What Sound Signal Means Altering To Port
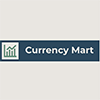
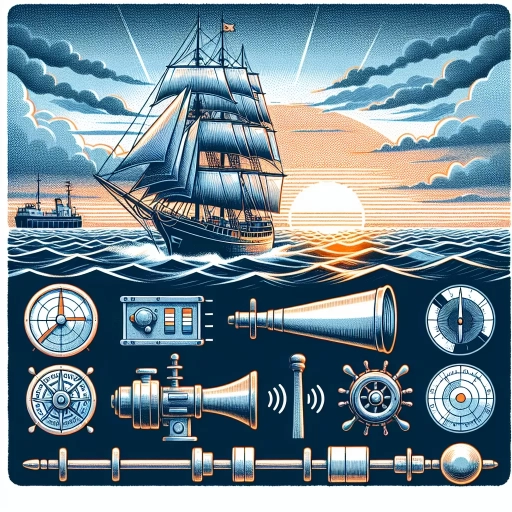
Sound signals are fundamental components of our auditory experience, conveying information and emotions through various frequencies and patterns. However, these signals can be altered to serve different purposes, enhancing or transforming their original intent. This article delves into the concept of altering sound signals, exploring three key aspects: understanding the nature of sound signals and how they can be modified, the techniques employed to alter these signals, and the applications and implications of such alterations. By grasping the underlying principles of sound signals, we can better appreciate the methods used to manipulate them. This understanding is crucial as it sets the stage for comprehending the broader impact of altered sound signals in various fields. In the following sections, we will first examine the basics of sound signals and their alteration, providing a foundational knowledge that will guide our discussion on the techniques and applications that follow. Transitioning to the next section, "Understanding Sound Signals and Their Alteration," we will explore the intricacies of sound signals and how they can be transformed.
Understanding Sound Signals and Their Alteration
Understanding sound signals and their alteration is a multifaceted topic that encompasses various aspects of acoustics and communication. At its core, this subject involves the definition of sound signals, which are vibrations that travel through a medium like air, water, or solids. These signals can be categorized into different types, each with unique characteristics and applications. For instance, there are analog and digital sound signals, each serving distinct purposes in audio technology. The importance of sound signals in communication cannot be overstated; they form the basis of verbal and non-verbal communication, enabling us to convey and interpret information effectively. By delving into these three key areas—definition, types, and importance—we can gain a comprehensive understanding of how sound signals function and how they are altered to suit various needs. To begin this exploration, it is essential to start with the fundamental concept: the definition of sound signals.
Definition of Sound Signals
**Definition of Sound Signals** Sound signals are vibrations that travel through a medium, such as air, water, or solid objects, and are perceived by the human ear or other sensory mechanisms. These signals are generated by the movement of particles in the medium, creating pressure waves that propagate outward from the source. The definition encompasses various aspects: **frequency**, which is the number of oscillations per second measured in Hertz (Hz); **amplitude**, which represents the intensity or loudness of the sound; and **wavelength**, the distance between two consecutive peaks or troughs of the wave. Sound signals can be classified into different types, including **mechanical waves** (which require a physical medium to propagate) and **electrical signals** (which are representations of sound in electronic form). Understanding these fundamental properties is crucial for comprehending how sound signals can be altered, such as through amplification, filtering, or modulation, to enhance or modify their characteristics for various applications like audio engineering, medical diagnostics, and communication systems. Altering sound signals involves manipulating these properties to achieve specific outcomes, such as improving sound quality, reducing noise, or encoding information. This manipulation can occur at different stages, from the initial generation of the sound to its final reception and interpretation by the listener. In the context of altering sound signals to "port" them—meaning to transfer or adapt them from one system or medium to another—the process often involves converting between different formats or adjusting the signal's characteristics to ensure compatibility and optimal performance. This could involve digital-to-analog conversion, frequency shifting, or adjusting the signal's amplitude to match the requirements of the target system. By understanding the definition and properties of sound signals, one can effectively alter them to meet specific needs, ensuring clear and accurate transmission and reception across various platforms.
