What Is Mer
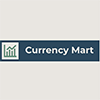
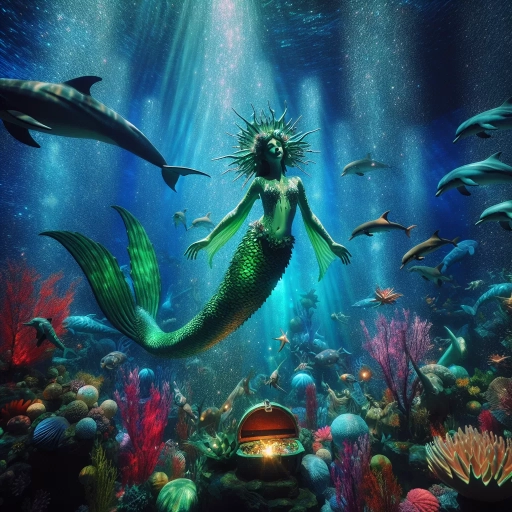
In the realm of cutting-edge technology, the term "mer" has emerged as a significant concept, particularly in fields such as data storage, artificial intelligence, and advanced materials. This innovative technology holds the potential to revolutionize various industries by offering unprecedented efficiency, durability, and versatility. To fully grasp the impact of mer, it is essential to delve into its fundamental principles, explore its diverse applications, and consider its future prospects. Understanding the basics of mer is crucial as it lays the groundwork for appreciating its wide-ranging uses across different sectors. From enhancing data storage capacities to driving advancements in AI and material science, mer's applications are vast and multifaceted. Moreover, as research continues to evolve, the future prospects and innovations in mer promise to unlock even more transformative possibilities. In this article, we will begin by understanding the basics of mer, setting the stage for a comprehensive exploration of its applications and future innovations.
Understanding the Basics of Mer
In the realm of financial markets, understanding the intricacies of financial instruments is crucial for making informed decisions. One such instrument that has garnered significant attention is the Mortgage-Backed Security (MBS), often referred to as a "mer." To delve into the world of MBS, it is essential to grasp its fundamental aspects. This article aims to provide a comprehensive overview by exploring three key areas: **Definition and Origin**, which will trace the roots and basic concept of MBS; **Key Components and Structure**, which will dissect the various elements that constitute an MBS; and **Historical Context and Evolution**, which will examine how MBS have evolved over time. By understanding these facets, readers will gain a solid foundation in the basics of MBS, enabling them to navigate the complexities of this financial product with confidence. This knowledge is vital for both investors and financial professionals seeking to leverage MBS effectively. Therefore, let us embark on this journey to **Understanding the Basics of Mer**.
Definition and Origin
**Definition and Origin** To delve into the concept of "mer," it is essential to understand its definition and origin. The term "mer" can have multiple meanings depending on the context, but in its most fundamental sense, it refers to a unit of measurement or a component within a larger system. In the realm of technology and software development, "mer" is often associated with the Mer project, an open-source software distribution that aims to provide a flexible and modular operating system framework. This framework is designed to be highly customizable, allowing developers to create tailored solutions for various devices and applications. The origin of the Mer project dates back to 2010 when it was initiated as a fork of the MeeGo operating system. MeeGo itself was a collaboration between Intel and Nokia, intended to create an open-source platform for mobile devices. However, following Nokia's shift towards Windows Phone, the MeeGo community decided to continue the project independently, leading to the birth of Mer. Since then, Mer has evolved significantly, incorporating contributions from a diverse community of developers and becoming a robust foundation for building specialized operating systems. One of the key aspects of Mer is its emphasis on modularity and flexibility. Unlike monolithic operating systems, Mer is composed of smaller, independent components that can be easily integrated or replaced. This modular architecture allows developers to create highly optimized systems for specific use cases, such as automotive infotainment systems, IoT devices, or even traditional desktop environments. The project's open-source nature ensures that it remains community-driven, fostering innovation and collaboration among developers worldwide. Understanding the basics of Mer involves recognizing its roots in the MeeGo project and its evolution into a versatile operating system framework. By leveraging its modular design, developers can build highly customized solutions that meet the unique demands of various industries and applications. This flexibility, combined with the community's continuous support and development, makes Mer an attractive choice for those seeking to create tailored operating systems that are both efficient and adaptable. As technology continues to advance and diversify, the Mer project stands as a testament to the power of open-source collaboration and innovation in software development.
