What Is Eht
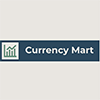
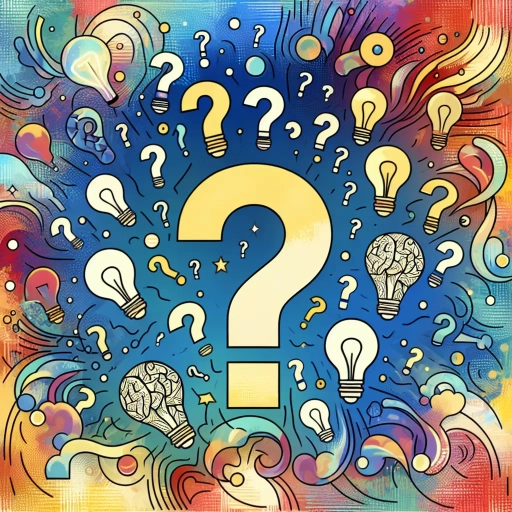
The Event Horizon Telescope (EHT) has revolutionized our understanding of the universe, offering unprecedented insights into the mysteries of black holes. This groundbreaking project has captured the imagination of scientists and the public alike, providing a window into the cosmos that was previously unimaginable. In this article, we will delve into the intricacies of EHT, starting with **Understanding the Basics of EHT**, where we explore the fundamental principles behind this innovative technology. We will then dive deeper into **How EHT Works: Technical Insights**, uncovering the sophisticated techniques and technologies that enable the EHT to achieve its remarkable observations. Finally, we will examine **Impact and Future Directions of EHT Research**, discussing how these findings are reshaping our knowledge of the universe and what future discoveries might hold. By understanding these aspects, we can appreciate the full scope of EHT's significance and its potential to continue transforming our understanding of the cosmos. Let's begin by **Understanding the Basics of EHT**.
Understanding the Basics of EHT
In the realm of advanced scientific inquiry, the Event Horizon Telescope (EHT) stands as a groundbreaking achievement, pushing the boundaries of our understanding of the universe. This innovative project has captivated the imagination of scientists and the public alike, offering unprecedented insights into the mysteries of black holes. To fully appreciate the significance of EHT, it is essential to delve into its foundational aspects. This article will explore the **Definition and Historical Context** of EHT, tracing its origins and the scientific landscape that led to its development. We will also examine the **Key Components and Technologies** that make EHT possible, highlighting the sophisticated engineering and collaborative efforts involved. Additionally, we will look at **Early Milestones and Breakthroughs**, detailing the pivotal moments that have marked EHT's journey towards capturing the first-ever image of a black hole. By understanding these core elements, readers will gain a comprehensive grasp of what makes EHT a revolutionary tool in modern astrophysics, ultimately leading to a deeper appreciation of **Understanding the Basics of EHT**.
Definition and Historical Context
**Definition and Historical Context** Understanding the basics of Event Horizon Telescope (EHT) begins with grasping its definition and historical context. The EHT is a network of telescopes that work together to form a virtual Earth-sized telescope, enabling scientists to observe objects in the universe with unprecedented resolution. This innovative technology allows for the direct imaging of black holes, a feat previously considered impossible due to their incredibly small angular sizes and the vast distances involved. Historically, the concept of black holes dates back to the 18th century with John Michell's proposal of "dark stars," but it wasn't until the 20th century that the modern understanding of black holes developed. Albert Einstein's theory of general relativity, introduced in 1915, predicted the existence of these cosmic phenomena. However, it wasn't until the 1960s that the term "black hole" was coined by American physicist John Wheeler. The idea of an Event Horizon Telescope was first proposed in the 1960s by Roger Penrose and Stephen Hawking, who theorized that it could be possible to observe the shadow of a black hole against the surrounding light. However, technological limitations at the time made this idea seem more like science fiction than reality. It wasn't until the advent of advanced radio astronomy techniques and the development of Very Long Baseline Interferometry (VLBI) in the latter half of the 20th century that the EHT project became feasible. The EHT project officially began in 2009 with a collaboration between various international research institutions. By combining data from a network of radio telescopes located around the globe, scientists can achieve the high angular resolution needed to observe objects at the event horizon of a black hole—the point beyond which nothing, including light, can escape its gravitational pull. This collaborative effort culminated in 2019 with the first-ever image of a black hole, captured at the center of galaxy M87. This groundbreaking achievement not only validated decades of theoretical work but also opened new avenues for understanding some of the universe's most mysterious phenomena. In summary, the EHT represents a significant leap forward in astronomical research, bridging theoretical predictions with observational evidence. Its development is rooted in centuries-old scientific curiosity and decades-long technological advancements, making it a testament to human ingenuity and collaborative scientific endeavor. As we continue to explore and understand more about black holes through EHT observations, we are poised to uncover deeper secrets about the cosmos and our place within it.
