What Gets Wetter The More It Dries
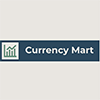
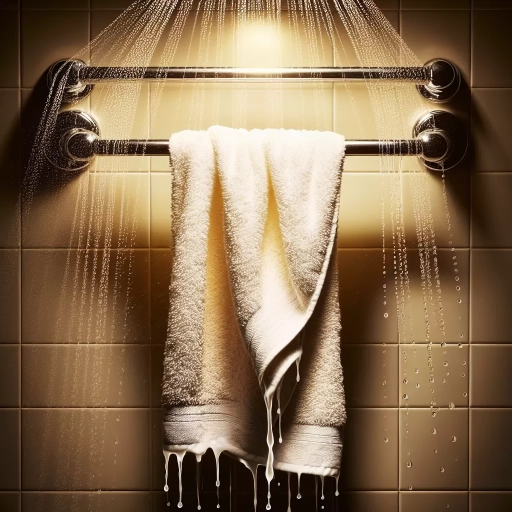
In the realm of everyday phenomena, there exists a fascinating paradox that challenges our intuitive understanding of how materials interact with water. The concept that something can become wetter the more it dries seems counterintuitive, yet it is a reality observed in certain absorbent materials. This intriguing phenomenon is not just a curiosity but has significant implications across various fields. In this article, we will delve into the paradox of absorbent materials, exploring how they defy conventional logic. We will also examine real-world applications and examples where this property is leveraged, from industrial processes to everyday products. Additionally, we will uncover the scientific principles behind this phenomenon, shedding light on the underlying mechanisms that make it possible. By understanding these aspects, we can appreciate the complexity and utility of materials that become wetter as they dry. Let us begin by unraveling the paradox of absorbent materials, where the seemingly impossible becomes a tangible reality.
The Paradox of Absorbent Materials
The paradox of absorbent materials lies in their unique ability to soak up and retain large amounts of liquid, often exceeding their own weight, while maintaining structural integrity. This phenomenon is both fascinating and crucial for various applications across industries. To delve into this paradox, it is essential to explore three key aspects: the properties of absorbent materials, examples of these materials in real-world use, and the scientific explanation behind their absorbency. Understanding the **Properties of Absorbent Materials** is foundational, as it reveals the intrinsic characteristics that enable these materials to absorb liquids efficiently. These properties include high surface area, porosity, and capillary action, which collectively allow for effective liquid uptake and retention. **Examples of Absorbent Materials** illustrate how these properties are utilized in practical scenarios. From everyday products like paper towels and diapers to industrial applications such as oil spill cleanups and medical devices, absorbent materials play a vital role in our daily lives. Finally, a **Scientific Explanation** provides insight into the underlying mechanisms that govern the absorbency of these materials. This involves understanding the interactions between the material's structure and the liquid being absorbed, which can be explained through principles of chemistry and physics. By examining these three facets, we can gain a comprehensive understanding of the paradoxical nature of absorbent materials. Let us begin by exploring the **Properties of Absorbent Materials**, which form the basis of their extraordinary capabilities.
Properties of Absorbent Materials
Absorbent materials, despite their seemingly paradoxical nature, possess a set of unique properties that make them indispensable in various applications. At the heart of their functionality lies their ability to absorb and retain liquids, a trait that is fundamentally tied to their structural and chemical characteristics. One of the primary properties of absorbent materials is their high surface area, which allows them to come into contact with a large volume of liquid. This is often achieved through the presence of pores or capillaries within the material, such as in the case of cellulose fibers in paper or the intricate network of channels in sponge-like materials. Another critical property is the material's affinity for water, known as hydrophilicity. Hydrophilic materials have a strong attraction to water molecules, enabling them to draw in and hold onto liquid effectively. This affinity can be enhanced by chemical treatments or the incorporation of hydrophilic groups into the material's structure. For instance, certain types of fabric can be treated with chemicals that increase their hydrophilic properties, making them more absorbent. The capillary action of absorbent materials is also a key factor in their performance. Capillary action refers to the ability of a material to draw liquid up through narrow spaces without the need for pressure. This phenomenon is crucial in materials like paper towels and sponges, where it allows the liquid to spread evenly throughout the material, maximizing absorption efficiency. In addition to these physical properties, the durability and resilience of absorbent materials are important considerations. Many absorbent materials are designed to withstand repeated use and exposure to liquids without losing their effectiveness. For example, superabsorbent polymers used in diapers and sanitary products can absorb multiple times their weight in liquid and retain it under pressure, making them highly durable. Moreover, the environmental impact of absorbent materials has become increasingly significant. Eco-friendly absorbent materials, such as those derived from natural fibers or biodegradable polymers, offer sustainable alternatives to traditional synthetic materials. These materials not only reduce waste but also minimize the environmental footprint associated with their production and disposal. The paradoxical nature of absorbent materials—that they get wetter the more they dry—can be understood through their unique behavior under different conditions. When an absorbent material dries out, it often becomes more efficient at absorbing liquid due to the re-establishment of its capillary network and the reactivation of its hydrophilic sites. This phenomenon highlights the dynamic interplay between the material's structure, its interaction with liquids, and its ability to recover its absorbency after drying. In conclusion, the properties of absorbent materials—high surface area, hydrophilicity, capillary action, durability, and environmental sustainability—make them versatile tools in a wide range of applications. Understanding these properties not only explains how these materials function but also underscores their importance in everyday life, from household cleaning products to advanced medical devices. The paradox of absorbent materials getting wetter the more they dry is a testament to their complex and fascinating nature, showcasing the intricate science behind their seemingly simple functionality.