Key Components and Structure
Understanding the basics of Mer, a version control system designed for distributed development, hinges on grasping its key components and structure. At its core, Mer is built around several fundamental elements that work in harmony to manage code repositories efficiently. Firstly, **Repositories** are the central storage units in Mer. These repositories contain all the files, history, and metadata associated with a project. Each repository is self-contained and can be cloned to create a local copy, allowing developers to work independently before merging changes back into the mainline. **Changesets** are another critical component. A changeset represents a set of changes made to the repository, including new files, modified files, and deleted files. Each changeset is uniquely identified by a hash and includes information about the author, date, and a description of the changes. This allows for precise tracking and rollback of modifications if needed. The **Revision Graph** is a visual representation of how changesets are related to each other. It shows the lineage of changesets, illustrating how different branches and merges have evolved over time. This graph is essential for understanding the history of a project and resolving conflicts that may arise during collaborative development. **Branches** enable multiple lines of development within a single repository. They allow teams to work on different features or versions of a project simultaneously without interfering with each other's work. Branches can be merged back into the mainline when they are complete, ensuring that all changes are integrated seamlessly. **Tags** and **Bookmarks** serve as markers within the revision graph. Tags are used to mark significant points in the history of a project, such as releases or milestones, while bookmarks provide a way to keep track of specific changesets that are frequently referenced. The **Mer Command Line Interface (CLI)** is the primary tool for interacting with Mer repositories. It offers a wide range of commands for creating, managing, and manipulating changesets, branches, tags, and bookmarks. The CLI provides flexibility and power, making it an indispensable tool for developers working with Mer. Lastly, **Extensions** play a crucial role in enhancing the functionality of Mer. These extensions can add new commands, modify existing behavior, or integrate Mer with other tools and systems. They allow developers to tailor Mer to their specific needs and workflows. In summary, understanding these key components—repositories, changesets, revision graphs, branches, tags/bookmarks, the CLI interface, and extensions—is essential for mastering Mer. By grasping how these elements interact and support each other, developers can leverage Mer's full potential to manage complex projects efficiently and effectively. This foundational knowledge sets the stage for more advanced topics in using Mer for collaborative software development.
Historical Context and Evolution
The historical context and evolution of MER (Mars Exploration Rover) are deeply intertwined with the broader narrative of space exploration and technological advancement. The journey began in the early 20th century, when scientists first proposed the idea of sending robotic missions to other planets. However, it wasn't until the 1960s that NASA's Mariner missions successfully imaged Mars, laying the groundwork for future exploration. The Viking missions in the 1970s marked a significant milestone by landing on Mars' surface, though they were limited in their mobility and scope. The real turning point came with the Mars Pathfinder mission in 1996, which included the Sojourner rover—the first rover to explore Mars. This small but robust rover demonstrated the feasibility of mobile exploration on another planet. Building on this success, NASA launched the MER program in 2003 with two rovers: Spirit and Opportunity. Designed to operate for just 90 days, these rovers far exceeded expectations, with Spirit operating until 2010 and Opportunity continuing its mission until 2019. The MER program was a technological marvel, featuring advanced navigation systems, solar panels for power, and a suite of scientific instruments to analyze Martian geology and climate. These rovers provided unprecedented insights into Mars' geological history, discovering evidence of ancient waterways and lakes that suggested a once-habitable environment. Their extended lifespan allowed for continuous data collection and adaptation to new challenges, such as navigating treacherous terrain and surviving harsh Martian winters. The success of MER paved the way for subsequent missions like the Curiosity Rover, launched in 2011, which is equipped with even more sophisticated tools and has been exploring Gale Crater since 2012. The Perseverance Rover, launched in 2020, continues this legacy with cutting-edge technology aimed at searching for signs of past or present life on Mars. Throughout this evolution, advancements in robotics, communication systems, and scientific instrumentation have been crucial. Each mission has built upon the lessons learned from its predecessors, driving innovation and pushing the boundaries of what is possible in interplanetary exploration. Understanding the historical context and evolution of MER is essential for appreciating the complexities and achievements of Mars exploration, as well as the ongoing quest to unravel the mysteries of the Red Planet. This narrative not only highlights human ingenuity but also underscores the importance of continuous scientific inquiry and technological innovation in expanding our understanding of the universe.
