynamic value that can be affected by various physical and chemical parameters. One of the most significant factors is **intermolecular forces**, which include van der Waals forces, hydrogen bonds, and ionic bonds. Substances with stronger intermolecular forces require more energy to overcome these attractions, resulting in higher melting points. For instance, ionic compounds like sodium chloride (NaCl) have very high melting points due to the strong electrostatic attraction between positively charged cations and negatively charged anions. Another crucial factor is **molecular weight**. Generally, as the molecular weight of a substance increases, so does its melting point. This is because larger molecules have more electrons and a greater surface area, leading to stronger intermolecular forces. However, this trend is not absolute and can be influenced by the shape and polarity of the molecules. **Polarity** also plays a significant role; polar molecules tend to have higher melting points than nonpolar molecules of similar size due to the additional dipole-dipole interactions. The **crystal structure** of a solid is another influential factor. The arrangement of atoms or molecules within the crystal lattice affects the strength of the intermolecular forces and thus the melting point. For example, substances with a more ordered and tightly packed crystal structure typically have higher melting points compared to those with less ordered structures. **Pressure** is another critical factor that can influence melting points. According to the Clausius-Clapeyron equation, an increase in pressure generally raises the melting point of a substance because it becomes more difficult for the solid to transition into a liquid under higher pressure. However, there are exceptions such as water, which exhibits an anomalous behavior where its melting point decreases with increasing pressure. Additionally, **impurities** can significantly affect the melting point of a substance. The presence of impurities can lower the melting point by disrupting the crystal lattice structure and reducing the overall intermolecular forces within the solid. This phenomenon is known as "freezing-point depression" or "melting-point depression." Lastly, **isotopic composition** can also have an impact on melting points, particularly in elements where different isotopes may form slightly different crystal structures or have varying intermolecular forces due to differences in atomic mass. In summary, understanding these key factors—intermolecular forces, molecular weight, polarity, crystal structure, pressure, impurities, and isotopic composition—provides a comprehensive insight into why different substances exhibit unique melting points. These factors collectively determine how much energy is required for a solid to melt into a liquid state, making each substance's melting behavior distinct and predictable based on its specific properties.
Physical and Chemical Aspects of Melting
Understanding the physical and chemical aspects of melting is crucial for grasping the fundamental principles that govern this phase transition. Melting, a process where a solid transforms into a liquid, involves intricate changes at both the molecular and thermodynamic levels. This article delves into three key areas: Phase Transition and Thermodynamics, Molecular Changes During Melting, and Energy Requirements for Melting. Firstly, the phase transition from solid to liquid is deeply rooted in thermodynamic principles, which dictate the conditions under which melting occurs. This includes the role of temperature, pressure, and the latent heat of fusion. Secondly, at the molecular level, melting involves significant changes in the arrangement and motion of molecules, transitioning from a rigid structure to a more fluid state. Lastly, the energy requirements for melting are critical, as they determine the amount of heat needed to overcome the intermolecular forces holding the solid together. By exploring these aspects, we gain a comprehensive understanding of the melting process. Let us begin by examining the thermodynamic framework that underpins phase transitions, which sets the stage for understanding the broader physical and chemical changes that occur during melting. This foundational knowledge is essential for appreciating the complex interplay of forces and energies involved in this everyday yet fascinating phenomenon. Transitioning to Phase Transition and Thermodynamics, we will explore how these principles govern the melting process.
Phase Transition and Thermodynamics
Phase transition, a fundamental concept in thermodynamics, is the process by which a system changes from one phase of matter to another. This phenomenon is intricately linked with the principles of thermodynamics, which govern the relationships between heat, work, and energy. During a phase transition, such as melting, the system undergoes a significant change in its physical state without altering its chemical composition. For instance, when ice melts into water, the solid phase transforms into the liquid phase at a specific temperature (the melting point) and pressure. Thermodynamically, phase transitions are characterized by changes in the Gibbs free energy of the system. The Gibbs free energy, \(G\), is a measure of the energy available to do work in a system at constant temperature and pressure. At the melting point, the Gibbs free energy of the solid phase equals that of the liquid phase, making it energetically favorable for the transition to occur. This equilibrium is described by the Clausius-Clapeyron equation, which relates the slope of the phase boundary in a phase diagram to the enthalpy and entropy changes during the transition. The thermodynamic properties that drive phase transitions include enthalpy (\(H\)), entropy (\(S\)), and specific heat capacity (\(C_p\)). Enthalpy change (\(\Delta H\)) during melting is known as the latent heat of fusion, which is the energy required to change the state of a substance from solid to liquid without changing its temperature. Entropy change (\(\Delta S\)) reflects the disorder or randomness of the molecules; during melting, entropy increases as molecules gain more freedom of movement in the liquid state compared to the rigid structure of the solid state. Understanding these thermodynamic principles is crucial for predicting and controlling phase transitions. For example, in materials science, knowledge of phase transitions helps in designing materials with specific properties, such as superconductors or shape-memory alloys. In biological systems, phase transitions are vital for processes like protein folding and membrane fluidity, which are essential for cellular function. Moreover, the study of phase transitions has practical applications in various fields. In engineering, it is used to design efficient cooling systems and to understand the behavior of materials under different conditions. In environmental science, understanding phase transitions helps in predicting climate phenomena such as ice melting and its impact on global weather patterns. In summary, phase transitions are complex thermodynamic processes that involve significant changes in the physical state of a substance while maintaining its chemical integrity. The principles of thermodynamics provide a robust framework for understanding these transitions, enabling us to predict and manipulate them for various scientific and technological applications. This understanding is particularly relevant when examining the physical and chemical aspects of melting, as it underscores the intricate balance between energy, entropy, and molecular structure that governs this fundamental process.
