What Is The Particle Theory Of Matter
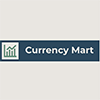
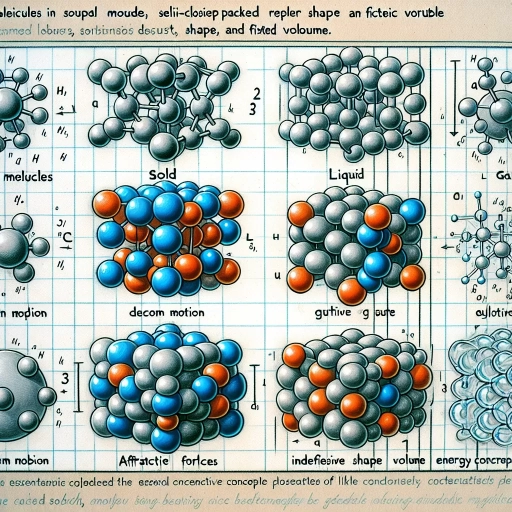
The particle theory of matter is a fundamental concept in physics that explains the nature and behavior of matter at its most basic level. This theory posits that all matter is composed of tiny, indivisible particles known as atoms and molecules, which are in constant motion. Understanding this theory is crucial for grasping various scientific principles and technological applications. In this article, we will delve into the core concepts of the particle theory, exploring its foundational ideas and how they shape our understanding of the physical world. We will also examine the wide-ranging applications and implications of this theory, from everyday phenomena to advanced scientific research. By the end of this journey, you will have a comprehensive view of how the particle theory underpins our knowledge of matter. Let us begin by introducing the particle theory of matter, laying the groundwork for a deeper exploration of its core concepts and far-reaching applications. **Introduction to the Particle Theory of Matter**
Introduction to the Particle Theory of Matter
The Particle Theory of Matter, a cornerstone of modern physics and chemistry, posits that all matter is composed of tiny, indivisible particles known as atoms and molecules. This theory has a rich historical development, tracing back to ancient philosophers such as Democritus and Epicurus, who first proposed the idea of matter being made up of tiny indivisible particles. Over centuries, this concept evolved through significant contributions from scientists like Dalton, Thomson, and Rutherford, each adding crucial layers to our understanding. The theory is built on key principles and assumptions, including the idea that atoms are the fundamental building blocks of matter, that they combine in specific ratios to form compounds, and that chemical reactions involve the rearrangement of these atoms. Today, the Particle Theory of Matter holds immense importance in modern science, underpinning fields such as chemistry, physics, and materials science. It guides our understanding of chemical reactions, the structure of materials, and the behavior of gases. In this article, we will delve into the **Introduction to the Particle Theory of Matter**, exploring its historical development, key principles and assumptions, and its significance in modern scientific endeavors.
Historical Development of the Theory
The historical development of the particle theory of matter is a rich and evolving narrative that spans thousands of years, reflecting the gradual refinement of human understanding about the fundamental nature of reality. This journey began in ancient times with philosophers such as Democritus, who around 450 BCE proposed the concept of atoms as indivisible, eternal particles that constitute all matter. This early atomic theory was largely speculative but laid the groundwork for future scientific inquiry. The idea remained dormant for centuries until the 17th and 18th centuries, when scientists like Pierre Gassendi and Antoine Lavoisier began to revive and expand upon atomic theories through empirical observations and experiments. A significant milestone was reached in the early 19th century with John Dalton's formulation of the modern atomic theory. Dalton's work, published in 1803, posited that elements are composed of small, indivisible particles called atoms and that compounds are formed when atoms of different elements combine in whole number ratios. This theory was supported by experimental evidence and marked a crucial shift from philosophical speculation to scientific validation. The late 19th and early 20th centuries saw further advancements with the discovery of subatomic particles. J.J. Thomson's discovery of electrons in 1897 revealed that atoms were not indivisible but rather composed of smaller particles. Ernest Rutherford's famous gold foil experiment in 1909 led to the development of the nuclear model of the atom, where protons reside in a central nucleus surrounded by electrons. Later, James Chadwick's discovery of neutrons in 1932 completed the basic structure of the atom as we understand it today. The particle theory of matter continued to evolve with the advent of quantum mechanics in the early 20th century. Scientists like Niels Bohr and Erwin Schrödinger introduced models that explained the behavior of electrons within atoms, further refining our understanding of atomic structure. The discovery of quarks by Murray Gell-Mann and George Zweig in the 1960s revealed that protons and neutrons are themselves composed of even smaller particles, adding another layer of complexity to the particle theory. Throughout this historical development, each breakthrough built upon previous discoveries, gradually unveiling a more detailed and accurate picture of matter at its most fundamental level. Today, the particle theory of matter is a cornerstone of physics and chemistry, guiding research in fields ranging from materials science to particle physics. This ongoing journey of discovery underscores the dynamic nature of scientific knowledge and highlights the importance of continuous inquiry into the nature of reality.
