What Is A Singlet
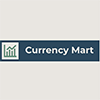
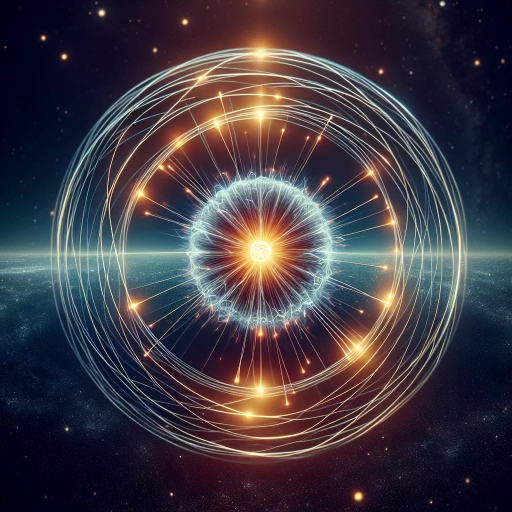
In the realm of quantum mechanics and materials science, the term "singlet" holds significant importance, yet it remains a concept often shrouded in mystery for those outside these fields. A singlet, in its most basic form, refers to a state where two particles, such as electrons or spins, are paired in such a way that their total spin is zero. This unique pairing has profound implications across various scientific disciplines. To fully grasp the essence of a singlet, it is crucial to delve into three key aspects: understanding the concept itself, exploring the underlying science, and examining its applications and significance. By starting with **Understanding the Concept of a Singlet**, we will lay the groundwork for a comprehensive exploration that will subsequently uncover **The Science Behind Singlets** and ultimately reveal **Applications and Significance of Singlets**. This journey will not only demystify the singlet but also highlight its pivotal role in advancing our knowledge and technological capabilities. Let us begin by unraveling the fundamental principles that define a singlet.
Understanding the Concept of a Singlet
Understanding the concept of a singlet is a multifaceted journey that delves into its definition and origin, historical context and evolution, and addresses common misconceptions. At its core, a singlet is a fundamental concept in quantum mechanics that describes the state of a particle with zero total spin. To fully grasp this idea, it is essential to explore its definition and origin, tracing back to the early days of quantum theory when scientists like Wolfgang Pauli and Werner Heisenberg laid the groundwork. This foundational understanding sets the stage for examining the historical context and evolution of the singlet, highlighting how it has been refined and applied across various fields over time. Additionally, clarifying common misconceptions about singlets is crucial, as these misunderstandings can obscure the true nature and significance of this quantum state. By navigating these three key aspects, we can gain a comprehensive and accurate understanding of what a singlet truly represents. Let us begin by delving into the definition and origin of this pivotal concept.
Definition and Origin
The concept of a singlet, a fundamental term in various scientific and everyday contexts, has its roots in distinct origins depending on the field of application. In physics, particularly quantum mechanics, a singlet refers to a state where two particles are paired in such a way that their total spin is zero. This concept is crucial for understanding phenomena like superconductivity and the behavior of magnetic materials. The term "singlet" in this context was coined by physicists to describe the unique spin configuration that distinguishes it from other states like triplets, where the total spin is non-zero. In chemistry, a singlet state pertains to the electronic configuration of molecules. Here, it describes a situation where all electrons are paired, leading to no net spin. This is in contrast to triplet states, where two unpaired electrons have parallel spins. The singlet state is often the ground state of molecules and plays a significant role in understanding chemical reactivity and spectroscopy. Beyond these scientific definitions, the term "singlet" also has a common usage in sports and fashion. In athletics, particularly wrestling and gymnastics, a singlet is a tight-fitting, sleeveless garment worn by competitors. This usage dates back to the early 20th century when athletes sought functional clothing that allowed for maximum mobility without hindrance. The design has evolved over time but remains a staple in these sports. In fashion, the term can refer to a type of women's undergarment or a one-piece swimsuit. This usage is more recent and reflects the evolution of clothing design towards more streamlined and functional pieces. Understanding the concept of a singlet across these diverse fields highlights the versatility of the term and its importance in different contexts. Whether it is describing subatomic particles, molecular states, athletic wear, or fashion items, the essence of a singlet lies in its unique characteristics that set it apart from other configurations or garments. This multifaceted nature underscores the richness and complexity of language, where a single term can encapsulate a wide range of meanings depending on the domain of application. By grasping these definitions and origins, one can appreciate the depth and breadth of human knowledge and innovation that underpin our understanding of the world around us.