Molecular Changes During Melting
During the process of melting, a substance undergoes significant molecular changes that transform it from a solid to a liquid state. At the molecular level, melting is characterized by the breaking of intermolecular forces that hold the molecules in a rigid, crystalline structure. In solids, molecules are tightly packed and arranged in a specific order due to strong intermolecular forces such as hydrogen bonds, ionic bonds, or van der Waals forces. As the temperature increases, the kinetic energy of the molecules also increases, causing them to vibrate more vigorously. When this kinetic energy surpasses the energy required to break these intermolecular forces, the solid begins to melt. As melting progresses, the rigid structure of the solid starts to disintegrate. The molecules gain enough energy to overcome their fixed positions and begin moving freely past one another. This increased mobility results in the loss of long-range order, leading to a more random arrangement of molecules typical of liquids. The breaking of these intermolecular bonds allows for greater spacing between molecules, which in turn increases the volume of the substance and reduces its density. The temperature at which this transition occurs is known as the melting point, a characteristic property of each substance. At this temperature, both solid and liquid phases coexist in equilibrium until all of the solid has melted. The energy required for melting is absorbed as latent heat of fusion, which does not change the temperature of the substance but rather changes its state from solid to liquid. In addition to these physical changes, some substances may also exhibit chemical changes during melting. For instance, certain polymers or biological molecules can undergo structural rearrangements or denaturation when heated. However, these chemical alterations are generally not part of the melting process itself but rather secondary effects that can occur under specific conditions. Understanding these molecular changes is crucial for various applications in fields such as materials science, chemistry, and engineering. For example, knowing how different materials melt can help in designing processes for manufacturing, recycling, and storage. Moreover, studying the melting behavior of biological molecules like proteins and nucleic acids provides insights into their stability and function under different conditions. In summary, the melting process involves a series of molecular transformations where intermolecular forces are broken, allowing molecules to transition from a rigid solid structure to a more fluid liquid state. This transition is marked by increased molecular mobility and a change in physical properties such as density and volume. Understanding these changes at the molecular level is essential for comprehending both the physical and chemical aspects of melting.
Energy Requirements for Melting
Melting, a fundamental process in both natural and industrial contexts, involves the transition of a substance from its solid to liquid state. This phase change is driven by the absorption of energy, which must overcome the intermolecular forces holding the solid structure together. The energy requirements for melting are influenced by several key factors, including the type of material, its purity, and environmental conditions. **Thermodynamic Considerations:** From a thermodynamic perspective, melting is an endothermic process, meaning it absorbs heat energy from the surroundings. The amount of energy required to melt a substance is quantified by its latent heat of fusion, which is the energy needed to change a unit mass of the substance from solid to liquid at its melting point. For example, water has a latent heat of fusion of approximately 334 joules per gram, indicating that 334 joules of energy must be absorbed by each gram of ice to convert it into liquid water without changing its temperature. **Material Properties:** Different materials have varying energy requirements due to differences in their molecular structures and intermolecular forces. Substances with strong intermolecular forces, such as ionic or covalent bonds, generally require more energy to melt compared to those with weaker forces like van der Waals interactions. For instance, metals like tungsten have high melting points due to their strong metallic bonds, necessitating significant amounts of energy for melting. In contrast, substances like wax or paraffin, which are held together by weaker van der Waals forces, melt at lower temperatures and require less energy. **Environmental Factors:** Environmental conditions also play a crucial role in determining the energy requirements for melting. Pressure, for instance, can affect the melting point of some substances. For water, an increase in pressure lowers its melting point—a phenomenon known as pressure melting point depression. This is why ice skates can glide smoothly over ice; the pressure exerted by the blades lowers the melting point of ice just enough to create a thin layer of water that reduces friction. **Purity and Impurities:** The purity of a substance can significantly impact its melting behavior. Pure substances typically have well-defined melting points and require consistent amounts of energy to melt. However, impurities can alter this behavior by either lowering or raising the melting point through a process known as freezing-point depression or elevation. For example, adding salt to ice lowers its melting point, which is why salt is used on icy roads during winter. **Industrial Applications:** Understanding the energy requirements for melting is crucial in various industrial processes. In metallurgy, precise control over melting temperatures and energies is essential for producing alloys with desired properties. Similarly, in food processing and pharmaceutical manufacturing, controlled melting processes ensure product quality and safety. For instance, chocolate manufacturers must carefully manage the melting and tempering processes to achieve the desired texture and appearance. In conclusion, the energy requirements for melting are multifaceted and influenced by a range of physical and chemical factors. By understanding these principles—whether it's the thermodynamic properties of latent heat of fusion or the impact of environmental conditions and impurities—scientists and engineers can optimize processes across diverse fields, from materials science to food technology. This knowledge not only enhances our ability to manipulate materials but also underscores the intricate balance between energy input and phase transitions that govern our everyday world.