Key Components and Technologies
Understanding the basics of Extended Haplotype Testing (EHT) requires a deep dive into its key components and technologies. At the heart of EHT lies the concept of haplotypes, which are sets of genetic variants that are inherited together on a single chromosome. The primary goal of EHT is to identify these haplotypes and their associations with specific traits or diseases. One crucial component is **Next-Generation Sequencing (NGS)**, which enables the rapid and cost-effective sequencing of entire genomes. NGS technologies, such as Illumina and PacBio, generate vast amounts of genomic data that are essential for identifying haplotypes. Another vital technology is **Single Nucleotide Polymorphism (SNP) arrays**, which help in genotyping individuals by detecting variations at specific points in the genome. These arrays are often used in conjunction with NGS data to validate findings and improve resolution. **Phasing algorithms** are another key component, as they reconstruct the haplotypes from genotypic data. Advanced computational methods like HapMap and SHAPEIT2 are employed to phase genotypes accurately, taking into account recombination patterns and linkage disequilibrium. **Genomic annotation tools** also play a significant role by providing context to the identified haplotypes, helping researchers understand their functional implications. **Machine learning and statistical analysis** are integral to EHT, allowing researchers to identify significant associations between haplotypes and phenotypes. Techniques such as logistic regression, principal component analysis, and genome-wide association studies (GWAS) are used to filter out noise and highlight meaningful correlations. Additionally, **bioinformatics pipelines** streamline the process from raw data to interpretable results, ensuring efficiency and reproducibility. Moreover, **population genetics databases** like the 1000 Genomes Project and HapMap provide essential reference data for comparing and validating haplotype findings. These databases offer a wealth of information on genetic diversity across different populations, which is critical for understanding the evolutionary context of haplotypes. In summary, the synergy between NGS, SNP arrays, phasing algorithms, genomic annotation tools, machine learning techniques, bioinformatics pipelines, and population genetics databases forms the robust framework of EHT. By leveraging these technologies and components, researchers can uncover complex genetic relationships that underpin human traits and diseases, ultimately advancing our understanding of genetic inheritance and its implications for health and medicine.