Examples of Absorbent Materials
In the intriguing realm of absorbent materials, several examples highlight the paradoxical nature of these substances, which often become more effective at drying the more they are exposed to moisture. One of the most common and versatile absorbent materials is **cellulose**, found in paper products like paper towels and tissues. Cellulose fibers have a high capacity to absorb water due to their hydrophilic (water-loving) properties, making them ideal for cleaning spills and wiping surfaces. However, the more cellulose absorbs water, the more it swells and becomes less effective at absorbing additional moisture, illustrating the paradox where increased wetness can lead to reduced absorbency over time. Another significant example is **diapers**, which rely on superabsorbent polymers (SAPs) like sodium polyacrylate. These polymers can absorb hundreds of times their weight in water, turning liquid into a gel-like substance that prevents leakage. Despite their high absorbency, SAPs work best when they are initially dry; as they absorb more liquid, their ability to retain additional moisture can diminish due to saturation, showcasing the paradox where the material's effectiveness peaks before it becomes fully saturated. **Cotton** is another absorbent material that exhibits this paradox. Cotton fibers are highly absorbent due to their natural hydrophilicity and capillary action, making them suitable for clothing and towels. However, as cotton absorbs more water, its fibers swell and become heavier, reducing its ability to dry quickly. This phenomenon is particularly evident in towels that become less absorbent after repeated washing and use because the fibers start to break down and lose their natural absorbency. In industrial applications, **activated carbon** is used for its high surface area and adsorption capabilities. While not traditionally thought of as an absorbent material in the same way as cellulose or cotton, activated carbon can absorb gases and liquids through adsorption. The paradox here lies in the fact that activated carbon's effectiveness can be compromised if it becomes too saturated with moisture or contaminants, reducing its ability to adsorb further substances. Lastly, **zeolites** are microporous minerals that have been used in various applications from water purification to cat litter due to their high absorbency and ion-exchange properties. Zeolites can absorb significant amounts of water and other substances but may lose their effectiveness if they become overly saturated or if their pores are clogged by absorbed materials, again highlighting the paradox where increased absorption can lead to decreased performance. These examples illustrate how absorbent materials, despite their initial high capacity for absorption, can become less effective as they become wetter. This paradox underscores the complex relationship between absorbency and saturation, emphasizing the importance of understanding the limitations and optimal conditions for using these materials in various applications.