Applications and Uses of Mer
Mer, a versatile and multifaceted element, has garnered significant attention across various sectors due to its diverse applications and uses. From industrial and commercial contexts where it plays a crucial role in manufacturing and technology, to scientific research and development where it aids in groundbreaking discoveries, mer's impact is profound. Additionally, its environmental implications and sustainability considerations are increasingly important as the world shifts towards more eco-friendly practices. This article delves into the industrial and commercial uses of mer, highlighting its role in driving innovation and efficiency. It also explores its significance in scientific research and development, where it facilitates advancements in fields such as medicine and technology. Furthermore, the article examines the environmental impact and sustainability of mer, discussing both the challenges and opportunities it presents. By understanding these facets, readers will gain a comprehensive insight into the applications and uses of mer, setting the stage for a deeper exploration of its fundamental properties and principles in the section titled "Understanding the Basics of Mer."
Industrial and Commercial Uses
Industrial and commercial uses of Mer, a versatile and innovative material, are multifaceted and transformative. In the industrial sector, Mer is utilized for its exceptional durability and resistance to wear and tear, making it an ideal choice for manufacturing components that require high performance under demanding conditions. For instance, Mer is used in the production of advanced machinery parts, such as gears and bearings, where its ability to withstand extreme temperatures and mechanical stress is crucial. Additionally, its lightweight yet robust nature makes it a preferred material for aerospace applications, contributing to the development of more efficient and sustainable aircraft. In the commercial realm, Mer's unique properties also find significant applications. It is widely used in the automotive industry for producing high-strength, low-weight components that enhance vehicle performance while reducing fuel consumption. Furthermore, Mer's corrosion resistance and ease of maintenance make it a popular choice for infrastructure projects such as bridges and high-rise buildings. Its aesthetic appeal combined with functional benefits also makes it suitable for architectural features like facades and cladding. Moreover, Mer's adaptability extends into consumer goods. It is incorporated into various products such as sports equipment, where its strength-to-weight ratio provides athletes with superior performance tools. In electronics, Mer is used in the manufacture of high-quality connectors and switches due to its excellent conductivity and reliability. The healthcare sector also benefits from Mer's biocompatibility and sterilization resistance, making it an essential material for medical devices and implants. The sustainability aspect of Mer is another significant factor driving its adoption across industries. Its recyclable nature and minimal environmental impact during production align with global efforts towards more eco-friendly practices. This not only reduces waste but also supports companies in achieving their sustainability goals. In summary, the industrial and commercial uses of Mer are diverse and impactful, leveraging its unique combination of strength, durability, and sustainability to drive innovation across various sectors. Whether in heavy-duty industrial applications or consumer products, Mer continues to play a vital role in enhancing performance, efficiency, and environmental responsibility. Its versatility ensures that it remains a cornerstone material for future technological advancements and industrial progress.