Early Milestones and Breakthroughs
Understanding the basics of the Event Horizon Telescope (EHT) requires a deep dive into its early milestones and breakthroughs, which have been instrumental in shaping our current understanding of black holes. The EHT project began taking shape in the early 2000s, driven by a vision to directly observe the environment around black holes. One of the first significant milestones was the formation of the EHT collaboration in 2009, bringing together researchers from around the world to pool their expertise and resources. This collaborative effort was crucial for overcoming the technical challenges associated with imaging objects that are millions or even billions of light-years away. A key breakthrough came with the development of Very Long Baseline Interferometry (VLBI), a technique that allows multiple telescopes to act as a single, Earth-sized telescope. By synchronizing observations across these telescopes, scientists could achieve the high angular resolution needed to resolve the vicinity of a black hole. The first successful VLBI experiment involving the EHT was conducted in 2017, when eight radio telescopes around the globe were synchronized to form a virtual Earth-sized telescope. This experiment culminated in the capture of the first-ever image of a black hole, located at the center of galaxy M87, which was unveiled to the public in April 2019. The imaging process itself was a monumental achievement, involving sophisticated algorithms and computational power. The Event Horizon Imaging Collaboration developed novel methods to reconstruct images from the raw data collected by the EHT network. These methods included the use of machine learning algorithms to correct for atmospheric distortions and other sources of noise, ensuring that the final image accurately represented the environment around the black hole. The success of this project has not only provided visual evidence for the existence of black holes but also opened new avenues for astrophysical research. By directly observing these cosmic phenomena, scientists can now study the dynamics of accretion disks, the behavior of matter in extreme gravitational fields, and even test predictions made by Einstein's theory of general relativity. The EHT's early milestones and breakthroughs have thus marked a significant shift in our understanding of black holes, transforming them from theoretical constructs to observable objects that continue to fascinate and intrigue us. As the EHT continues to evolve with new technologies and collaborations, it promises to reveal even more secrets about the universe's most enigmatic objects.
How EHT Works: Technical Insights
The Event Horizon Telescope (EHT) is a groundbreaking astronomical project that has revolutionized our understanding of the universe by capturing the first-ever image of a black hole. This achievement was made possible through a combination of cutting-edge technologies and meticulous scientific processes. At the heart of EHT's success lies **Radio Interferometry and Data Collection**, which involves synchronizing a network of telescopes around the globe to form a virtual Earth-sized telescope. This technique allows for unprecedented resolution and the ability to observe distant objects with remarkable clarity. However, the raw data collected must undergo **Signal Processing and Image Reconstruction**, sophisticated algorithms that transform the vast amounts of data into coherent images. Despite these advancements, **Challenges in Observing Black Holes** persist, including dealing with atmospheric interference and the extreme environments surrounding these cosmic phenomena. Understanding these technical insights is crucial for appreciating the monumental effort behind EHT's discoveries. By delving into these aspects, we can gain a deeper appreciation for how EHT works and its significance in modern astronomy, setting the stage for **Understanding the Basics of EHT**.
Radio Interferometry and Data Collection
Radio interferometry is a cornerstone of the Event Horizon Telescope (EHT) project, enabling scientists to achieve unprecedented resolution and detail in their observations of celestial objects. This technique leverages the principle of interference to combine signals from multiple radio telescopes, effectively creating a virtual Earth-sized telescope. Here’s how it works: each participating telescope collects radio waves emitted by a target object, such as a black hole. These signals are then recorded along with precise timing information using atomic clocks. The data from all telescopes are subsequently transported to a central location where they are synchronized and correlated, mimicking the effect of a single telescope with a diameter equal to the distance between the farthest two telescopes. The data collection process is meticulous and involves several critical steps. First, each telescope must be calibrated to ensure that the collected data are accurate and consistent. This includes adjusting for atmospheric conditions and instrumental variations. Once the data are recorded, they are transported to a central facility where they undergo a process called "correlation," where the signals from different telescopes are cross-matched to extract the interference patterns. These patterns contain valuable information about the source's structure and brightness distribution. The EHT's global network of telescopes spans across the globe, from Hawaii to Chile, allowing for continuous observation as the Earth rotates. This geographical spread maximizes the baseline lengths between telescopes, thereby enhancing the angular resolution of the combined system. The collected data are then processed using sophisticated algorithms that reconstruct the image of the observed object. For instance, in the case of observing a black hole, these algorithms help scientists visualize the shadow cast by the black hole's event horizon against the surrounding accretion disk. The sheer volume and complexity of the data necessitate advanced computational resources and sophisticated software tools. The EHT uses techniques such as Very Long Baseline Interferometry (VLBI) to handle these challenges. VLBI involves recording the raw data on media that can be transported to a central facility for processing, ensuring that the timing and phase information are preserved with high accuracy. In summary, radio interferometry and the meticulous data collection process are essential components of the EHT's technical arsenal. By synchronizing and correlating signals from multiple telescopes around the world, scientists can achieve resolutions that would be impossible with a single telescope, allowing them to study cosmic phenomena with unprecedented detail and precision. This capability has revolutionized our understanding of black holes and other extreme astrophysical objects, providing insights into the fundamental physics governing these enigmatic regions of space.
