What Is Power Measured In
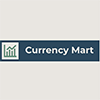
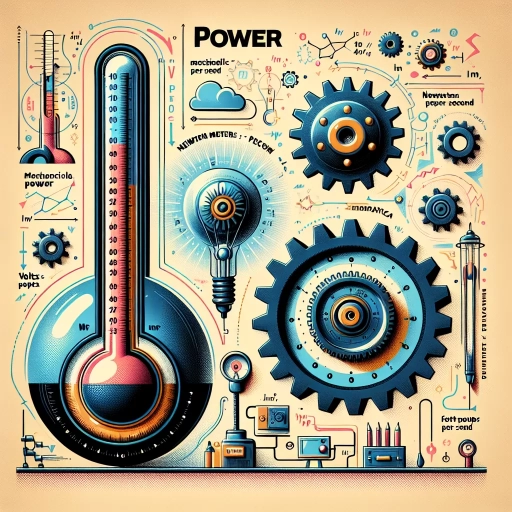
Power, a fundamental concept in physics and engineering, is a measure of the rate at which work is done or energy is transferred. Understanding power is crucial in various fields, from electrical engineering to mechanical engineering and beyond. This article delves into the intricacies of power measurement, starting with the basics of how power is defined and calculated. We will explore the units and standards that govern power measurement, highlighting the importance of consistent and accurate measurement. Additionally, we will discuss practical applications and tools used in power measurement, showcasing how these principles are applied in real-world scenarios. By grasping these foundational concepts, readers will gain a comprehensive understanding of power measurement. Let's begin by understanding the basics of power measurement.
Understanding the Basics of Power Measurement
Understanding the basics of power measurement is crucial in various aspects of science, technology, and everyday life. This fundamental concept is multifaceted, encompassing several key areas that are essential for a comprehensive grasp. First, it is important to delve into the **Definition and Units of Power**, which provides the foundational knowledge necessary to understand how power is quantified and expressed. This includes familiarizing oneself with units such as watts and horsepower, as well as the mathematical formulas that define power in terms of energy and time. Additionally, exploring the **Historical Development of Power Measurement** offers insights into how our understanding of power has evolved over time, from early mechanical measurements to modern electronic methods. Lastly, recognizing the **Importance in Various Fields** highlights the critical role power measurement plays in engineering, physics, and other disciplines, where accurate power measurements are vital for efficiency, safety, and innovation. By starting with a clear understanding of the definition and units of power, we can build a robust foundation for further exploration into its historical development and practical applications.
Definition and Units of Power
**Definition and Units of Power** Power, a fundamental concept in physics and engineering, is defined as the rate at which work is done or energy is transferred. It quantifies how quickly a system can perform tasks or deliver energy. Mathematically, power (\(P\)) is expressed as the ratio of work (\(W\)) to time (\(t\)), given by the formula \(P = \frac{W}{t}\). This definition underscores the importance of power in understanding efficiency and performance in various systems, from mechanical engines to electrical circuits. The standard unit of power in the International System of Units (SI) is the watt (W), named after James Watt, a Scottish engineer who significantly improved the steam engine. One watt is equal to one joule per second, where a joule is the unit of energy. This unit provides a universal benchmark for comparing different power sources and devices. For instance, a light bulb might consume 60 watts of power, while a high-performance computer might require several hundred watts. In addition to watts, other units of power are used for specific contexts. For example, horsepower (hp) is commonly used in automotive and mechanical engineering, particularly in the United States. One horsepower is approximately equal to 746 watts. This unit was originally defined by James Watt to help explain the power of steam engines in terms that were more relatable to the general public, who were familiar with the power of horses. In electrical engineering, power can also be expressed in terms of kilowatts (kW), megawatts (MW), and gigawatts (GW) for larger scales. These units are essential for describing the capacity of power plants, transmission lines, and other large-scale electrical systems. For instance, a small residential solar panel system might produce a few kilowatts, while a large nuclear power plant could generate several gigawatts. Understanding the units of power is crucial for designing, operating, and optimizing various systems. It allows engineers and technicians to calculate energy consumption, efficiency, and performance metrics accurately. For example, knowing the power rating of an electrical appliance helps in selecting appropriate wiring and circuit breakers to ensure safe operation. Similarly, in mechanical systems, power measurements are vital for determining the required size of motors or engines to achieve desired performance levels. In summary, power is a critical parameter that measures the rate of energy transfer or work done. The watt, as the standard unit of power, provides a consistent framework for comparing different power sources and devices across various fields. Understanding these definitions and units is essential for effective design, operation, and optimization of systems in engineering and technology.