Key Principles and Assumptions
The particle theory of matter, a foundational concept in physical science, is built upon several key principles and assumptions that underpin our understanding of the structure and behavior of matter. At its core, this theory posits that all matter is composed of tiny, indivisible particles known as atoms or molecules. A crucial principle is the **Law of Conservation of Mass**, which states that matter cannot be created or destroyed in a chemical reaction, only rearranged. This law underscores the idea that the total mass of reactants equals the total mass of products. Another fundamental assumption is the **Atomic Hypothesis**, proposed by John Dalton in the early 19th century. This hypothesis asserts that elements are composed of small, indivisible particles called atoms, and that compounds are formed when atoms of different elements combine in whole number ratios. The **Law of Definite Proportions** supports this by stating that a chemical compound always contains its component elements in fixed ratios by mass, regardless of its source or method of preparation. The particle theory also relies on the concept of **Kinetic Molecular Theory**, which describes the behavior of gases. This theory assumes that gases consist of widely spaced particles (molecules or atoms) that are in constant random motion due to thermal energy. The collisions between these particles and the walls of their container explain the pressure exerted by a gas. Additionally, the theory assumes that there are no intermolecular forces between gas molecules, which simplifies the mathematical treatment of gas behavior. Furthermore, the **Quantum Mechanical Model** of the atom, developed in the 20th century, introduces the concept that electrons occupy specific energy levels or shells around the nucleus. This model assumes that electrons behave both as particles and waves, leading to a more nuanced understanding of atomic structure and chemical bonding. These principles and assumptions collectively form a robust framework for understanding the particle nature of matter. They enable scientists to predict and explain a wide range of phenomena, from the properties of gases to the formation of chemical bonds. By recognizing that matter is composed of discrete particles with specific properties and behaviors, the particle theory of matter provides a coherent and predictive model that has been instrumental in advancing our knowledge of the physical world. This foundational theory continues to guide scientific inquiry and technological innovation, underscoring its importance in modern science.
Importance in Modern Science
In the realm of modern science, the particle theory of matter stands as a cornerstone, underpinning our understanding of the fundamental nature of reality. This theory posits that all matter is composed of tiny, indivisible particles known as atoms and subatomic particles such as electrons, protons, and neutrons. The importance of this theory cannot be overstated; it has revolutionized various fields including physics, chemistry, and materials science. By recognizing that matter is not continuous but rather discrete, scientists have been able to explain a wide range of phenomena from the behavior of gases to the properties of solids and liquids. For instance, the kinetic theory of gases, which is based on the particle theory, explains how gas molecules interact and move, providing insights into thermodynamics and statistical mechanics. In chemistry, understanding the atomic structure has enabled the development of molecular models and chemical bonding theories, which are crucial for synthesizing new compounds and understanding chemical reactions. Furthermore, advancements in particle physics have led to breakthroughs in technology such as transistors, lasers, and computer chips, which are integral to modern electronics. The particle theory also forms the basis for quantum mechanics, a branch of physics that describes the behavior of particles at the atomic and subatomic level. This has opened up new avenues in fields like nanotechnology and quantum computing. Additionally, medical imaging techniques such as positron emission tomography (PET) rely on the principles derived from particle physics. The particle theory of matter thus serves as a foundational framework that not only enhances our comprehension of the microscopic world but also drives innovation across multiple disciplines, making it an indispensable component of modern scientific inquiry. Its impact is evident in both theoretical advancements and practical applications, solidifying its role as a pivotal concept in contemporary science.