Signal Processing and Image Reconstruction
Signal processing and image reconstruction are pivotal components in the operation of the Event Horizon Telescope (EHT), enabling scientists to capture and interpret the intricate details of black holes. At the heart of EHT's functionality lies a sophisticated network of radio telescopes distributed across the globe, forming a virtual Earth-sized telescope through a technique known as Very Long Baseline Interferometry (VLBI). This method involves synchronizing the observations from each telescope to create a coherent image, akin to piecing together a puzzle. The signal processing begins with the collection of raw data from each telescope, which includes radio waves emitted by hot gas swirling around black holes. These signals are recorded on high-speed hard drives and then transported to a central location for processing. Here, sophisticated algorithms synchronize the data streams, compensating for the differences in arrival times due to the Earth's rotation and the vast distances between telescopes. This synchronization is crucial as it allows the signals to be combined coherently, enhancing the resolution and sensitivity of the observations. Once synchronized, the data undergoes a series of complex transformations to correct for atmospheric and instrumental distortions. Techniques such as phase calibration and fringe fitting are employed to ensure that the signals from different telescopes are aligned accurately, thereby maximizing the quality of the interferometric data. The processed data is then fed into advanced image reconstruction algorithms, which use computational methods like CLEAN (a deconvolution algorithm) or more recent machine learning approaches to reconstruct the image of the black hole. Image reconstruction in this context is particularly challenging due to the sparse nature of the data and the inherent noise present in the observations. The EHT uses a variety of imaging techniques, including regularized maximum likelihood methods and Bayesian inference, to produce high-fidelity images. These methods leverage prior knowledge about the expected structure of black holes and the physics governing their environments to constrain the reconstruction process, thereby enhancing the reliability and detail of the final images. The culmination of these efforts results in stunning, high-resolution images of black holes, such as the first-ever image of the supermassive black hole at the center of galaxy M87. These images not only provide visual confirmation of theoretical predictions but also offer unprecedented insights into the physics of these enigmatic objects. By pushing the boundaries of signal processing and image reconstruction, scientists using EHT continue to unravel the mysteries of black holes, contributing significantly to our understanding of the universe's most extreme environments.
Challenges in Observing Black Holes
Observing black holes is one of the most daunting challenges in astrophysics, primarily due to their nature as regions of spacetime where gravity is so strong that nothing, including light, can escape once it falls within a certain boundary known as the event horizon. This makes direct observation impossible. Several key challenges complicate the study of black holes: 1. **Invisibility**: Since no light can escape from a black hole, it does not emit or reflect any electromagnetic radiation, making it invisible to our telescopes. Scientists must rely on indirect methods to detect and study these cosmic phenomena. 2. **Gravitational Interference**: The intense gravitational field of a black hole warps spacetime around it, affecting the paths of nearby stars, gas, and even light. This gravitational lensing can distort observations and make precise measurements difficult. 3. **Distance and Scale**: Black holes are typically found at vast distances from Earth, and their event horizons are incredibly small compared to their surroundings. For instance, the supermassive black hole at the center of the Milky Way galaxy has an event horizon diameter of about 12 million kilometers, but it is located over 26,000 light-years away. 4. **Interstellar Medium**: The interstellar medium (ISM) and intergalactic medium (IGM) can absorb or scatter light and other forms of electromagnetic radiation, further complicating observations. This interference can obscure or distort signals that might otherwise provide clues about black hole activity. 5. **Technological Limitations**: Even with advanced telescopes like the Event Horizon Telescope (EHT), resolving the tiny angular scales required to observe black holes is a significant technological challenge. The EHT uses a technique called very long baseline interferometry (VLBI) to achieve high angular resolution by combining data from multiple telescopes around the world. 6. **Data Processing**: The sheer volume of data collected by such networks of telescopes necessitates sophisticated algorithms and computational resources to reconstruct images of black holes. This process involves intricate data processing and calibration to ensure accuracy. Despite these challenges, scientists have made groundbreaking progress in recent years. The EHT's ability to form a virtual Earth-sized telescope has allowed for the first-ever direct imaging of a black hole's shadow, providing unprecedented insights into these enigmatic objects. By overcoming these technical hurdles, researchers continue to unravel the mysteries surrounding black holes, enhancing our understanding of the universe's most extreme environments.