Historical Context and Evolution
The concept of a singlet, a fundamental unit in quantum mechanics and particle physics, has its roots deeply embedded in the historical context and evolutionary journey of scientific understanding. To fully grasp the significance of a singlet, it is essential to delve into the historical backdrop that shaped our current comprehension. In the early 20th century, the dawn of quantum mechanics revolutionized the way scientists perceived the atomic and subatomic world. Max Planck's introduction of the quantum hypothesis in 1900 marked the beginning of this era, followed by Albert Einstein's explanation of the photoelectric effect in 1905. However, it was Niels Bohr's atomic model in 1913 that laid the groundwork for understanding atomic structure and electron behavior. Bohr's model, though rudimentary by today's standards, introduced the concept of discrete energy levels and electron spin, which are crucial for understanding singlets. The 1920s saw a flurry of activity with the development of wave mechanics by Erwin Schrödinger and matrix mechanics by Werner Heisenberg. These theories provided a more comprehensive framework for understanding quantum systems but still lacked a complete description of electron interactions. It wasn't until the introduction of quantum field theory (QFT) in the 1930s and 1940s that physicists began to understand how particles interact at a fundamental level. QFT, developed by physicists such as Paul Dirac, Julian Schwinger, and Richard Feynman, introduced the concept of particles as excitations of underlying fields, which is pivotal for understanding singlet states. In the context of quantum mechanics, a singlet refers to a state where two or more particles have their spins aligned in such a way that their total spin is zero. This concept became clearer with the development of quantum statistics and the distinction between bosons and fermions. The Pauli exclusion principle, formulated by Wolfgang Pauli in 1925, explained how fermions (particles with half-integer spin) behave in multi-particle systems, leading to a deeper understanding of singlet states. The evolution of our understanding of singlets continued with advancements in nuclear physics and the discovery of new particles. The discovery of antiparticles by Paul Dirac in 1928 and the subsequent experimental verification by Carl Anderson in 1932 expanded our understanding of particle interactions. Later, the development of quantum chromodynamics (QCD) in the 1970s provided a detailed theory of strong interactions, further elucidating how quarks and gluons interact within hadrons, which often form singlet states. In modern physics, singlets play a critical role in various fields, including condensed matter physics, where they are used to describe exotic states in superconductors and superfluids. In particle physics, singlet states are crucial for understanding the behavior of quarks within hadrons and the properties of Higgs bosons. The Higgs boson itself, discovered in 2012 at CERN, is a scalar boson that exists as a singlet under the electroweak symmetry group, highlighting the importance of singlet states in fundamental theories. In conclusion, the concept of a singlet is deeply intertwined with the historical evolution of quantum mechanics and particle physics. From the early models of atomic structure to the sophisticated theories of quantum field theory and beyond, each milestone has contributed to our current understanding of singlet states. This historical context not only enriches our comprehension but also underscores the dynamic nature of scientific inquiry, where each discovery builds upon the foundations laid by predecessors.
Common Misconceptions
When delving into the concept of a singlet, it is crucial to address common misconceptions that often cloud understanding. One prevalent misconception is that a singlet refers exclusively to a type of athletic wear, such as a sleeveless shirt. While this is indeed a common usage, the term "singlet" has a broader and more nuanced meaning in various contexts. In physics, particularly quantum mechanics, a singlet state describes a system where two particles are coupled in such a way that their total spin is zero. This concept is fundamental in understanding phenomena like quantum entanglement and spin dynamics. Another misconception arises from the assumption that singlets are always related to pairs or dualities; however, in chemistry and materials science, a singlet state can refer to an electronic state where all electrons are paired, leading to no net spin. This distinction is vital for understanding molecular properties and reactivity. Additionally, some people mistakenly believe that singlets are inherently stable or simple; however, singlet states can be highly sensitive to environmental conditions and require precise control to maintain. For instance, in the field of quantum computing, maintaining a singlet state is crucial for quantum gate operations but is also notoriously challenging due to decoherence. By clarifying these misconceptions, one can gain a deeper appreciation for the multifaceted nature of singlets across different scientific disciplines and avoid confusion that might arise from oversimplified or incorrect interpretations. Understanding these nuances not only enriches one's knowledge but also highlights the intricate and fascinating world of singlets beyond their everyday connotations.