Scientific Research and Development
Scientific research and development (R&D) are the cornerstones of innovation, driving advancements in various fields and transforming theoretical concepts into practical applications. Within the context of Materials Engineering Research (MER), R&D plays a pivotal role in discovering new materials, enhancing existing ones, and optimizing their properties for diverse uses. This process involves a systematic approach, starting with fundamental research to understand the underlying principles of material behavior. Scientists conduct experiments, gather data, and analyze results to identify patterns and trends that can be leveraged for material improvement. The R&D cycle in MER is iterative and collaborative, often involving multidisciplinary teams of chemists, physicists, engineers, and mathematicians. These teams employ cutting-edge technologies such as computational modeling, nanotechnology, and advanced manufacturing techniques to design and test materials with specific attributes. For instance, researchers might focus on developing sustainable materials that are biodegradable or recyclable, addressing environmental concerns while maintaining performance standards. Another area of focus could be the creation of smart materials that respond to environmental stimuli, such as shape-memory alloys or self-healing polymers. The applications of these R&D efforts are far-reaching and impactful. In the aerospace industry, lightweight yet incredibly strong materials like carbon fiber composites are crucial for reducing fuel consumption and enhancing safety. In healthcare, biocompatible materials are being developed for medical implants and drug delivery systems, improving patient outcomes and quality of life. Additionally, advancements in energy storage materials are driving the development of more efficient batteries and supercapacitors, which are essential for the widespread adoption of renewable energy sources. Moreover, R&D in MER often leads to breakthroughs that have broader societal implications. For example, research on advanced ceramics has led to the development of more durable and efficient components for water filtration systems, contributing to global efforts to ensure clean water access. Similarly, innovations in polymer science have enabled the creation of flexible electronics and wearable technology, opening new avenues for consumer electronics and wearable health monitoring devices. In summary, scientific research and development in Materials Engineering Research are vital for pushing the boundaries of what is possible with materials. By combining rigorous scientific inquiry with innovative engineering solutions, researchers can create materials that meet the complex demands of modern society, from sustainability and healthcare to energy and technology. This ongoing cycle of discovery and application ensures that MER continues to drive progress across multiple sectors, enhancing our daily lives and shaping the future.
Environmental Impact and Sustainability
The environmental impact and sustainability of Mer, a versatile and innovative material, are crucial considerations in its applications and uses. As the world grapples with climate change, resource depletion, and pollution, materials like Mer offer promising solutions. Mer's composition and production process are designed to minimize environmental footprint. For instance, Mer can be derived from renewable resources, reducing reliance on fossil fuels and lowering greenhouse gas emissions. This sustainable sourcing not only conserves natural resources but also supports biodiversity by promoting eco-friendly practices in agriculture and forestry. In terms of end-of-life management, Mer is engineered to be recyclable and biodegradable, significantly reducing waste and the need for landfills. This circular economy approach ensures that Mer products can be reused or decomposed without harming the environment. Additionally, Mer's durability and longevity mean that it requires less frequent replacement compared to traditional materials, thereby reducing the overall demand on raw materials and energy consumption. The applications of Mer further underscore its sustainability. In construction, Mer can replace traditional building materials that have high carbon footprints, such as concrete and steel. Its lightweight yet robust properties make it an ideal choice for sustainable architecture, enabling the creation of energy-efficient buildings that require less energy for heating and cooling. In packaging, Mer offers a biodegradable alternative to plastics, helping to combat the global plastic pollution crisis by reducing marine litter and protecting wildlife. Moreover, Mer's versatility extends to agricultural uses where it can enhance soil health and water retention, improving crop yields while reducing the need for synthetic fertilizers and pesticides. This not only supports sustainable agriculture but also helps in maintaining soil biodiversity and mitigating the effects of climate change on food security. In conclusion, the environmental impact and sustainability of Mer are foundational to its value proposition. By leveraging renewable resources, promoting recyclability and biodegradability, and offering durable solutions across various industries, Mer stands as a beacon of innovation in the quest for a more sustainable future. Its applications not only address immediate environmental challenges but also pave the way for long-term ecological balance and resilience. As the world continues to seek out sustainable materials that align with environmental stewardship, Mer emerges as a compelling choice for those committed to reducing their ecological footprint while driving technological advancement.