Impact and Future Directions of EHT Research
The Event Horizon Telescope (EHT) has revolutionized our understanding of the universe, particularly in the realm of black hole research. Since its inception, the EHT has made groundbreaking scientific discoveries that have significantly contributed to our knowledge of these cosmic phenomena. This article delves into the impact and future directions of EHT research, exploring three key areas: **Scientific Discoveries and Contributions**, which highlights the major breakthroughs achieved through EHT observations; **Potential for Future Observations and Studies**, which outlines the exciting possibilities for upcoming research endeavors; and **Collaborative Efforts and Global Implications**, which discusses the international cooperation and broader societal impacts of this research. By understanding these aspects, we can better appreciate the transformative power of the EHT and its role in advancing our comprehension of the universe. To fully grasp the significance of these advancements, it is essential to first **Understand the Basics of EHT**, which provides a foundational overview of this innovative technology and its operational principles.
Scientific Discoveries and Contributions
Scientific discoveries and contributions have been the cornerstone of human progress, driving innovation and transforming our understanding of the universe. The Event Horizon Telescope (EHT) project is a prime example of such groundbreaking research, showcasing the power of collaborative science in unveiling the mysteries of the cosmos. The EHT's achievement in capturing the first-ever image of a black hole in 2019 marked a significant milestone, confirming decades of theoretical predictions and opening new avenues for astrophysical research. This feat was made possible by the integration of cutting-edge technology, sophisticated algorithms, and international cooperation among scientists from diverse disciplines. The EHT's contributions extend beyond visual confirmation; they have provided invaluable insights into the physics of black holes. By observing the shadow of the supermassive black hole at the center of galaxy M87, scientists have gained a deeper understanding of general relativity, gravitational lensing, and the behavior of matter in extreme environments. These findings have sparked a flurry of research into black hole formation, accretion processes, and their role in shaping galaxy evolution. Moreover, the EHT's success has inspired the development of new observational techniques and technological advancements, such as very long baseline interferometry (VLBI), which will be crucial for future astronomical studies. Looking ahead, the impact and future directions of EHT research are vast and promising. As the EHT continues to observe and study black holes across the universe, it is expected to shed light on some of the most fundamental questions in cosmology, including the nature of dark matter and dark energy. The project's expansion to include more telescopes and advanced instrumentation will enhance its resolution and sensitivity, allowing for detailed studies of black hole environments and potentially even the detection of gravitational waves. Furthermore, the EHT's interdisciplinary approach serves as a model for future scientific endeavors, highlighting the importance of global collaboration and the integration of diverse expertise in tackling complex problems. In conclusion, the scientific discoveries and contributions of the EHT project exemplify the transformative power of scientific inquiry. By pushing the boundaries of what is thought possible, these researchers have not only expanded our knowledge but also paved the way for future breakthroughs that will continue to shape our understanding of the universe. As we move forward, the EHT's legacy will inspire new generations of scientists to explore, innovate, and uncover the secrets that lie at the heart of our cosmos.