Scientific Explanation
Scientific explanation is the cornerstone of understanding natural phenomena, and it plays a crucial role in unraveling the paradox of absorbent materials. At its core, scientific explanation involves the systematic observation, measurement, and experimentation to formulate hypotheses and theories that explain how and why certain events occur. In the context of absorbent materials, such as sponges or towels, scientific explanation delves into the physical and chemical properties that govern their behavior. When we observe that an absorbent material appears to get wetter the more it dries, it seems counterintuitive at first glance. However, a closer examination through the lens of scientific inquiry reveals the underlying mechanisms. The key lies in understanding capillary action, surface tension, and the structure of the material itself. Capillary action, for instance, is the ability of a liquid to flow through a narrow space without the need for pressure; this is crucial in how absorbent materials draw in and retain water. Surface tension, another fundamental concept, influences how water molecules interact with the material's surface, affecting absorption rates. Moreover, the microscopic structure of absorbent materials—often comprising a network of pores and fibers—plays a significant role. These pores and fibers create a large surface area that allows for efficient water absorption. When an absorbent material is used repeatedly, it may undergo changes such as compaction or alteration in its pore structure due to mechanical stress or drying cycles. This can temporarily reduce its absorbency but does not necessarily mean it becomes less wet; rather, it may distribute water differently within its structure. From a thermodynamic perspective, drying an absorbent material involves the removal of water molecules from its pores and fibers. This process can sometimes make the material appear wetter because the remaining water redistributes within the material's structure, potentially leading to a more uniform saturation. Additionally, some absorbent materials may exhibit hysteresis in their water retention properties, meaning their ability to absorb and release water can vary depending on whether they are being wetted or dried. In conclusion, the paradox of absorbent materials getting wetter the more they dry is resolved through a comprehensive scientific explanation that considers capillary action, surface tension, material structure, and thermodynamic principles. By understanding these underlying mechanisms, we can better appreciate why such materials behave in seemingly contradictory ways and how they can be optimized for various applications. This exemplifies how scientific explanation not only clarifies natural phenomena but also provides practical insights that can enhance our daily lives and technological advancements.
Real-World Applications and Examples
In today's world, the real-world applications of various technologies and innovations are multifaceted and far-reaching. From industrial uses that drive economic growth and efficiency, to household applications that enhance daily life, and environmental impacts that shape our sustainability efforts, these applications are integral to modern society. Industrial uses, for instance, leverage advanced technologies to optimize production processes, improve safety standards, and reduce operational costs. Household applications, on the other hand, bring convenience and comfort into our homes through smart devices and energy-efficient solutions. Meanwhile, the environmental impact of these technologies is a critical consideration, as they can either exacerbate or mitigate ecological challenges. Understanding these diverse applications is crucial for appreciating the full scope of innovation's influence. In this article, we will delve into these three key areas, starting with the significant role that industrial uses play in shaping our economic landscape. --- **Industrial Uses**
Industrial Uses
In the realm of industrial applications, the phenomenon of materials getting wetter the more they dry is a fascinating and highly utilizable concept. This unique property, often observed in certain types of porous materials and superabsorbents, has far-reaching implications across various sectors. For instance, in the textile industry, fabrics engineered with such properties can be used to create advanced moisture-wicking clothing. These garments are designed to absorb and retain moisture from the skin, allowing it to evaporate quickly, thereby keeping the wearer cool and dry. This technology is particularly beneficial for athletes and individuals working in high-temperature environments, enhancing comfort and performance. In agriculture, these materials can be integrated into soil amendments to improve water retention and reduce the need for frequent irrigation. By absorbing and holding water, these superabsorbent polymers help maintain soil moisture levels, ensuring that plants receive consistent hydration even during periods of drought. This not only conserves water but also promotes healthier plant growth and higher crop yields. The construction industry also leverages these materials to enhance building durability and sustainability. Superabsorbent concrete additives can absorb excess water during the mixing process, reducing the risk of cracking and improving the overall strength of the concrete. Additionally, these additives can help in self-healing concrete by absorbing water and releasing it when needed to repair micro-cracks, thereby extending the lifespan of structures. In the field of environmental engineering, these materials play a crucial role in water treatment and pollution control. Superabsorbent polymers can be used to clean up oil spills by absorbing oil while repelling water, making the cleanup process more efficient and effective. Furthermore, they can be employed in wastewater treatment plants to remove contaminants and heavy metals from water, contributing to cleaner and safer water supplies. The pharmaceutical industry also benefits from these materials, particularly in drug delivery systems. Superabsorbent hydrogels can be designed to release medications in a controlled manner, responding to changes in moisture levels. This allows for more precise and efficient drug delivery, improving patient outcomes and reducing side effects. Lastly, in the automotive sector, these materials are used in various applications such as soundproofing and vibration damping. By absorbing moisture and maintaining their structural integrity, these materials help in creating quieter and more comfortable driving experiences. In summary, the unique property of materials getting wetter the more they dry opens up a wide array of industrial applications that span multiple sectors. From enhancing athletic performance through advanced textiles to improving water conservation in agriculture, these materials are revolutionizing how we approach various challenges in modern industry. Their versatility and effectiveness make them invaluable tools for engineers and innovators seeking to create more efficient, sustainable, and high-performance products.