Historical Development of Power Measurement
The historical development of power measurement is a narrative of continuous innovation and refinement, driven by the evolving needs of science, technology, and industry. The concept of power, as a measure of the rate at which work is done or energy is transferred, has its roots in ancient civilizations. However, the systematic measurement of power began to take shape during the Industrial Revolution. In the late 18th century, James Watt, a Scottish engineer, introduced the term "horsepower" as a way to describe the power output of steam engines relative to the power of horses. This was a crucial step in standardizing power measurement, allowing for more accurate comparisons between different machines. Watt's work laid the foundation for later developments in power measurement. The 19th century saw significant advancements with the discovery of electrical principles by scientists such as Michael Faraday and James Clerk Maxwell. The introduction of electrical power systems necessitated new methods for measuring power. In 1886, Nikola Tesla and George Westinghouse developed the alternating current (AC) system, which required more sophisticated measurement techniques compared to direct current (DC). The invention of the wattmeter by J.A. Aron in 1888 marked a pivotal moment in power measurement. The wattmeter allowed for direct measurement of electrical power, enabling engineers to monitor and optimize power systems with greater precision. This innovation was followed by the development of various types of wattmeters, including those designed for AC systems. In the 20th century, advancements in electronics and computing further refined power measurement techniques. The introduction of digital multimeters and power analyzers enabled more accurate and detailed measurements of power quality, including parameters such as voltage, current, frequency, and phase angle. These tools are essential in modern electrical engineering for ensuring the efficiency and reliability of power systems. Today, power measurement is a highly specialized field with a wide range of applications from industrial automation to renewable energy systems. Advanced technologies such as smart meters and IoT devices provide real-time data on power consumption and generation, facilitating better energy management and optimization. The historical development of power measurement reflects a continuous quest for precision and efficiency, underpinning many of the technological advancements that shape our world today. Understanding these historical developments is crucial for grasping the basics of power measurement. It highlights how different eras and technological breakthroughs have contributed to our current ability to measure and manage power effectively. This knowledge serves as a foundation for further exploration into the intricacies of power measurement and its applications across various fields.
Importance in Various Fields
Power measurement is crucial across various fields due to its direct impact on efficiency, safety, and performance. In **engineering**, accurate power measurement is essential for designing and optimizing systems, whether it's electrical, mechanical, or thermal. Engineers rely on precise power measurements to ensure that systems operate within specified parameters, reducing the risk of overheating, overloading, or underperformance. This is particularly critical in the development of high-performance technologies such as electric vehicles and renewable energy systems. In **medicine**, power measurement plays a vital role in medical imaging and diagnostic equipment. For instance, ultrasound machines and MRI scanners require precise power control to generate accurate images and ensure patient safety. Incorrect power levels can lead to distorted images or even harm patients, making reliable power measurement indispensable. In **industrial settings**, power measurement is key to energy management and cost control. Companies use power meters to monitor energy consumption in real-time, helping them identify areas of inefficiency and implement cost-saving measures. This not only reduces operational costs but also contributes to environmental sustainability by minimizing energy waste. In **research and development**, power measurement is fundamental for scientific experiments and data collection. Scientists need to measure power accurately to understand the behavior of materials, devices, and systems under various conditions. This is particularly important in fields like materials science and nanotechnology where small variations in power can significantly affect experimental outcomes. In **consumer electronics**, power measurement ensures that devices operate safely and efficiently. Manufacturers must adhere to strict standards for power consumption to prevent overheating, electrical shocks, and other safety hazards. This is especially relevant for battery-powered devices where incorrect power management can lead to reduced battery life or even device failure. In **aviation and aerospace**, precise power measurement is critical for the safe operation of aircraft and spacecraft. Power systems in these vehicles must be meticulously monitored to ensure reliable performance under extreme conditions. Any malfunction or miscalculation in power measurement could have catastrophic consequences. In **environmental monitoring**, power measurement helps in assessing the impact of human activities on the environment. For example, measuring the power output of renewable energy sources like wind turbines and solar panels helps in evaluating their effectiveness and potential for widespread adoption. Overall, the importance of power measurement cannot be overstated. It underpins the efficiency, safety, and reliability of systems across diverse fields, making it a fundamental aspect of modern technology and innovation. Understanding the basics of power measurement is thus essential for anyone involved in these areas, as it provides the foundation for optimizing performance and ensuring compliance with safety standards.