Types of Sound Signals
Sound signals are fundamental components of auditory communication and can be categorized into several types based on their characteristics and applications. **Analog Sound Signals** are continuous waveforms that vary in amplitude and frequency over time, mimicking the natural sound waves we hear. These signals are commonly used in traditional audio systems such as vinyl records, cassette tapes, and early radio broadcasts. In contrast, **Digital Sound Signals** are discrete representations of sound, where the continuous waveform is converted into a series of numerical values. This format is used in modern audio technologies like CDs, MP3s, and digital streaming services due to its higher fidelity and resistance to degradation. **Periodic Sound Signals** repeat their waveform pattern over regular intervals, producing a consistent pitch. These signals are often used in music and speech synthesis. **Aperiodic Sound Signals**, on the other hand, do not repeat their pattern and are typically associated with noise or random sounds. **Pulse Signals** consist of short bursts of sound separated by periods of silence, commonly found in medical equipment like ultrasound machines. **Modulated Sound Signals** involve altering a carrier wave by varying its amplitude, frequency, or phase to encode information. This technique is crucial in telecommunications for transmitting audio over long distances without significant loss of quality. For instance, **Amplitude Modulation (AM)** changes the amplitude of the carrier wave according to the information signal, while **Frequency Modulation (FM)** changes the frequency. Understanding these types of sound signals is essential for altering them effectively. For example, in audio processing, engineers might use **Filtering** to remove unwanted frequencies from an analog signal or apply **Compression** to adjust the dynamic range of a digital signal. **Reverberation** and **Echo** effects can also be added to enhance the spatial perception of sound. In the context of porting sound signals across different platforms or mediums, it is crucial to consider the compatibility and integrity of the signal. For instance, converting an analog signal to digital requires careful sampling and quantization to avoid loss of detail. Similarly, when transmitting sound signals over networks, ensuring that the signal is properly modulated and encoded can prevent distortion and ensure high-quality playback. In summary, understanding the various types of sound signals—whether analog or digital, periodic or aperiodic—is vital for effective alteration and transmission. Each type has its unique characteristics and applications, and recognizing these differences allows for better manipulation and enhancement of sound in various contexts.
Importance of Sound Signals in Communication
Sound signals play a crucial role in communication, serving as a fundamental medium through which information is conveyed. In various contexts, from everyday conversations to complex technological systems, sound signals are essential for transmitting data accurately and efficiently. For instance, in human communication, speech is the primary form of sound signal that allows individuals to express thoughts, emotions, and ideas. The nuances of tone, pitch, and volume within speech provide additional layers of meaning, enhancing the richness and depth of communication. In technological realms, sound signals are vital for numerous applications. In telecommunications, voice calls rely on sound signals to connect people across distances. Similarly, in audio broadcasting, radio and television stations use sound signals to disseminate news, entertainment, and educational content to a wide audience. The integrity of these signals is paramount; any alteration or distortion can lead to miscommunication or loss of information. Moreover, sound signals are critical in safety and warning systems. Alarms and sirens, for example, use distinct sound patterns to alert people to potential dangers such as fires, natural disasters, or security breaches. These signals must be clear and recognizable to ensure prompt and appropriate responses. In scientific research and medical diagnostics, sound signals are used extensively. Ultrasound technology, for instance, employs high-frequency sound waves to create images of internal body structures, aiding in medical diagnosis. Seismologists use seismic waves (a type of sound wave) to study earthquakes and understand Earth's internal structure. The importance of sound signals extends into environmental monitoring as well. Acoustic sensors can detect changes in ecosystems by analyzing the sounds produced by wildlife, helping researchers monitor biodiversity and detect early signs of ecological disturbances. However, the alteration of sound signals can have significant consequences. In communication systems, signal degradation due to noise or interference can lead to misunderstandings or complete loss of information. In medical diagnostics, altered sound signals from ultrasound or other imaging technologies could result in misdiagnosis. Therefore, maintaining the integrity of sound signals through proper transmission, reception, and processing is crucial. In summary, sound signals are indispensable in various aspects of communication and beyond. Their accuracy and clarity are essential for effective transmission of information, ensuring safety, facilitating scientific research, and supporting medical diagnostics. Understanding how sound signals can be altered and mitigating these alterations are key to maintaining their reliability and effectiveness.
Techniques for Altering Sound Signals
In the realm of sound signal processing, several techniques are employed to alter and manipulate audio signals, enhancing their quality, clarity, and overall impact. Among these techniques, Amplitude Modulation, Frequency Modulation, and Phase Modulation stand out as fundamental methods. Amplitude Modulation involves varying the amplitude of a carrier wave in accordance with the information signal, allowing for efficient transmission and reception of audio data. Frequency Modulation, on the other hand, alters the frequency of the carrier wave to encode information, offering superior noise resistance and fidelity. Phase Modulation, a less common but equally effective method, changes the phase of the carrier wave to convey information. Each of these techniques has its unique applications and advantages, making them indispensable tools in various fields such as telecommunications, audio engineering, and broadcasting. By understanding these modulation techniques, professionals can optimize sound signals for better performance and user experience. Let's delve into the specifics of Amplitude Modulation first, exploring how it works and its practical applications.