The Science Behind Singlets
The science behind singlets, a fundamental concept in quantum mechanics and materials science, is multifaceted and intriguing. To fully understand the properties and behaviors of singlets, it is essential to delve into three key areas: Quantum Mechanics and Spin States, Molecular Structure and Bonding, and Energy Levels and Transitions. Quantum Mechanics and Spin States provide the foundational framework for understanding how singlets arise from the interaction of electron spins. This realm explains how paired electrons in a molecule can align their spins in a way that results in a singlet state, characterized by zero net spin. Molecular Structure and Bonding shed light on how the arrangement of atoms within a molecule influences the formation of singlets. The bonding patterns and molecular geometry play crucial roles in determining the electronic configuration and thus the possibility of singlet states. Energy Levels and Transitions reveal the dynamic aspect of singlets, explaining how these states can be achieved or altered through various energy transitions. This includes understanding the absorption and emission spectra that are indicative of these transitions. By exploring these interconnected concepts, we can gain a comprehensive understanding of the science behind singlets. Let us begin by examining the role of Quantum Mechanics and Spin States in the formation of these unique electronic configurations.
Quantum Mechanics and Spin States
Quantum Mechanics and Spin States are fundamental concepts that underpin the understanding of singlets, a term often used in various scientific contexts. At its core, Quantum Mechanics is a branch of physics that describes the behavior of matter and energy at an atomic and subatomic level. Here, particles such as electrons, protons, and neutrons exhibit properties that defy classical physics, including wave-particle duality and superposition. One of the key aspects of Quantum Mechanics is the concept of spin states. Spin is an intrinsic property of particles, akin to angular momentum but distinct from it. It is a measure of the intrinsic angular momentum carried by particles like electrons and protons. Unlike classical angular momentum, which can take on any value, spin is quantized, meaning it can only assume specific discrete values. For electrons, the spin can be either +1/2 or -1/2, often referred to as "up" and "down" spin states, respectively. This quantization is crucial because it leads to the Pauli Exclusion Principle, which states that no two electrons in an atom can have the same set of quantum numbers, including spin state. In the context of singlets, particularly in chemistry and materials science, spin states play a pivotal role. A singlet state refers to a situation where two electrons are paired such that their spins are antiparallel (one up and one down), resulting in a total spin of zero. This pairing is stable and often preferred due to the lower energy state it occupies compared to unpaired electrons. The singlet state is crucial in understanding molecular bonding, especially in organic chemistry where it explains the stability of many molecules. Moreover, the concept of spin states extends beyond chemistry into fields like quantum computing and magnetic resonance imaging (MRI). In quantum computing, spin states are used as qubits—the fundamental units of quantum information—due to their ability to exist in superposition (both up and down simultaneously) and entanglement (correlated states). In MRI, the alignment of nuclear spins in a magnetic field allows for detailed imaging of internal structures within the body. The study of spin states also delves into the realm of quantum entanglement, where two or more particles become correlated in such a way that the state of one particle cannot be described independently of the others. This phenomenon has profound implications for our understanding of quantum systems and has been experimentally verified numerous times. In summary, the principles of Quantum Mechanics, particularly the concept of spin states, are essential for understanding the behavior of particles at the atomic and subatomic level. The singlet state, characterized by paired electrons with antiparallel spins, is a fundamental aspect of molecular stability and has far-reaching implications across various scientific disciplines. By grasping these concepts, we gain deeper insights into the intricate world of quantum phenomena and their applications in modern science and technology.