Units and Standards for Measuring Power
Measuring power is a fundamental aspect of various scientific and engineering disciplines, and understanding the units and standards involved is crucial for accurate and meaningful communication. This article delves into the essential concepts of power measurement, focusing on three key areas: Watt and Derived Units, the International System of Units (SI), and Conversion Between Different Units. Firstly, we explore Watt and Derived Units, which are the foundational measures of power in the SI system. The watt, named after James Watt, is the standard unit of power and is widely used across different fields. Understanding derived units such as kilowatts and megawatts is also vital for practical applications. Secondly, the International System of Units (SI) provides a coherent framework for measuring power. The SI system ensures consistency and precision in scientific and technical measurements, making it an indispensable tool for global communication and collaboration. Lastly, Conversion Between Different Units is essential for comparing and interpreting data from various sources. This section will guide readers through the process of converting between different power units, ensuring that they can seamlessly navigate between different measurement systems. By examining these three critical aspects, this article aims to provide a comprehensive understanding of power measurement, starting with the foundational concept of Watt and Derived Units.
Watt and Derived Units
In the realm of measuring power, the Watt (W) stands as a fundamental unit, derived from the International System of Units (SI). Named after James Watt, the Scottish engineer who improved the steam engine, this unit quantifies the rate at which work is done or energy is transferred. A Watt is defined as one joule per second, symbolizing the power required to perform one joule of work in one second. This definition underscores its role in measuring both mechanical and electrical power. Derived units of the Watt are essential for various applications across different scales. For instance, the kilowatt (kW), which equals 1,000 Watts, is commonly used to measure the power output of household appliances and industrial machinery. The megawatt (MW), representing 1 million Watts, is often employed in describing the capacity of power plants and large-scale energy systems. On the other end of the spectrum, smaller units like milliwatts (mW) and microwatts (μW) are used in electronics and telecommunications to measure low levels of power consumption. The Watt also forms the basis for other derived units that measure related quantities. For example, the watt-hour (Wh) or kilowatt-hour (kWh) measures energy consumption over time, where one kWh equals 1,000 Watts used over one hour. This unit is crucial for billing electricity usage and evaluating the efficiency of energy storage systems. In scientific and engineering contexts, understanding these derived units is vital for accurate calculations and comparisons. For instance, in thermodynamics, the Watt can be related to other units such as the British Thermal Unit (BTU) or the calorie, allowing for cross-disciplinary analysis. In electrical engineering, knowing how to convert between Watts and other power units like volts and amperes (using Ohm's law) is essential for designing circuits and systems. Moreover, the Watt has practical implications in everyday life. It helps consumers make informed decisions about energy efficiency when purchasing appliances or vehicles. For example, a refrigerator with a lower Watt rating indicates lower energy consumption, which can lead to cost savings over time. In summary, the Watt and its derived units are indispensable in measuring power across various domains. From industrial power plants to household electronics, these units provide a standardized framework for quantifying energy transfer and consumption. Their precise definitions and applications ensure that engineers, scientists, and consumers alike can accurately assess and manage power resources effectively.
International System of Units (SI)
The International System of Units (SI) is the global standard for measurement, ensuring uniformity and precision across various scientific, technical, and everyday applications. Established by the 11th General Conference on Weights and Measures in 1960, SI is based on seven fundamental units: meter (length), kilogram (mass), second (time), kelvin (temperature), ampere (electric current), mole (amount of substance), and candela (luminous intensity). These base units are used to derive a wide range of derived units, such as the watt for power, which is defined as one joule per second. The SI system's coherence and logical structure make it an indispensable tool for scientific research, engineering, and international trade. For measuring power, the watt is the SI unit, representing the rate at which work is done or energy is transferred. This unit is crucial in fields like electrical engineering, where it quantifies the power consumption or output of devices and systems. The use of SI units ensures that measurements are consistent and comparable worldwide, facilitating collaboration and innovation across borders. By adhering to the SI system, scientists and engineers can communicate effectively, conduct precise calculations, and develop technologies with global applicability. In summary, the International System of Units provides a robust framework for measuring power and other physical quantities, underpinning modern science and technology with its clarity, consistency, and universality.