Future Prospects and Innovations in Mer
In the rapidly evolving landscape of technology and innovation, the future prospects and innovations in the field of Mer are poised to revolutionize various sectors. This article delves into the exciting advancements and potential challenges that lie ahead. We will explore **Emerging Technologies and Trends**, highlighting cutting-edge developments that are set to transform the industry. Additionally, we will discuss **Potential Challenges and Limitations**, examining the obstacles that must be overcome to fully harness these innovations. Finally, we will analyze the **Global Impact and Future Directions**, considering how these advancements will shape the world and guide future research. Understanding these aspects is crucial for grasping the broader implications of Mer's evolution. To fully appreciate these insights, it is essential to start by **Understanding the Basics of Mer**, laying the groundwork for a comprehensive exploration of its future trajectory. By combining these perspectives, we aim to provide a holistic view of Mer's potential and its role in shaping the future.
Emerging Technologies and Trends
As we delve into the future prospects and innovations in the realm of MER (Manufacturing Execution Systems), it becomes evident that emerging technologies and trends are poised to revolutionize the industry. At the forefront of these advancements is the integration of Artificial Intelligence (AI) and Machine Learning (ML), which are transforming traditional manufacturing processes into intelligent, adaptive systems. AI-driven predictive maintenance, for instance, enables real-time monitoring and proactive intervention, significantly reducing downtime and enhancing overall efficiency. Additionally, ML algorithms can analyze vast datasets to optimize production workflows, predict demand, and improve product quality. The Internet of Things (IoT) is another critical trend, as it interconnects various devices and sensors within the manufacturing ecosystem, fostering a seamless flow of data. This interconnectedness allows for real-time monitoring and control, enabling manufacturers to make data-driven decisions that drive productivity and reduce costs. Furthermore, advancements in robotics and automation are leading to the development of more sophisticated and versatile machines that can perform complex tasks with precision, thereby enhancing safety and reducing human error. Blockchain technology is also making inroads into MER, offering a secure and transparent way to manage supply chains and track product lifecycle. By leveraging blockchain, manufacturers can ensure the authenticity of components, trace products through the supply chain, and maintain compliance with regulatory standards. Moreover, the adoption of Augmented Reality (AR) and Virtual Reality (VR) is enhancing training programs and operational procedures by providing immersive experiences that simulate real-world scenarios, thereby improving worker skills and reducing the risk of accidents. Cloud computing plays a pivotal role in MER by providing scalable infrastructure that supports the processing of large datasets generated by IoT devices and other sources. Cloud-based solutions facilitate collaboration across different departments and locations, ensuring that all stakeholders have access to the same information in real-time. This integration of cloud technology with other emerging trends such as 5G networks promises even faster data transmission rates, lower latency, and greater reliability. In conclusion, the convergence of these emerging technologies—AI, ML, IoT, blockchain, AR/VR, cloud computing, and 5G networks—is set to redefine the landscape of MER. As these innovations continue to evolve and mature, they will drive unprecedented levels of efficiency, productivity, and innovation in manufacturing execution systems. By embracing these trends, manufacturers can position themselves at the forefront of industry transformation and unlock new avenues for growth and competitiveness in an increasingly digital world.