Practical Applications and Examples of Melting
Melting, a fundamental physical process, has far-reaching implications across various domains, making it a crucial aspect of both industrial and everyday life. This article delves into the practical applications and examples of melting, highlighting its significance in three key areas. First, we explore the **Industrial Uses of Melting Processes**, where melting is a cornerstone in manufacturing, from the production of metals and alloys to the creation of plastics and glass. These processes are essential for modern industry, enabling the fabrication of materials that underpin our technological advancements. Second, we examine the **Environmental Impacts of Melting**, discussing how natural phenomena such as glacier melting and ice cap thawing affect global climate patterns and ecosystems. Understanding these impacts is vital for addressing climate change and its consequences. Finally, we look at **Everyday Examples of Melting Phenomena**, illustrating how melting occurs in our daily lives, from cooking and food preservation to seasonal changes in weather. By understanding these diverse applications, we can appreciate the multifaceted role of melting in shaping our world. Let us begin by exploring the industrial uses of melting processes, where the transformative power of this physical change is most evident.
Industrial Uses of Melting Processes
Melting processes are integral to various industrial sectors, where they play a crucial role in manufacturing, recycling, and material transformation. In the **metallurgy industry**, melting is essential for producing alloys and pure metals. For instance, steel production involves melting iron ore with other elements like carbon and manganese to create different grades of steel, which are then used in construction, automotive, and consumer goods. Similarly, aluminum smelting involves melting bauxite ore to produce aluminum ingots, which are further processed into sheets, foils, and other products used in packaging, aerospace, and electronics. In **chemical processing**, melting is used to purify substances and create new compounds. For example, the production of fertilizers often involves melting ammonium nitrate or other nitrogen-based compounds to ensure uniformity and purity. Additionally, in the **pharmaceutical industry**, melting points are critical in the synthesis of drugs and the formulation of dosage forms such as tablets and capsules. The precise control of melting temperatures helps in achieving the desired physical and chemical properties of pharmaceuticals. The **plastics industry** heavily relies on melting processes for manufacturing a wide range of products. Extrusion and injection molding techniques involve melting plastic pellets to form items like pipes, packaging materials, and consumer goods. The ability to control the melting point of plastics allows for the creation of materials with specific properties, such as flexibility, strength, and resistance to heat. In **recycling**, melting is a key step in reprocessing materials like metals and plastics. Scrap metal is melted down to produce new metal products, reducing the need for raw materials and conserving natural resources. Similarly, plastic recycling involves melting post-consumer plastics to create new products such as bottles, containers, and textiles. The **food industry** also utilizes melting processes, particularly in the production of chocolate and other confectionery items. Tempering chocolate involves heating and cooling it to achieve a stable crystal structure that gives chocolate its glossy appearance and snappy texture. Additionally, melting fats and oils is crucial in baking and cooking processes. Furthermore, **energy applications** benefit from melting processes. For example, in concentrated solar power systems, molten salt is used as a heat transfer fluid to store thermal energy, which can then be used to generate electricity even when the sun is not shining. This technology enhances the efficiency and reliability of renewable energy systems. In **construction**, melting processes are used in the production of asphalt and bitumen, which are essential for road surfacing and roofing materials. The quality of these materials is directly influenced by the melting and mixing processes, ensuring durability and performance under various environmental conditions. In summary, melting processes are fundamental across a broad spectrum of industries, enabling the transformation of raw materials into valuable products. The precise control of melting temperatures and conditions is critical for achieving desired properties and ensuring the quality of final products. Whether in metallurgy, chemical processing, plastics manufacturing, recycling, food production, energy applications, or construction, the industrial uses of melting processes underscore their importance in modern manufacturing and technology.