Household Applications
In the realm of household applications, the concept of materials that get wetter the more they dry may seem counterintuitive, but it holds significant practical value. One of the most compelling examples is in the field of cleaning products. Superabsorbent polymers, commonly found in dishwashing sponges and mops, are designed to absorb and retain large amounts of water relative to their mass. These polymers become wetter as they dry because they release water slowly, allowing for prolonged cleaning efficiency. For instance, a dish sponge that incorporates these polymers can absorb spills and wipe down surfaces effectively even after multiple uses, making it an indispensable tool in any kitchen. Another area where this phenomenon is beneficial is in laundry detergents. Some modern detergents contain superabsorbent particles that help in better water absorption and retention during the washing cycle. These particles get wetter as they dry because they continue to absorb water from the fabric, ensuring that clothes are thoroughly cleaned and rinsed. This technology enhances the overall washing performance by preventing water spots and improving fabric softness. In addition, this principle is applied in personal care products such as diapers and sanitary pads. The superabsorbent materials used in these products can absorb several times their weight in liquid, making them highly effective at managing moisture. As these materials dry, they release moisture slowly, which helps in maintaining skin health by preventing irritation and discomfort. This technology has revolutionized the way we manage hygiene and comfort in daily life. Furthermore, household insulation materials also benefit from this concept. Certain types of insulation, such as those made from superabsorbent fibers, can absorb moisture from the air and release it slowly as they dry. This helps in maintaining a stable indoor climate by regulating humidity levels and preventing mold growth. Such materials are particularly useful in regions with high humidity or in areas prone to dampness. Lastly, gardening and plant care products often utilize materials that get wetter as they dry. For example, superabsorbent hydrogels used in potting soils can retain water and release it gradually as the soil dries out. This ensures that plants receive a steady supply of moisture without overwatering, which can be detrimental to plant health. These hydrogels are especially beneficial for indoor plants or during periods of drought when consistent watering is challenging. In conclusion, the phenomenon of materials that get wetter the more they dry has numerous practical applications within household settings. From cleaning tools and laundry detergents to personal care products and insulation materials, these technologies enhance efficiency, comfort, and overall quality of life. By leveraging these innovative materials, households can achieve better hygiene, improved comfort, and more effective management of moisture-related challenges.
Environmental Impact
The environmental impact of various processes and products is a critical aspect to consider in today's world, especially when discussing real-world applications and examples. For instance, the concept of something getting "wetter the more it dries" might seem paradoxical, but it highlights the complex interactions within ecosystems and human activities. One such example is the use of certain types of detergents and soaps that, despite their drying effects on skin, can contribute to water pollution. These chemicals can enter waterways through wastewater, affecting aquatic life and water quality. Similarly, in agriculture, the overuse of fertilizers and pesticides can lead to soil degradation and increased runoff into rivers and lakes, exacerbating issues like eutrophication and biodiversity loss. In urban settings, the construction of impermeable surfaces such as roads and buildings can disrupt natural water cycles, leading to increased stormwater runoff and decreased groundwater recharge. This not only exacerbates flooding but also reduces the ability of soil to act as a natural filter, further contaminating water bodies. On the other hand, innovative solutions like green roofs and permeable pavements are being implemented to mitigate these effects by allowing rainwater to infiltrate the soil, thereby reducing urban runoff and improving air quality. Another significant area of environmental impact is industrial processes, particularly those involving water-intensive operations. For example, textile manufacturing often involves extensive use of water for dyeing and washing fabrics, resulting in large volumes of wastewater that may contain harmful chemicals. However, advancements in technology have led to the development of more sustainable practices such as waterless dyeing techniques and closed-loop systems that recycle water, significantly reducing the environmental footprint of these industries. Moreover, the production and disposal of single-use plastics have profound environmental implications. Plastic waste often ends up in oceans, contributing to marine pollution and harming marine life. The decomposition process of plastics can take hundreds of years, during which time they break down into microplastics that are ingested by animals and potentially enter the human food chain. Initiatives such as banning single-use plastics, promoting recycling, and developing biodegradable alternatives are crucial steps towards mitigating this issue. In conclusion, understanding the environmental impact of various activities is essential for developing sustainable solutions that minimize harm to ecosystems. By examining real-world applications and examples—such as the paradoxical effect of certain detergents or the consequences of industrial processes—we can identify areas for improvement and implement strategies that protect our environment while meeting human needs. This holistic approach ensures that we not only address immediate issues but also work towards a more sustainable future for generations to come.