Molecular Structure and Bonding
Molecular structure and bonding are fundamental concepts in understanding the properties and behaviors of molecules, including those that form the basis of singlets. At its core, molecular structure refers to the arrangement of atoms within a molecule, while bonding describes the forces that hold these atoms together. The primary types of chemical bonds are ionic, covalent, and metallic, each resulting from different interactions between electrons and nuclei. In covalent bonds, which are particularly relevant to organic molecules like those found in singlets, atoms share one or more pairs of electrons to achieve a stable electronic configuration. This sharing can be symmetrical (as in single bonds) or involve multiple pairs (as in double and triple bonds), influencing the molecule's shape and reactivity. The shape of a molecule, determined by the spatial arrangement of its atoms, is crucial for understanding its physical and chemical properties. For instance, the tetrahedral shape of methane (CH₄) and the planar shape of ethene (C₂H₄) are direct consequences of their covalent bonding patterns. The strength and type of covalent bonds also play a significant role in determining the durability and flexibility of materials. In the context of singlets, which are typically made from synthetic fibers like polyester or nylon, the covalent bonds within these polymers contribute to their strength, elasticity, and resistance to wear. Polyester, for example, is composed of long chains of repeating units linked by strong covalent bonds, making it a durable fabric suitable for everyday wear. Moreover, the molecular structure influences the thermal properties of materials. The arrangement of atoms and the nature of their bonds affect how molecules absorb and release heat, which is important for understanding how fabrics like those in singlets behave under different conditions. For instance, the high melting point of polyester is a result of its robust covalent bonds, ensuring that it retains its shape even when exposed to moderate heat. In addition to covalent bonds, intermolecular forces such as van der Waals forces and hydrogen bonds also play a crucial role in the overall properties of materials. These forces determine how molecules interact with each other, influencing characteristics like softness, breathability, and moisture-wicking ability—key factors in the comfort and performance of clothing items like singlets. Understanding molecular structure and bonding is essential for designing and manufacturing fabrics with specific properties. By manipulating the types of bonds and the arrangement of atoms within polymer chains, scientists can create materials that are tailored to meet particular needs—whether it's for athletic wear requiring moisture-wicking properties or casual wear needing durability and comfort. This scientific foundation underpins the development of high-quality singlets that combine functionality with comfort, making them an integral part of modern apparel.
Energy Levels and Transitions
Energy levels and transitions are fundamental concepts in understanding the behavior of atoms and molecules, particularly relevant when discussing the science behind singlets. In atomic physics, energy levels refer to the quantized states that electrons can occupy around an atom. These levels are discrete and correspond to specific energies, which are determined by the principal quantum number (n), the azimuthal quantum number (l), and the magnetic quantum number (m). When an electron moves from one energy level to another, it undergoes an energy transition. During these transitions, electrons either absorb or emit energy in the form of photons. For instance, when an electron jumps from a lower energy level to a higher one, it absorbs a photon with an energy equal to the difference between the two levels. Conversely, when an electron falls from a higher energy level to a lower one, it emits a photon with the same energy difference. These transitions are governed by selection rules, which dictate the allowed transitions based on the changes in quantum numbers. In the context of singlets, which are a type of molecular state where all electron spins are paired, understanding energy levels and transitions is crucial. Singlets are typically the ground state of molecules with an even number of electrons, where each electron is paired with another electron of opposite spin in the same orbital. However, when these molecules are excited by absorbing energy (such as light), they can transition to higher energy states known as triplets, where two electrons have parallel spins. The transition from a singlet to a triplet state is generally forbidden by selection rules because it involves a change in the total spin of the system. However, this transition can occur through mechanisms like intersystem crossing or spin-orbit coupling, which allow for a change in spin state. Understanding these transitions is essential for applications in fields such as photochemistry and photophysics, where the manipulation of singlet and triplet states can lead to significant outcomes like fluorescence or phosphorescence. Moreover, the study of energy levels and transitions in singlets has practical implications in materials science and technology. For example, organic light-emitting diodes (OLEDs) rely on the efficient conversion of electrical energy into light through the emission from excited singlet states. In these devices, optimizing the energy levels and transitions between singlet and triplet states can significantly enhance their efficiency and lifespan. In summary, energy levels and transitions form the backbone of understanding the behavior of singlets and their role in various scientific and technological applications. By grasping these fundamental principles, researchers can better design and optimize systems that exploit the unique properties of singlet states, leading to advancements in fields ranging from basic chemistry to cutting-edge technology.