Amplitude Modulation
Amplitude Modulation (AM) is a fundamental technique in altering sound signals, where the amplitude of a carrier wave is varied in accordance with the information signal. This method is widely used in radio broadcasting and other communication systems. Here’s how it works: the carrier wave, typically a high-frequency sinusoidal wave, has its amplitude modified by the modulating signal, which is the sound or information being transmitted. The resulting modulated wave retains the frequency of the carrier but has an amplitude that fluctuates according to the modulating signal. In practical terms, AM involves multiplying the carrier wave by the modulating signal plus a bias term to ensure that the amplitude never goes below zero. This multiplication process creates sidebands around the carrier frequency, which carry the information. The sidebands are symmetrically distributed around the carrier frequency and contain all the necessary information from the original signal. One of the key advantages of AM is its simplicity and ease of implementation. It requires minimal equipment and can be demodulated using simple circuits, making it accessible for a wide range of applications. However, AM has some drawbacks; it is susceptible to noise and interference because any external noise can alter the amplitude of the signal, leading to distortion. Additionally, AM is less efficient in terms of power usage compared to other modulation techniques like Frequency Modulation (FM), as it requires more power to achieve the same signal strength. Despite these limitations, AM remains a crucial technique in various fields. In radio broadcasting, AM is used for medium-wave transmissions due to its ability to travel long distances at night when ionospheric conditions are favorable. It is also used in aircraft communication systems because it can penetrate through clouds and other obstacles more effectively than FM signals. In summary, Amplitude Modulation is an essential method for altering sound signals by varying the amplitude of a carrier wave according to an information signal. While it offers simplicity and wide applicability, it also comes with challenges related to noise susceptibility and power efficiency. Understanding AM is crucial for appreciating how sound signals are modified and transmitted in various communication systems.
Frequency Modulation
Frequency Modulation (FM) is a technique for altering sound signals by varying the frequency of a carrier wave in accordance with the amplitude of the modulating signal. This method is widely used in radio broadcasting, telecommunications, and other applications where high-quality audio transmission is crucial. In FM, the frequency of the carrier wave changes in proportion to the amplitude of the modulating signal, while the amplitude of the carrier wave remains constant. This contrasts with Amplitude Modulation (AM), where the amplitude of the carrier wave is varied. The process of FM involves several key steps: first, the audio signal to be transmitted is used to modulate the frequency of a high-frequency carrier wave. The resulting modulated wave has a frequency that deviates from the carrier frequency by an amount proportional to the amplitude of the modulating signal. This deviation is known as the frequency deviation and is typically measured in Hertz. The modulation index, which is the ratio of the frequency deviation to the modulating frequency, determines how much the frequency deviates from its original value. FM offers several advantages over AM, including higher resistance to noise and interference, better sound quality, and greater bandwidth efficiency. Because FM signals are less susceptible to electromagnetic interference and static, they provide clearer and more stable audio transmission. Additionally, FM can support stereo broadcasting and other advanced audio formats, making it a preferred choice for high-fidelity applications. In practical terms, FM is used extensively in commercial radio broadcasting, where it allows for the transmission of high-quality audio signals over long distances without significant degradation. It is also employed in various communication systems, such as satellite communications and mobile networks, due to its robustness against interference. Technologically, FM systems involve sophisticated components such as oscillators, mixers, and demodulators. The oscillator generates the carrier wave, while the mixer combines this carrier with the modulating signal to produce the modulated wave. At the receiving end, a demodulator extracts the original audio signal from the modulated wave by detecting changes in frequency. In summary, Frequency Modulation is a powerful technique for altering sound signals that enhances audio quality and resilience against interference. Its widespread adoption in broadcasting and telecommunications underscores its importance in modern communication systems. By leveraging FM's capabilities, engineers can ensure reliable and high-quality transmission of sound signals across various mediums.