Potential for Future Observations and Studies
The Event Horizon Telescope (EHT) has opened unprecedented avenues for future observations and studies, significantly expanding our understanding of black holes and the universe. As a collaborative effort involving a network of telescopes around the globe, the EHT has demonstrated its capability to capture high-resolution images of black hole environments, such as the shadow of the supermassive black hole at the center of M87. This achievement sets the stage for more detailed investigations into the physics of black holes, including their accretion processes, jet formation mechanisms, and the role they play in shaping galaxy evolution. Future observations with the EHT will likely focus on monitoring changes in black hole environments over time, providing insights into dynamic processes like accretion rate variations and jet activity. The inclusion of new telescopes and technological advancements, such as next-generation receivers and improved data processing algorithms, will enhance the resolution and sensitivity of EHT observations. This will allow scientists to study smaller black holes, including those in our own galaxy, the Milky Way, offering a more comprehensive view of black hole populations across different scales. Moreover, the EHT's success has sparked interest in multi-messenger astronomy, where observations from various wavelengths and even gravitational wave detectors are combined to gain a holistic understanding of astrophysical phenomena. For instance, coordinating EHT observations with those from other telescopes and LIGO/Virgo could provide a more complete picture of black hole mergers and their aftermath. This interdisciplinary approach promises to reveal new aspects of black hole physics and cosmology, potentially challenging current theories and leading to groundbreaking discoveries. Additionally, the EHT's findings have significant implications for theoretical astrophysics. By comparing observational data with simulations based on general relativity and magnetohydrodynamics, researchers can refine models of black hole behavior and test fundamental theories under extreme conditions. This interplay between observation and theory will drive advancements in our understanding of gravity, spacetime, and the intricate interactions within black hole systems. In conclusion, the potential for future observations and studies with the EHT is vast and promising. As this project continues to evolve with new technologies and collaborations, it is poised to revolutionize our knowledge of black holes and their role in the cosmos. The ongoing and future research directions of the EHT will not only deepen our scientific understanding but also inspire new generations of astronomers and physicists to explore the mysteries of the universe.
Collaborative Efforts and Global Implications
The collaborative efforts behind the Event Horizon Telescope (EHT) project exemplify the power of global scientific cooperation, with profound implications for our understanding of the universe. This international endeavor, involving over 200 researchers from more than 20 countries, has achieved what was once considered impossible: capturing the first-ever image of a black hole. The EHT's success is a testament to the synergy that arises when diverse expertise and resources are pooled together. By leveraging advanced radio telescopes around the globe, scientists have been able to form a virtual Earth-sized telescope, enabling them to observe objects that are otherwise invisible due to their immense distances and small angular sizes. The global implications of this research are multifaceted. Firstly, it has significantly advanced our knowledge of black holes, confirming key predictions of Einstein's theory of general relativity and providing new insights into these enigmatic objects. The EHT's findings have also sparked a wave of public interest in astronomy, inspiring future generations of scientists and engineers. Moreover, the technological innovations developed during this project—such as sophisticated data processing algorithms and high-precision timing systems—have far-reaching applications beyond astrophysics, potentially benefiting fields like medicine, climate science, and telecommunications. Furthermore, the EHT initiative sets a precedent for future large-scale scientific collaborations. It demonstrates that complex, ambitious projects can be successfully executed through coordinated international efforts, fostering a culture of cooperation and mutual respect among nations. This model can be replicated in other areas of research, such as climate change mitigation, pandemic response, and sustainable energy development, where global collaboration is crucial for addressing pressing global challenges. Looking ahead, the impact of EHT research will continue to grow as new observations are conducted and analyzed. Future directions include expanding the network of participating telescopes to improve resolution and sensitivity, allowing for more detailed studies of black holes and other cosmic phenomena. Additionally, integrating next-generation technologies like quantum computing and artificial intelligence will enhance data analysis capabilities, unlocking even deeper secrets of the universe. As we continue to push the boundaries of what is possible through collaborative science, we not only advance our understanding of the cosmos but also build stronger, more interconnected global communities.