Conversion Between Different Units
Conversion between different units is a crucial aspect of measuring power, as it allows for the comparison and communication of power values across various systems and applications. Power, typically measured in watts (W), can be expressed in several other units depending on the context. For instance, in electrical engineering, power is often measured in kilowatts (kW) or megawatts (MW) for larger scales. To convert between these units, one simply multiplies or divides by the appropriate factor: 1 kW = 1,000 W and 1 MW = 1,000,000 W. In mechanical engineering, power might be measured in horsepower (hp), which is particularly common in automotive and industrial contexts. The conversion from horsepower to watts involves knowing that 1 hp is approximately equal to 746 W. This conversion is essential for comparing the performance of engines or motors across different specifications. In scientific and technical contexts, power can also be expressed in joules per second (J/s), which is equivalent to watts. This unit is useful when discussing energy transfer rates over time. For example, if an electrical device consumes 100 joules of energy in one second, it operates at a power of 100 watts. Additionally, in some specialized fields like nuclear power or high-energy physics, power might be expressed in gigawatts (GW) or even terawatts (TW). These larger units simplify the representation of enormous power outputs, such as those produced by nuclear reactors or high-energy particle accelerators. Understanding these conversions is vital for accurate communication and calculation in various fields. For instance, an engineer designing a power grid needs to convert between megawatts and gigawatts to ensure that the system can handle the total load efficiently. Similarly, a physicist studying high-energy phenomena must be able to convert between joules per second and terawatts to understand the scale of energy release. In summary, the ability to convert between different units of power is fundamental to ensuring precision and clarity in technical and scientific work. Whether dealing with small-scale electronics or large-scale industrial applications, knowing how to convert watts to kilowatts, horsepower, joules per second, or even gigawatts and terawatts is essential for accurate measurement and effective communication of power values. This versatility in unit conversion enhances the understanding and application of power measurements across diverse disciplines.
Practical Applications and Tools for Power Measurement
In the realm of power measurement, precision and accuracy are paramount for efficient operation and safety across various industries. This article delves into the practical applications and tools that facilitate reliable power measurement, highlighting three key areas: Electrical Power Measurement Devices, Mechanical Power Measurement Techniques, and Advanced Technologies in Power Measurement. Electrical power measurement devices are crucial for monitoring and controlling electrical systems, ensuring optimal performance and minimizing energy waste. Mechanical power measurement techniques, on the other hand, focus on the physical aspects of power transmission, providing insights into system efficiency and potential areas for improvement. Advanced technologies in power measurement leverage cutting-edge innovations such as IoT sensors and AI analytics to enhance data accuracy and real-time monitoring capabilities. By exploring these facets, this article aims to provide a comprehensive understanding of the tools and methods available for accurate power measurement. We begin by examining the fundamental role of Electrical Power Measurement Devices in modern power management systems.
Electrical Power Measurement Devices
Electrical power measurement devices are crucial tools in various fields, including electrical engineering, industrial automation, and energy management. These devices are designed to accurately measure the amount of electrical power being consumed or generated in a system. Here are some key types and their practical applications: 1. **Wattmeters**: These devices measure the real power (in watts) being used by an electrical circuit. Wattmeters are essential in industrial settings to monitor energy consumption and ensure efficient use of power. 2. **Multimeters**: While primarily used for measuring voltage, current, and resistance, some advanced multimeters also offer power measurement capabilities. They are versatile and widely used in both professional and DIY contexts. 3. **Power Analyzers**: These sophisticated instruments provide detailed measurements of various power parameters such as real power, reactive power, apparent power, and power factor. Power analyzers are invaluable in research and development, quality control, and troubleshooting complex electrical systems. 4. **Smart Meters**: These modern devices not only measure electrical power but also communicate the data to utilities or other monitoring systems. Smart meters enable real-time monitoring of energy usage, facilitating better energy management and billing accuracy. 5. **Current Transformers (CTs) and Potential Transformers (PTs)**: These are used in conjunction with other measurement devices to measure high currents and voltages safely and accurately. CTs and PTs are critical components in power distribution systems. 6. **Power Quality Analyzers**: These devices assess the quality of electrical power by measuring parameters such as voltage sag, swell, harmonics, and flicker. Power quality analyzers help in identifying issues that could affect equipment performance or lifespan. 7. **Energy Meters**: These meters measure the total energy consumed over a period of time (in kilowatt-hours or kWh). Energy meters are commonly used in residential and commercial settings for billing purposes. In practical applications, these devices support various functions: - **Energy Efficiency**: By accurately measuring power consumption, these devices help in identifying areas where energy can be saved. - **System Monitoring**: Continuous monitoring ensures that electrical systems operate within safe limits, reducing the risk of overloads or failures. - **Troubleshooting**: Detailed measurements from power analyzers and multimeters aid in diagnosing electrical issues quickly. - **Billing and Cost Management**: Smart meters and energy meters provide precise data for accurate billing and cost management. - **Research and Development**: Advanced power measurement devices are essential for testing new electrical systems and components. In summary, electrical power measurement devices are indispensable for ensuring the efficient, safe, and reliable operation of electrical systems across different sectors. Their ability to provide accurate and detailed measurements makes them critical tools for both everyday monitoring and complex technical applications.