Phase Modulation
Phase modulation is a technique used to alter sound signals by modifying the phase of a carrier wave in accordance with the information signal. This method is closely related to frequency modulation (FM), as both involve changing the frequency or phase of the carrier wave to encode information. In phase modulation, the phase of the carrier wave is directly proportional to the amplitude of the modulating signal. This means that as the amplitude of the modulating signal changes, so does the phase of the carrier wave, allowing for efficient transmission and reception of audio information. To implement phase modulation, an audio signal is first converted into an electrical signal, which then modulates a high-frequency carrier wave. The phase of this carrier wave shifts in response to variations in the amplitude of the audio signal. At the receiving end, demodulation is performed to extract the original audio signal from the phase-modulated carrier wave. This process involves detecting changes in phase and converting them back into amplitude variations, thereby reconstructing the original sound. Phase modulation offers several advantages over other modulation techniques. It provides a high signal-to-noise ratio (SNR), which means that it can maintain good sound quality even in noisy environments. Additionally, phase modulation is less susceptible to interference because phase changes are more robust against noise compared to amplitude changes. However, it requires more complex circuitry for modulation and demodulation compared to amplitude modulation (AM). In practical applications, phase modulation is often used in conjunction with frequency modulation in systems like FM radio broadcasting. Here, pre-emphasis and de-emphasis circuits are used to enhance high-frequency components of the audio signal before modulation and to restore the original frequency response after demodulation. This combination ensures that high-quality audio signals are transmitted with minimal distortion. Moreover, phase modulation has found its place in digital communication systems where it is used in various forms such as Quadrature Phase Shift Keying (QPSK) and Quadrature Amplitude Modulation (QAM). These techniques are essential for modern telecommunications, enabling efficient data transmission over wireless channels. In summary, phase modulation is a powerful technique for altering sound signals by modifying the phase of a carrier wave according to an information signal. Its ability to maintain high sound quality and resist noise makes it a valuable tool in both analog and digital communication systems. Whether used alone or in combination with other modulation techniques, phase modulation plays a crucial role in ensuring reliable transmission of audio information across various media.
Applications and Implications of Altered Sound Signals
The manipulation of sound signals has far-reaching implications across various fields, transforming the way we interact with and utilize audio. In the realm of **Audio Engineering and Music Production**, altered sound signals enable artists and engineers to create unique sonic landscapes, enhancing the quality and creativity of music. This is achieved through techniques such as pitch correction, reverb, and distortion, which are essential tools in modern music production. Beyond music, **Telecommunications and Radio Broadcasting** benefit from signal processing to improve transmission quality, reduce noise, and ensure clear communication over long distances. Additionally, **Medical and Scientific Uses** of altered sound signals include diagnostic tools like ultrasound and auditory testing, which rely on precise manipulation of sound waves to gather critical data. Understanding these applications is crucial for advancing technology and improving user experiences. As we delve into the specifics, we will first explore how altered sound signals are revolutionizing **Audio Engineering and Music Production**.
Audio Engineering and Music Production
Audio engineering and music production are intricately linked disciplines that involve the manipulation and enhancement of sound signals to create high-quality audio content. At the heart of these fields is the understanding of how sound signals can be altered to achieve specific artistic, technical, or practical goals. Audio engineers use a variety of techniques such as equalization, compression, reverb, and distortion to shape the sonic characteristics of recorded or live sound. In music production, these techniques are applied to craft a cohesive and engaging musical experience. For instance, equalization allows engineers to boost or cut specific frequency ranges to balance the mix, while compression helps control dynamic range, ensuring that loud parts are not overpowering and quiet parts are audible. Reverb adds spatial depth to recordings, simulating the natural ambiance of different environments, while distortion can introduce a gritty texture or warmth to instruments. The applications of altered sound signals are vast and diverse. In live performances, audio engineers adjust sound signals in real-time to ensure optimal sound quality for the audience. This involves monitoring levels, adjusting EQ settings, and using effects like reverb and delay to enhance the overall sound. In post-production for film and television, altered sound signals play a crucial role in creating immersive audio experiences. Sound designers use techniques such as pitch-shifting and time-stretching to create unique sound effects that enhance the visual narrative. Additionally, in music production, producers often experiment with altered sound signals to create innovative sounds that set their artists apart. For example, using pitch correction tools like Auto-Tune can create distinctive vocal effects, while advanced editing software allows for precise manipulation of individual tracks within a mix. The implications of altered sound signals extend beyond the technical realm into artistic and cultural domains. The ability to manipulate sound has opened up new creative possibilities for musicians and producers, enabling them to push boundaries and explore new genres. For instance, electronic music genres such as EDM and hip-hop heavily rely on altered sound signals to create their distinctive sounds. Moreover, the accessibility of digital audio workstations (DAWs) has democratized music production, allowing artists to produce high-quality recordings without the need for expensive studio equipment. This democratization has led to a proliferation of independent artists and labels, contributing to a more diverse and vibrant musical landscape. In conclusion, the alteration of sound signals is a fundamental aspect of audio engineering and music production. These techniques not only enhance the technical quality of audio but also provide artists with powerful tools for creative expression. As technology continues to evolve, the possibilities for altering sound signals will expand further, likely leading to even more innovative applications across various industries.