The Science Behind the Phenomenon
The phenomenon of water rising through a narrow tube or spreading across a surface, often observed in everyday life, is rooted in several fundamental scientific principles. At its core, this phenomenon is influenced by three key factors: capillary action, surface tension and adhesion, and molecular structure and behavior. Capillary action, the ability of a liquid to flow through a narrow space without the need for pressure, is a crucial aspect that explains how water can defy gravity and rise up a tube. Surface tension and adhesion play significant roles as well, as they determine the interaction between the liquid and the surrounding material, affecting how the liquid spreads or rises. Lastly, the molecular structure and behavior of the liquid itself are essential in understanding the intermolecular forces that drive these interactions. By delving into these three areas, we can gain a comprehensive understanding of the intricate mechanisms behind this fascinating phenomenon. Let's begin by exploring capillary action in more detail.
Capillary Action
Capillary action, a fundamental phenomenon in the realm of physics and chemistry, is the ability of a liquid to flow through a narrow space, such as a tube or a porous material, without the need for pressure. This process is driven by the combination of two primary forces: adhesion and cohesion. Adhesion refers to the attractive forces between the liquid and the surface of the capillary material, while cohesion pertains to the attractive forces within the liquid itself. When these forces are balanced in a way that adhesion exceeds cohesion, the liquid is drawn into the capillary space. The science behind capillary action can be explained through the concept of surface tension. Surface tension is a property of liquids that causes them to behave as if they have an "elastic skin" at their surface. This tension arises from the cohesive forces between molecules at the surface of the liquid, which are more strongly attracted to each other than to the surrounding air or solid surfaces. In a capillary tube, this surface tension causes the liquid to form a concave meniscus (curved surface) that rises up the tube. The height to which the liquid rises in a capillary tube can be predicted using the equation for capillary rise: \( h = \frac{2 \gamma \cos(\theta)}{\rho g r} \), where \( h \) is the height of the rise, \( \gamma \) is the surface tension of the liquid, \( \theta \) is the contact angle between the liquid and the tube material, \( \rho \) is the density of the liquid, \( g \) is the acceleration due to gravity, and \( r \) is the radius of the capillary tube. This equation highlights how various factors such as surface tension, contact angle, and tube radius influence capillary action. Capillary action plays a crucial role in numerous natural and engineered systems. For instance, it is essential for plant physiology, enabling plants to transport water from their roots to their leaves through xylem tissues. In human-made systems, capillary action is utilized in paper towels and sponges to absorb spills efficiently. It also underpins the functioning of microfluidic devices used in biomedical research and diagnostics. Moreover, understanding capillary action has significant implications for materials science and engineering. Researchers design materials with specific surface properties to either enhance or inhibit capillary action. For example, hydrophilic (water-loving) surfaces can be engineered to facilitate water uptake in applications like water purification systems or biomedical implants. Conversely, hydrophobic (water-repelling) surfaces can be designed to prevent water from entering certain areas, which is useful in waterproof coatings or self-cleaning surfaces. In summary, capillary action is a fascinating phenomenon that leverages the interplay between adhesion and cohesion to facilitate liquid flow through narrow spaces. Its principles are rooted in surface tension and can be quantitatively described using well-established equations. The importance of capillary action extends across various fields from biology to engineering, making it a cornerstone of understanding how liquids interact with their surroundings. This intricate mechanism not only explains why certain materials get wetter as they dry but also underscores the versatility and critical role it plays in both natural and engineered systems.