Environmental Impacts of Melting
The environmental impacts of melting are multifaceted and far-reaching, influencing various ecosystems and global climate dynamics. One of the most significant consequences is the rise in sea levels due to the melting of polar ice caps and glaciers. This not only threatens coastal communities with increased flooding and erosion but also disrupts marine habitats, affecting species that depend on these icy environments. For instance, the melting of Arctic sea ice has severe implications for polar bears, whose hunting grounds are shrinking, leading to malnutrition and reduced populations. In addition to sea-level rise, melting glaciers contribute to changes in global weather patterns. Glaciers act as natural reservoirs of freshwater, and their melting can alter river flow regimes, impacting agriculture, hydroelectric power generation, and drinking water supplies. This is particularly evident in regions like the Himalayas, where glacial melt affects the water security of millions of people downstream. Melting permafrost, a layer of soil and rock that remains frozen for two or more consecutive years, releases methane and carbon dioxide—potent greenhouse gases that accelerate global warming. This feedback loop exacerbates climate change, creating a self-reinforcing cycle where more warming leads to more melting, which in turn releases more greenhouse gases. Furthermore, the loss of ice cover can have profound effects on local ecosystems. For example, the melting of alpine glaciers exposes previously ice-covered terrain to new plant and animal species, leading to changes in biodiversity. However, this can also result in the loss of unique alpine flora and fauna adapted to these cold environments. In terms of practical applications and examples, understanding the environmental impacts of melting is crucial for developing strategies to mitigate these effects. For instance, monitoring glacier health through satellite imagery and field observations helps scientists predict future changes in sea levels and water availability. This information is vital for urban planning, particularly in coastal areas, where adaptive measures such as seawalls and flood-resistant construction can be implemented. Moreover, initiatives aimed at reducing greenhouse gas emissions, such as transitioning to renewable energy sources and implementing carbon capture technologies, are essential for slowing the rate of melting. Conservation efforts focused on preserving glacier habitats and supporting species adaptation also play a critical role in managing the ecological impacts of melting. In conclusion, the environmental impacts of melting are complex and interconnected, influencing everything from global climate patterns to local biodiversity. By understanding these dynamics and applying practical solutions, we can better manage the consequences of melting and work towards a more sustainable future.
Everyday Examples of Melting Phenomena
Melting phenomena are ubiquitous in our everyday lives, manifesting in various forms that often go unnoticed yet play crucial roles in our daily routines and environments. One of the most common examples is the melting of ice and snow during seasonal transitions. As temperatures rise in spring, the frozen water on roads, sidewalks, and rooftops begins to melt, transforming solid ice into liquid water. This process not only affects the physical landscape but also impacts urban planning and infrastructure, as municipalities must manage water runoff and potential flooding. In culinary contexts, melting is a fundamental process. Chefs rely on the melting of butter and chocolate to create smooth sauces and desserts. The precise control of melting temperatures is essential for achieving the desired consistency and flavor in dishes like hollandaise sauce or chocolate truffles. Similarly, in baking, the melting of fats and sugars contributes to the texture and structure of pastries and cakes. The automotive industry also relies heavily on melting phenomena. For instance, the manufacturing of car parts involves melting metals such as aluminum and steel to shape them into various components. Additionally, the use of antifreeze in car radiators prevents engine coolant from freezing in cold temperatures, ensuring that the engine operates efficiently by maintaining a stable temperature through the controlled melting and freezing points of the coolant. In environmental science, the melting of polar ice caps and glaciers is a significant concern due to its implications for global climate change. Rising global temperatures cause these massive ice formations to melt at an alarming rate, contributing to sea-level rise and altering ecosystems. This phenomenon is closely monitored by scientists who study its impact on biodiversity, ocean currents, and weather patterns. Furthermore, melting plays a critical role in medical applications. In pharmaceuticals, the melting point of drugs is crucial for their stability and effectiveness. For example, certain medications must be stored below their melting points to maintain their potency. In medical procedures, the use of heat to melt adhesives or seal tissues is common in surgeries and wound care. Even in recreational activities, melting is integral. Skiers and snowboarders experience the effects of melting snow firsthand as warmer temperatures soften the snow, changing its texture from powdery to slushy. This transformation affects the performance of their equipment and the overall skiing experience. Lastly, in construction and architecture, melting phenomena are considered during the design phase. For instance, the selection of materials for roofing must account for the melting of snow and ice to ensure that water does not seep into buildings. Similarly, the use of de-icing salts on walkways and roads prevents ice formation by lowering the freezing point of water, thereby enhancing safety during winter months. In summary, melting phenomena are not just abstract scientific concepts but are deeply intertwined with various aspects of our daily lives. From culinary arts to environmental science, automotive manufacturing to medical applications, understanding and managing melting processes is essential for efficiency, safety, and sustainability.