Potential Challenges and Limitations
As we delve into the future prospects and innovations in MER (Methane-Ethylene Rubber), it is crucial to acknowledge the potential challenges and limitations that may impede its full realization. One of the primary hurdles is the scalability of production processes. Currently, MER synthesis involves complex chemical reactions that require precise control over reaction conditions, which can be challenging to replicate on a large scale. This scalability issue not only affects production costs but also hampers the widespread adoption of MER in various industries. Additionally, environmental concerns play a significant role; while MER itself is a more sustainable alternative to traditional rubber materials, the production process involves chemicals and energy consumption that could have adverse environmental impacts if not managed properly. Another challenge lies in the material's durability and performance under extreme conditions. Although MER exhibits superior resistance to certain chemicals and abrasion compared to conventional rubbers, its long-term performance in harsh environments such as high temperatures or exposure to aggressive chemicals remains a subject of ongoing research. Ensuring consistent quality across different batches is also a challenge due to the variability in raw material sources and processing conditions. From an economic perspective, the cost of raw materials and the complexity of the manufacturing process contribute to higher production costs, making MER less competitive with traditional rubber materials in some applications. Furthermore, regulatory frameworks may need to be adapted or expanded to accommodate the unique properties and uses of MER, which could delay its market entry. Innovations in MER also face intellectual property challenges as companies and researchers navigate patent landscapes to protect their discoveries while avoiding infringement on existing patents. This legal complexity can slow down the pace of innovation and collaboration. Despite these challenges, ongoing research and development are addressing these limitations. Advances in green chemistry and process optimization are expected to reduce environmental footprints and improve scalability. New formulations and additives are being explored to enhance durability and performance under various conditions. Economic incentives such as subsidies for sustainable materials could help offset higher production costs, making MER more viable in the market. In conclusion, while potential challenges and limitations exist for MER, they also present opportunities for innovation and improvement. Addressing these issues through continued research, technological advancements, and strategic collaborations will be pivotal in unlocking the full potential of MER and ensuring its successful integration into future applications. By overcoming these hurdles, we can harness the unique benefits of MER to drive sustainability and innovation across multiple industries.
Global Impact and Future Directions
As we delve into the future prospects and innovations in MER (Methane Emissions Reduction), it is crucial to consider the global impact and future directions of these efforts. The reduction of methane emissions has far-reaching implications that extend beyond environmental benefits, influencing economic, social, and health outcomes worldwide. Methane, a potent greenhouse gas with a global warming potential 28 times higher than carbon dioxide over a 100-year period, is primarily emitted through human activities such as agriculture, natural gas systems, and landfills. Reducing these emissions can significantly mitigate climate change, helping to achieve the goals set forth in the Paris Agreement. Globally, initiatives to reduce methane emissions are gaining momentum. For instance, the Global Methane Pledge, launched at COP26, aims to reduce methane emissions by 30% by 2030 compared to 2020 levels. This collective effort involves over 100 countries and underscores the recognition of methane's critical role in addressing climate change. Technological innovations are also driving progress; advanced sensors and drones are being deployed to detect and repair methane leaks in real-time, while biogas capture systems are transforming agricultural waste into clean energy. The economic benefits of methane reduction are substantial. By capturing and utilizing methane from landfills and agricultural operations, communities can generate revenue through the sale of biogas as a clean energy source. Additionally, reducing methane leaks in the oil and gas sector can prevent financial losses due to lost product. These economic incentives are likely to drive further investment in MER technologies. From a social perspective, reducing methane emissions can improve public health by decreasing air pollution. Methane is often released alongside other harmful gases like volatile organic compounds (VOCs) and particulate matter, which contribute to respiratory diseases and other health issues. Cleaner air quality resulting from MER efforts can lead to better health outcomes and improved quality of life for communities near emission sources. Looking ahead, future directions in MER will likely involve more sophisticated technologies and integrated policy frameworks. Artificial intelligence and machine learning could enhance predictive maintenance and leak detection capabilities, while blockchain technology might ensure transparency and accountability in emission reporting. Policy-wise, stricter regulations and incentives for adopting best practices will be essential in driving widespread adoption of MER strategies. In conclusion, the global impact of reducing methane emissions is multifaceted and profound. As we move forward, it is imperative that we continue to innovate and implement effective strategies for MER. By leveraging technology, fostering international cooperation, and aligning economic incentives with environmental goals, we can create a more sustainable future where the benefits of methane reduction are realized across all sectors of society.