Core Concepts of the Particle Theory
The Particle Theory of Matter is a fundamental concept in physics and chemistry that explains the behavior and properties of matter at its most basic level. This theory posits that all matter is composed of tiny particles, such as atoms and molecules, which interact and move in various ways. Understanding these core concepts is crucial for grasping the nature of different states of matter—solid, liquid, and gas—as well as the dynamics of particle motion and energy. In this article, we will delve into three key aspects of the Particle Theory: **Atoms and Molecules**, which will explore the building blocks of matter; **States of Matter: Solid, Liquid, Gas**, which will discuss how particles arrange themselves in different phases; and **Particle Motion and Energy**, which will examine how particles move and interact with each other. By exploring these topics, we will gain a comprehensive understanding of the Particle Theory of Matter, setting the stage for a deeper dive into its principles and applications. Transitioning seamlessly into the heart of our discussion, let us begin with an **Introduction to the Particle Theory of Matter**.
Atoms and Molecules
**Atoms and Molecules: Core Concepts of the Particle Theory** At the heart of the particle theory of matter lies the understanding of atoms and molecules, which are the fundamental building blocks of all substances. An **atom** is the smallest unit of a chemical element that retains the properties of that element. It consists of three main components: protons, neutrons, and electrons. **Protons** and **neutrons** reside in the nucleus, which is the central part of the atom, while **electrons** orbit around it in electron shells. The number of protons in an atom determines its chemical identity, making each element unique. When atoms combine, they form **molecules**. This combination occurs through chemical bonds, which can be ionic, covalent, or metallic. In an **ionic bond**, one or more electrons are transferred from one atom to another, resulting in the formation of ions with opposite charges that attract each other. **Covalent bonds** involve the sharing of electron pairs between atoms, allowing them to achieve a stable electronic configuration. **Metallic bonds** occur in metals where electrons are delocalized and move freely among a lattice of positively charged ions. The arrangement and bonding of atoms within molecules determine their physical and chemical properties. For instance, water (H₂O) is a molecule composed of two hydrogen atoms covalently bonded to a single oxygen atom. This specific arrangement gives water its unique properties such as high surface tension and boiling point, which are crucial for life on Earth. Understanding atoms and molecules is essential for comprehending various phenomena in chemistry and physics. For example, the behavior of gases can be explained by the kinetic molecular theory, which posits that gas molecules are in constant motion and collide with each other and their container walls. Similarly, the properties of solids and liquids can be understood by examining how their constituent molecules are arranged and interact. In summary, atoms and molecules are the foundational elements of matter according to the particle theory. Their structure, bonding, and interactions govern the properties of substances and underpin many natural processes. This fundamental knowledge is pivotal for advancing fields such as chemistry, materials science, and engineering, enabling us to develop new materials, technologies, and understand complex biological systems. By grasping these core concepts, we gain a deeper insight into the intricate world of matter at its most basic level.