Applications and Significance of Singlets
The concept of singlets, particularly in the context of molecular states, has far-reaching implications across various scientific disciplines. Singlets play a crucial role in chemical reactions and catalysis, where they can significantly influence reaction pathways and efficiencies. Additionally, they are integral to biological processes, including photosynthesis, where the transfer of energy from light to chemical bonds relies on singlet states. Furthermore, technological innovations and advancements in materials science also benefit from the unique properties of singlets, enabling the development of novel materials and devices. This article delves into these three key areas, starting with the pivotal role of singlets in chemical reactions and catalysis. By understanding how singlets interact and influence these processes, we can unlock new avenues for improving catalytic efficiency, enhancing biological functions, and driving technological progress. Transitioning to the first supporting section, we explore how singlets participate in and facilitate chemical reactions and catalysis, highlighting their significance in modern chemistry.
Chemical Reactions and Catalysis
Chemical reactions are the cornerstone of various industrial, biological, and environmental processes, and their efficiency is often significantly enhanced by the presence of catalysts. A catalyst is a substance that speeds up a chemical reaction without being consumed or altered in the process. This concept is crucial in understanding the applications and significance of singlets, particularly in contexts where energy transfer and molecular interactions play pivotal roles. In chemical reactions, catalysts work by lowering the activation energy required for the reaction to proceed, thereby increasing the rate of reaction. This can be achieved through several mechanisms, such as providing an alternative reaction pathway with a lower energy barrier or stabilizing transition states. For instance, in the Haber-Bosch process, iron-based catalysts are used to facilitate the synthesis of ammonia from nitrogen and hydrogen gases, a reaction that is vital for the production of fertilizers and other chemicals. Catalysis is not limited to industrial processes; it also plays a critical role in biological systems. Enzymes, which are biological catalysts, are essential for numerous metabolic pathways. They ensure that biochemical reactions occur at rates that are compatible with life processes. For example, lactase is an enzyme that catalyzes the breakdown of lactose into glucose and galactose in the human digestive system, enabling individuals to digest milk. The significance of catalysis extends to environmental applications as well. Catalytic converters in vehicles use precious metals like platinum and palladium to convert harmful pollutants such as carbon monoxide, hydrocarbons, and nitrogen oxides into less harmful substances like carbon dioxide, water vapor, and nitrogen. This technology has been instrumental in reducing air pollution and improving air quality. In the context of singlets—specifically singlet oxygen (¹O₂) and singlet states in general—catalysis can be particularly relevant. Singlet oxygen is a highly reactive species that can be generated through photosensitization processes involving light and certain molecules. This reactive oxygen species is used in photodynamic therapy to treat cancer by selectively targeting and destroying cancer cells while sparing healthy tissue. The efficiency of this process can be enhanced by catalysts that improve the generation or stabilization of singlet oxygen. Moreover, singlet states in molecules can influence catalytic processes by altering the electronic properties of catalysts or substrates. For example, in some photocatalytic systems, the excitation of molecules to singlet states can initiate redox reactions that drive catalytic cycles. Understanding these interactions is crucial for developing more efficient photocatalysts for applications such as water splitting and organic synthesis. In summary, catalysis is a fundamental aspect of chemical reactions that enhances their efficiency and selectivity. The applications of catalysis span industrial, biological, and environmental domains, and its significance is further underscored by its role in processes involving singlet states. By leveraging catalysts to manipulate singlet states effectively, scientists can develop innovative solutions for various challenges, from improving industrial processes to advancing medical treatments. This synergy between catalysis and singlet states highlights the profound impact that understanding these concepts can have on advancing technology and improving human well-being.