Telecommunications and Radio Broadcasting
Telecommunications and radio broadcasting are pivotal sectors that rely heavily on the manipulation and transmission of sound signals. In the context of telecommunications, sound signals are altered to enhance clarity, reduce noise, and optimize data transmission. For instance, voice compression algorithms are used to reduce the bandwidth required for voice calls, allowing more simultaneous connections over the same network. Additionally, echo cancellation techniques are employed to eliminate feedback loops that can disrupt communication quality. These alterations ensure that voice communications remain clear and uninterrupted, even in environments with high levels of background noise. In radio broadcasting, sound signal alteration is crucial for maintaining broadcast quality and adhering to regulatory standards. Audio compression and limiting are used to ensure that the broadcast signal stays within the allowed dynamic range, preventing distortion and maintaining a consistent volume level. Equalization techniques are applied to adjust the frequency balance of the audio, enhancing the overall listening experience by boosting or cutting specific frequency ranges. Furthermore, noise reduction algorithms help remove unwanted hiss or hum from the signal, resulting in a cleaner and more polished broadcast. These alterations not only improve the listener's experience but also help broadcasters comply with industry standards for signal quality. The implications of these altered sound signals are multifaceted. For telecommunications, improved signal quality translates into better customer satisfaction and reduced dropped calls, which can have significant economic benefits for service providers. In radio broadcasting, high-quality audio enhances listener engagement and loyalty, which can lead to increased advertising revenue. Moreover, the technological advancements in sound signal manipulation have driven innovation in related fields such as music production and audio engineering, where artists and producers rely on sophisticated tools to create and refine their work. From a broader perspective, the ability to alter sound signals has opened up new avenues for accessibility. For example, audio processing techniques can be used to create assistive technologies like real-time speech-to-text systems or audio descriptions for visually impaired individuals. These applications underscore the transformative power of sound signal manipulation in both practical and social contexts. In summary, the alteration of sound signals in telecommunications and radio broadcasting is a critical aspect of ensuring high-quality communication and entertainment. These alterations not only enhance user experience but also drive technological innovation and have significant social implications, making them a cornerstone of modern communication systems.
Medical and Scientific Uses
Altered sound signals have numerous medical and scientific applications, significantly enhancing diagnostic accuracy, therapeutic efficacy, and research capabilities. In the medical field, altered sound signals are crucial in ultrasound technology, where high-frequency sound waves are modified to create detailed images of internal organs and tissues. This technique is invaluable for diagnosing conditions such as gallstones, liver disease, and fetal development during pregnancy. Additionally, Doppler ultrasound uses altered sound signals to measure blood flow and detect vascular diseases, aiding in the early detection of conditions like atherosclerosis. In audiology, altered sound signals are used in hearing aids and cochlear implants to enhance auditory perception for individuals with hearing impairments. These devices modify sound waves to compensate for specific types of hearing loss, improving speech recognition and overall auditory function. Furthermore, auditory brainstem response (ABR) testing employs altered sound signals to assess the integrity of the auditory pathway from the inner ear to the brainstem, helping diagnose hearing disorders in infants and adults alike. Scientifically, altered sound signals play a pivotal role in non-destructive testing and material analysis. Techniques like acoustic emission testing use altered sound waves to detect stress and defects in materials, ensuring the structural integrity of critical components in industries such as aerospace and construction. In geophysics, seismic surveys involve altering sound signals to explore subsurface structures, aiding in the discovery of mineral deposits and understanding geological formations. Moreover, altered sound signals are integral to bioacoustics research, where scientists study the vocalizations of animals to understand their behavior, habitat, and population dynamics. This field has significant implications for conservation biology and wildlife management. For instance, analyzing altered sound signals from whale vocalizations helps researchers track migration patterns and monitor population health. In neuroscience, altered sound signals are used in brain-computer interfaces (BCIs) to decode neural activity associated with auditory perception. This research has the potential to develop new treatments for neurological disorders such as tinnitus and auditory hallucinations. Additionally, psychoacoustic studies involving altered sound signals help understand human perception of sound, informing the development of more effective noise reduction technologies and audio compression algorithms. Overall, the manipulation of sound signals is a versatile tool with far-reaching implications across various medical and scientific disciplines. By altering sound waves, researchers and clinicians can gain deeper insights into biological systems, improve diagnostic tools, and develop innovative therapeutic interventions. This underscores the importance of continued research into the applications and implications of altered sound signals in advancing both medical practice and scientific knowledge.