States of Matter: Solid, Liquid, Gas
The particle theory of matter, a foundational concept in physics and chemistry, explains the behavior and properties of substances through the interactions of their constituent particles. At the heart of this theory are the three primary states of matter: solid, liquid, and gas. Each state is characterized by distinct particle arrangements and behaviors that are crucial for understanding various physical phenomena. In **solids**, particles are closely packed and have a fixed position in space. These particles vibrate slightly due to thermal energy but do not change their relative positions. This rigid structure gives solids their shape and volume, making them resistant to changes in shape when external forces are applied. The strong intermolecular forces between particles in solids result in higher melting points compared to liquids and gases. **Liquids** exhibit particles that are close together but are free to move past one another. This mobility allows liquids to take the shape of their container while retaining their volume. The intermolecular forces in liquids are weaker than those in solids, resulting in lower melting points and the ability to flow. However, these forces are still strong enough to maintain some degree of cohesion, preventing particles from spreading out freely. In **gases**, particles are widely spaced and are free to move in any direction. This freedom of movement means that gases expand to fill any container they occupy, adopting both the shape and volume of their container. The intermolecular forces between gas particles are very weak, allowing them to move rapidly and randomly. As a result, gases have neither definite shape nor definite volume under standard conditions. Understanding these states of matter through the lens of particle theory is essential for explaining various physical processes such as phase transitions (melting, boiling, condensation), diffusion, and the behavior of materials under different conditions. For instance, when a solid is heated, the increased kinetic energy of its particles can overcome the strong intermolecular forces, causing it to melt into a liquid. Similarly, cooling a gas can reduce the kinetic energy of its particles enough for them to come together and form droplets of liquid or even solidify. The particle theory also helps in predicting how substances will behave under different conditions of temperature and pressure. For example, increasing pressure on a gas can force its particles closer together, potentially causing it to liquefy or solidify if the temperature is appropriate. Conversely, reducing pressure on a liquid can allow its particles to spread out more freely, turning it into a gas. In summary, the states of matter—solid, liquid, and gas—are fundamental aspects of the particle theory of matter. Each state is defined by the arrangement and mobility of particles, which in turn determine the physical properties and behaviors of substances. This understanding is pivotal for explaining a wide range of natural phenomena and technological applications across various fields of science and engineering.
Particle Motion and Energy
**Particle Motion and Energy** At the heart of the particle theory of matter lies the concept of particle motion and its intrinsic relationship with energy. This fundamental principle underscores that all matter is composed of tiny particles, such as atoms and molecules, which are in constant motion. The kinetic energy associated with these particles is a direct result of their motion, and it varies significantly depending on the temperature and state of the substance. In solids, particles vibrate in place due to thermal energy, while in liquids, they have enough kinetic energy to slide past one another. In gases, particles are free to move randomly and rapidly, occupying the entire volume of their container. The energy of these particles is not just kinetic; it also includes potential energy, which arises from the interactions between particles. For instance, in a solid, the potential energy is high due to strong intermolecular forces that hold the particles in fixed positions. As temperature increases, kinetic energy rises, allowing particles to overcome these forces and transition from solid to liquid (melting) or from liquid to gas (vaporization). This process is governed by the laws of thermodynamics, which dictate how energy is transferred and transformed within systems. The motion of particles is also influenced by external forces such as gravity and electromagnetic forces. In a gravitational field, particles experience a downward force that affects their motion, while electromagnetic forces can cause particles to attract or repel each other. Understanding these interactions is crucial for explaining phenomena like diffusion, where particles move from areas of higher concentration to areas of lower concentration until equilibrium is reached. Moreover, the concept of particle motion and energy is pivotal in explaining various physical and chemical processes. For example, chemical reactions involve the breaking and forming of bonds between atoms, which requires specific amounts of energy. Catalysts work by lowering the activation energy needed for reactions to occur, thereby increasing the rate of reaction without altering the overall energy change. In conclusion, the motion and energy of particles form a cornerstone of the particle theory of matter. By grasping how these particles interact and move under different conditions, we can explain a wide range of natural phenomena and understand the underlying principles that govern the behavior of matter at its most fundamental level. This understanding not only enriches our knowledge of physical sciences but also has practical applications in fields such as chemistry, physics, and engineering.