Surface Tension and Adhesion
Surface tension and adhesion are fundamental forces that govern the behavior of liquids and their interactions with other substances, playing a crucial role in understanding why some materials appear to get wetter the more they dry. **Surface tension** is the property of a liquid that causes it to behave as if it has an "elastic skin" at its surface. This phenomenon arises due to the intermolecular forces between the molecules of the liquid, which are stronger at the surface than in the bulk. These forces cause the molecules at the surface to be drawn inward, creating a sort of "film" that minimizes the surface area of the liquid. This is why liquids tend to form droplets and why they resist external forces that try to increase their surface area. **Adhesion**, on the other hand, is the force that attracts two different substances to each other. When a liquid comes into contact with a solid, adhesion occurs between the molecules of the liquid and those of the solid. The strength of adhesion depends on the nature of both substances; for example, water adheres well to glass but poorly to Teflon. The interplay between surface tension and adhesion determines how a liquid spreads or beads up on a surface. If adhesion is stronger than surface tension, the liquid will spread out and wet the surface; if surface tension dominates, the liquid will form droplets and resist spreading. In materials that appear to get wetter as they dry, such as certain types of fabrics or porous materials, this behavior can be attributed to changes in the surface properties over time. For instance, some fabrics may initially repel water due to their hydrophobic (water-repelling) nature, but as they dry after being exposed to moisture, their surface chemistry can alter. This alteration might increase the adhesion between water molecules and the fabric's surface, making it more hydrophilic (water-attracting) over time. Similarly, in porous materials like paper or sponge-like substances, drying can cause the pores to become more open or the surface to become more hydrophilic, enhancing water absorption. Understanding these phenomena is crucial for various applications, from textile engineering to biomedical research. For example, in textile design, knowing how surface tension and adhesion affect fabric behavior can help create materials that are either highly water-repellent or highly absorbent depending on the desired application. In biomedical contexts, these principles are essential for designing medical devices that interact with bodily fluids or for developing new drug delivery systems. In summary, the intricate balance between surface tension and adhesion underpins many everyday observations and technological innovations. By grasping these fundamental forces, scientists and engineers can better explain why certain materials exhibit unique wetting behaviors and develop new materials with tailored properties for specific uses. This understanding not only enhances our appreciation of natural phenomena but also drives innovation across diverse fields.
Molecular Structure and Behavior
Molecular structure and behavior are fundamental to understanding the intriguing phenomenon where certain materials appear to get wetter the more they dry. At the heart of this phenomenon lies the intricate dance of molecules and their interactions. When we consider a material's molecular structure, we are looking at the arrangement and bonding of its constituent atoms. These arrangements dictate the material's physical and chemical properties, including its ability to absorb or repel water. In materials that exhibit this unique behavior, such as certain types of polymers or gels, the molecular structure often includes hydrophilic (water-attracting) groups. These groups are typically polar, meaning they have a partial positive charge on one end and a partial negative charge on the other, allowing them to form hydrogen bonds with water molecules. However, these materials also often contain hydrophobic (water-repelling) regions that resist water absorption. The interplay between these hydrophilic and hydrophobic regions is crucial. When the material is initially exposed to water, the hydrophilic groups attract water molecules, causing the material to swell as it absorbs moisture. However, as the material dries, the hydrophobic regions become more pronounced due to changes in molecular conformation or the evaporation of water from the surface. This can lead to an increase in the material's surface area or the exposure of more hydrophilic sites, making it appear "wetter" because it becomes more receptive to subsequent water exposure. Another key factor is the concept of capillary action, where water moves through narrow spaces without the need for pressure. In materials with a porous structure, such as certain types of fabrics or sponges, capillary action can enhance water absorption. As these materials dry, their pores may become more defined or accessible, facilitating greater water uptake upon subsequent exposure. Additionally, the behavior of molecules at the surface of these materials plays a significant role. Surface tension and contact angle are critical parameters that influence how water interacts with the material. A lower contact angle indicates that water spreads more easily across the surface, while a higher contact angle suggests that water beads up and rolls off. Changes in these surface properties during drying can alter how the material interacts with water, contributing to its apparent increased wetness. Understanding these molecular structures and behaviors not only explains why some materials seem to get wetter as they dry but also opens avenues for designing new materials with tailored properties. For instance, engineers can synthesize polymers with specific hydrophilic and hydrophobic regions to create materials that are highly absorbent yet resistant to drying out completely. This knowledge has practical applications in fields such as textiles, biomedical devices, and even environmental remediation technologies. In summary, the phenomenon of materials getting wetter as they dry is rooted in their molecular structure and behavior. The interplay between hydrophilic and hydrophobic regions, changes in molecular conformation during drying, capillary action in porous materials, and surface properties all contribute to this intriguing effect. By delving into these scientific principles, we gain insights into how materials interact with water and how we can engineer new materials with unique properties that benefit various industries and applications.