Biological Processes and Photosynthesis
In the intricate tapestry of life, biological processes are the fundamental mechanisms that sustain and drive the complexity of living organisms. Among these, photosynthesis stands out as a cornerstone of life on Earth, underpinning the food chain and atmospheric balance. Photosynthesis is the biochemical process by which green plants, algae, and some bacteria convert light energy from the sun into chemical energy in the form of organic compounds, such as glucose. This process not only provides energy for plant growth but also produces oxygen as a byproduct, which is crucial for the survival of nearly all aerobic organisms. The significance of photosynthesis extends far beyond its role in plant metabolism. It is the primary source of organic matter and oxygen in the biosphere, supporting the entire food web. Herbivores feed on plants, carnivores feed on herbivores, and decomposers break down dead organisms, all relying on the energy stored through photosynthesis. Moreover, photosynthesis plays a critical role in regulating Earth's climate by removing carbon dioxide from the atmosphere and releasing oxygen, thus influencing the global carbon cycle. The efficiency of photosynthesis is influenced by various factors, including light intensity, temperature, water availability, and nutrient supply. Chlorophyll, a green pigment found in chloroplasts of plant cells, is central to this process as it absorbs light energy and transfers it to other molecules, initiating the conversion of carbon dioxide and water into glucose and oxygen. This complex interplay highlights the sophisticated biochemical machinery involved in photosynthesis. Understanding the biological processes underlying photosynthesis has numerous practical applications. For instance, agricultural practices aim to optimize photosynthetic efficiency through techniques such as crop rotation, fertilization, and irrigation. Additionally, research into photosynthetic pathways has inspired innovations in renewable energy technologies, such as biofuels and artificial photosynthesis systems designed to mimic nature's efficiency in converting sunlight into usable energy. In the context of singlets—excited states of molecules with paired electron spins—photosynthesis offers a fascinating example of their role in biological systems. During the light-dependent reactions of photosynthesis, light absorption by chlorophyll and other pigments leads to the formation of excited singlet states. These singlet states are crucial for initiating electron transfer reactions that drive the subsequent biochemical steps necessary for energy conversion. The efficient transfer of energy from these singlet states to other molecules within the photosynthetic apparatus underscores the importance of understanding singlet dynamics in biological processes. In conclusion, photosynthesis exemplifies the intricate and essential biological processes that underpin life on Earth. Its significance extends from supporting the food chain and regulating atmospheric composition to inspiring technological innovations. The role of singlets in photosynthesis highlights their critical function in energy conversion within biological systems, emphasizing the importance of continued research into these molecular states for both fundamental understanding and practical applications.
Technological Innovations and Materials Science
Technological innovations and advancements in materials science have revolutionized the field of textiles, particularly in the development and application of singlets. A singlet, a lightweight, sleeveless garment, has evolved significantly from its humble beginnings as a simple athletic wear item. Today, it is a testament to the synergy between technology and materials science. Modern singlets are crafted using cutting-edge materials such as moisture-wicking fabrics, breathable mesh panels, and high-performance synthetic fibers like polyester and nylon. These materials are engineered to enhance athletic performance by regulating body temperature, managing sweat, and providing comfort during intense physical activities. For instance, moisture-wicking fabrics like Coolmax and Dri-FIT are designed to draw sweat away from the skin, allowing it to evaporate quickly, thus keeping athletes cool and dry. This technology not only improves performance but also reduces the risk of skin irritation and discomfort. Moreover, advancements in nanotechnology have led to the development of smart fabrics that incorporate nanoparticles for enhanced functionality. These fabrics can offer UV protection, antimicrobial properties, and even self-cleaning capabilities. For example, some singlets now feature built-in UPF (Ultraviolet Protection Factor) protection, safeguarding athletes from harmful UV rays during outdoor activities. Additionally, antimicrobial treatments can prevent the growth of bacteria and odor-causing microorganisms, ensuring that the garment remains fresh and hygienic over multiple uses. The integration of wearable technology is another significant innovation in singlet design. Many contemporary singlets are equipped with wearable sensors that monitor vital signs such as heart rate, body temperature, and muscle activity. These sensors provide real-time data that can be used to optimize training regimens, prevent injuries, and enhance overall athletic performance. For instance, some high-tech singlets include built-in ECG sensors that track heart activity during exercise, offering valuable insights into cardiovascular health. Furthermore, sustainable materials science has also played a crucial role in the evolution of singlets. Eco-friendly fabrics made from recycled plastics, organic cotton, and plant-based materials are becoming increasingly popular. These sustainable options not only reduce environmental impact but also offer comparable performance to traditional synthetic fibers. Brands are now focusing on circular economy practices by designing singlets that are recyclable or biodegradable at the end of their life cycle. In conclusion, the intersection of technological innovations and materials science has transformed the humble singlet into a sophisticated piece of athletic wear. From high-performance fabrics to wearable technology and sustainable materials, these advancements have significantly enhanced the functionality, comfort, and environmental footprint of singlets. As technology continues to evolve, it is likely that future singlets will incorporate even more innovative features that further elevate athletic performance while promoting sustainability and well-being.