Applications and Implications of the Particle Theory
The Particle Theory of Matter, a cornerstone of modern physics and chemistry, posits that all matter is composed of tiny, indivisible particles such as atoms and molecules. This fundamental concept has far-reaching implications across various scientific disciplines and technological fields. In this article, we will delve into the applications and implications of the Particle Theory, exploring its significance in three key areas. First, we will examine how the theory underpins our understanding of chemical reactions and processes, enabling us to predict and control the transformations of substances. Next, we will discuss how the Particle Theory influences our comprehension of the physical properties of materials, from the behavior of gases to the structure of solids. Finally, we will highlight the technological innovations that have been driven by this theory, transforming industries and daily life. By understanding these applications, we can appreciate the profound impact of the Particle Theory on both scientific knowledge and practical innovation, setting the stage for a deeper exploration into the Introduction to the Particle Theory of Matter.
Chemical Reactions and Processes
Chemical reactions and processes are fundamental to understanding the particle theory of matter, as they illustrate how particles interact and transform at the atomic and molecular levels. These reactions involve the breaking and forming of chemical bonds, which are essentially interactions between electrons and nuclei of atoms. According to the particle theory, matter is composed of tiny particles called atoms, which in turn are made up of even smaller particles such as protons, neutrons, and electrons. During a chemical reaction, these particles rearrange themselves to form new substances with different properties. For instance, in a combustion reaction like the burning of methane (CH₄) in oxygen (O₂), methane molecules react with oxygen molecules to produce carbon dioxide (CO₂) and water (H₂O). At the particle level, this involves the breaking of C-H and O-O bonds in the reactants and the formation of new C-O and H-O bonds in the products. This process is driven by the energy changes associated with bond formation and breaking, which can be exothermic or endothermic depending on the reaction. The implications of these processes are vast and diverse. In industrial chemistry, understanding chemical reactions allows for the synthesis of a wide range of materials from pharmaceuticals to polymers. For example, the production of ammonia (NH₃) through the Haber-Bosch process involves the reaction of nitrogen (N₂) and hydrogen (H₂) gases under high pressure and temperature, illustrating how specific conditions can be optimized to favor desired outcomes. In biological systems, chemical reactions are crucial for life processes such as metabolism, where enzymes catalyze reactions that break down nutrients to release energy or synthesize new biomolecules. The particle theory helps explain why certain enzymes are highly specific to particular substrates by detailing how the shape and charge distribution of enzyme active sites match those of their substrates at a molecular level. Furthermore, environmental science relies heavily on understanding chemical reactions to address issues like pollution and climate change. For example, the formation of acid rain involves the reaction of sulfur dioxide (SO₂) and nitrogen oxides (NOx) with water vapor in the atmosphere, leading to harmful effects on ecosystems. Knowing these mechanisms allows scientists to develop strategies for mitigating such impacts. In summary, chemical reactions and processes are integral to applying the particle theory of matter across various fields. By understanding how particles interact at the atomic and molecular levels, scientists can explain, predict, and manipulate chemical transformations that underpin both natural phenomena and human innovations. This deepens our appreciation for the intricate dance of particles that governs our world and empowers us to harness these principles for societal benefit.