Mechanical Power Measurement Techniques
Mechanical power measurement techniques are crucial for assessing the performance and efficiency of mechanical systems, ensuring they operate within optimal parameters. These techniques involve various methods to quantify the power output or input of mechanical devices such as engines, motors, and gearboxes. One common method is the use of dynamometers, which measure torque and rotational speed to calculate power. For instance, a hydraulic dynamometer can absorb the power output of an engine and convert it into a measurable form, while an eddy current dynamometer uses electromagnetic principles to provide precise readings. Another technique involves the use of strain gauges and load cells to measure forces and torques directly. These sensors can be integrated into the mechanical system to provide real-time data on power consumption or generation. In addition, tachometers are used to measure rotational speed, which, when combined with torque measurements, allows for accurate power calculations. Advanced tools like data acquisition systems and software enable the collection and analysis of data from multiple sensors simultaneously, providing comprehensive insights into system performance. In practical applications, these techniques are essential for optimizing engine performance in automotive and aerospace industries. For example, in engine testing, dynamometers help engineers fine-tune engine settings to achieve maximum efficiency and power output. In industrial settings, power measurement is critical for monitoring and maintaining machinery health, predicting maintenance needs, and ensuring compliance with safety standards. Furthermore, in renewable energy systems such as wind turbines and hydroelectric power plants, accurate power measurement is vital for optimizing energy production and grid integration. The choice of measurement technique depends on the specific application and the type of mechanical system being evaluated. For high-precision applications, laser-based methods like laser Doppler velocimetry can measure very small changes in speed and position. In contrast, simpler methods such as using a Prony brake or a rope brake may suffice for less demanding applications where cost and simplicity are key considerations. Overall, mechanical power measurement techniques are indispensable tools in engineering and industry, enabling the precise assessment and optimization of mechanical systems. By leveraging these methods, engineers can ensure that machines operate efficiently, safely, and reliably, which is critical for both economic and environmental sustainability.
Advanced Technologies in Power Measurement
Advanced technologies in power measurement have revolutionized the way energy is monitored, managed, and optimized across various industries. At the forefront of these advancements are smart meters and IoT (Internet of Things) devices, which enable real-time monitoring and data analytics. Smart meters, for instance, provide detailed insights into energy consumption patterns, allowing utilities to implement dynamic pricing models and consumers to make informed decisions about their energy usage. These meters also facilitate remote meter reading, reducing the need for physical inspections and enhancing operational efficiency. Another significant development is the integration of artificial intelligence (AI) and machine learning (ML) algorithms in power measurement systems. AI-driven tools can predict energy demand, detect anomalies in power consumption, and optimize grid operations. For example, predictive analytics can help utilities anticipate peak demand periods, ensuring that the grid remains stable and resilient. Additionally, AI-powered sensors can identify potential faults in the system before they occur, reducing downtime and improving overall reliability. The advent of advanced sensor technologies, such as Hall effect sensors and Rogowski coils, has also enhanced the accuracy and reliability of power measurements. These sensors offer high precision and robustness, making them ideal for applications in industrial settings where accurate power monitoring is critical. Furthermore, the use of wireless communication protocols like Zigbee and Wi-Fi has simplified the deployment of these sensors, enabling seamless integration into existing infrastructure. In the realm of renewable energy, advanced technologies are playing a crucial role in optimizing power output from solar and wind installations. Advanced inverters equipped with real-time monitoring capabilities ensure that these systems operate at maximum efficiency. Moreover, technologies like power quality analyzers help maintain the stability of the grid by monitoring voltage, current, and frequency parameters, ensuring that the integration of renewable sources does not compromise grid reliability. The practical applications of these technologies extend beyond utility companies to industries such as manufacturing, healthcare, and data centers. In manufacturing, precise power measurement helps in optimizing production processes, reducing energy waste, and improving product quality. In healthcare, accurate power monitoring is essential for ensuring the continuous operation of critical medical equipment. Data centers, which are among the largest consumers of electricity, rely on advanced power measurement tools to optimize cooling systems and reduce energy consumption. In conclusion, advanced technologies in power measurement are transforming the way energy is managed and utilized. From smart meters and AI-driven analytics to advanced sensors and renewable energy optimization, these innovations are driving efficiency, reliability, and sustainability across diverse sectors. As these technologies continue to evolve, they will play an increasingly vital role in shaping a more energy-efficient future.