Physical Properties of Materials
The physical properties of materials are fundamentally influenced by the particle theory of matter, which posits that all substances are composed of tiny, indivisible particles called atoms or molecules. These particles interact with each other through various forces, determining the macroscopic properties we observe. For instance, the density of a material is a direct result of how closely packed these particles are and their mass. In solids, particles are tightly arranged in a regular pattern, leading to higher densities compared to liquids and gases where particles have more freedom to move and spread out. The thermal conductivity of a material also depends on the arrangement and interaction of its particles; metals, with their free electrons that can easily transfer energy, are generally good conductors, while insulators like wood or plastic have particles that are more tightly bound and less able to transfer heat. The particle theory also explains other critical physical properties such as melting and boiling points. These transition temperatures are determined by the strength of the intermolecular forces between particles. Substances with strong intermolecular forces require more energy to overcome these forces, resulting in higher melting and boiling points. Conversely, substances with weaker intermolecular forces have lower transition temperatures. Additionally, the theory helps in understanding the viscosity and surface tension of liquids. Viscosity, or resistance to flow, is influenced by the strength of intermolecular forces and the size of the molecules; thicker liquids like honey have stronger forces and larger molecules compared to thinner liquids like water. Surface tension, which causes liquids to behave as if they have an "elastic skin" at their surface, arises from the imbalance of intermolecular forces at the surface relative to those within the bulk of the liquid. Furthermore, the particle theory underpins our understanding of phase changes and the behavior of gases. The ideal gas law (PV = nRT) is derived from assumptions about the behavior of gas molecules as they move freely and collide elastically with each other and their container walls. This law allows us to predict how gases will behave under different conditions of pressure, volume, and temperature. In practical applications, these principles are crucial in fields such as engineering, where materials are selected based on their specific physical properties to meet the demands of various applications. For example, in aerospace engineering, materials must withstand extreme temperatures and stresses; understanding their particle-level behavior is essential for designing safe and efficient aircraft components. In summary, the physical properties of materials—such as density, thermal conductivity, melting and boiling points, viscosity, surface tension, and phase behavior—are all rooted in the interactions and arrangements of their constituent particles as described by the particle theory of matter. This foundational understanding enables us to predict and manipulate material properties for a wide range of technological and scientific applications.
Technological Innovations Based on the Theory
Technological innovations rooted in the particle theory of matter have revolutionized various fields, transforming our understanding and interaction with the physical world. This theory posits that matter is composed of tiny, indivisible particles called atoms and molecules, which are in constant motion. By leveraging this fundamental concept, scientists and engineers have developed groundbreaking technologies that underpin modern society. One significant application is in the realm of materials science. The particle theory has enabled the creation of advanced materials with tailored properties, such as nanomaterials and composites. These materials are engineered at the atomic and molecular level to exhibit superior strength, conductivity, and durability. For instance, graphene—a single layer of carbon atoms arranged in a hexagonal lattice—has been harnessed for its exceptional electrical conductivity and mechanical strength, leading to innovations in electronics, energy storage, and biomedical devices. In the field of electronics, the particle theory forms the basis for semiconductor technology. Understanding how electrons behave at the atomic level has allowed for the development of transistors, diodes, and integrated circuits that power modern computing and communication systems. This has led to the miniaturization of electronic devices, enabling the creation of smartphones, laptops, and other portable technologies that have transformed global communication and information exchange. The particle theory also has profound implications for energy production and storage. Nuclear power plants, for example, rely on the principles of atomic reactions to generate electricity. Additionally, advancements in battery technology—such as lithium-ion batteries—have been driven by a deeper understanding of ionic interactions at the molecular level. These batteries are crucial for electric vehicles and renewable energy systems, contributing significantly to sustainable energy solutions. Furthermore, medical technology has benefited immensely from insights derived from the particle theory. Diagnostic tools like MRI (Magnetic Resonance Imaging) machines operate based on the principles of nuclear magnetic resonance, where hydrogen nuclei align in response to magnetic fields. This technology allows for detailed imaging of internal body structures without invasive procedures. Similarly, targeted drug delivery systems use nanoparticles to transport therapeutic agents directly to diseased cells, enhancing treatment efficacy while minimizing side effects. In conclusion, technological innovations grounded in the particle theory of matter have far-reaching implications across multiple disciplines. From advanced materials and electronics to energy solutions and medical diagnostics, these innovations underscore the profound impact of understanding matter at its most fundamental level. As research continues to delve deeper into the behavior of atoms and molecules, we can anticipate even more transformative technologies that will shape our future.