What Metals Are Not Magnetic
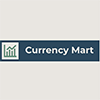
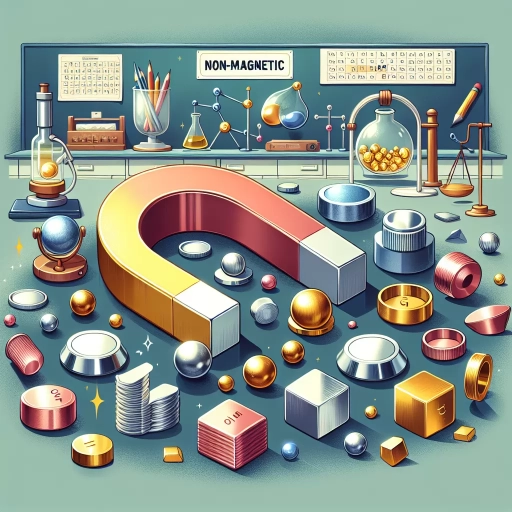
In the vast and diverse world of metals, not all exhibit magnetic properties. While some metals are renowned for their strong magnetic capabilities, others remain non-magnetic, each with unique characteristics and applications. This article delves into the fascinating realm of non-magnetic metals, exploring their classification, specific examples, and the underlying reasons for their lack of magnetism. We begin by providing an overview and classification of non-magnetic metals, understanding how they are categorized and distinguished from their magnetic counterparts. Next, we will examine specific examples of non-magnetic metals, highlighting their uses and importance in various industries. Finally, we will delve into the scientific explanations behind why some metals are not magnetic, shedding light on the atomic and structural factors that contribute to this phenomenon. By understanding these aspects, we can better appreciate the diverse properties of metals and their critical roles in modern technology. Let us start with a comprehensive overview and classification of non-magnetic metals.
Non-Magnetic Metals: Overview and Classification
Non-magnetic metals are a crucial category of materials that play a significant role in various industrial and technological applications. These metals, unlike their magnetic counterparts, do not exhibit magnetic properties, making them invaluable in contexts where magnetism could be detrimental. This article provides a comprehensive overview of non-magnetic metals, delving into their definition and properties, classification based on atomic structure, and their common applications. Firstly, understanding the definition and properties of non-magnetic metals is essential to grasp their unique characteristics. This includes their atomic composition, electrical conductivity, and mechanical strength, which set them apart from magnetic metals. Secondly, the classification of these metals based on their atomic structure reveals how their electronic configurations influence their non-magnetic behavior. Finally, exploring the common applications of non-magnetic metals highlights their importance in fields such as aerospace, medical devices, and electrical engineering. By examining these aspects, we can appreciate the versatility and utility of non-magnetic metals. To begin this exploration, let's delve into the **Definition and Properties of Non-Magnetic Metals**, where we will uncover the fundamental characteristics that define these materials and distinguish them from other metals.
Definition and Properties of Non-Magnetic Metals
Non-magnetic metals are a category of metals that do not exhibit magnetic properties, meaning they do not attract magnets or show any significant magnetic behavior. These metals are crucial in various industrial and technological applications where magnetic interference needs to be minimized. The definition of non-magnetic metals hinges on their atomic structure and the arrangement of electrons, particularly the absence of unpaired electrons which are responsible for magnetism in ferromagnetic materials like iron, nickel, and cobalt. One of the key properties of non-magnetic metals is their lack of ferromagnetism. Ferromagnetism arises from the alignment of unpaired electron spins in the same direction, creating a net magnetic moment. In contrast, non-magnetic metals have paired electron spins or no unpaired electrons, resulting in no net magnetic moment. This characteristic makes them ideal for use in environments where magnetic fields could interfere with operations, such as in medical equipment, electronic devices, and aerospace components. Another significant property is their high electrical conductivity. Many non-magnetic metals, such as copper, aluminum, and silver, are excellent conductors of electricity. This property is vital for applications in electrical wiring, circuits, and electronic components where efficient current flow is essential. Additionally, these metals often possess high thermal conductivity, making them suitable for heat transfer applications like radiators and heat sinks. Non-magnetic metals also exhibit a range of mechanical properties that make them versatile. For instance, titanium and zirconium are known for their high strength-to-weight ratio and corrosion resistance, making them valuable in aerospace and marine industries. Similarly, tin and lead have low melting points and are used extensively in soldering and as protective coatings against corrosion. Furthermore, the chemical inertness of some non-magnetic metals is another important property. For example, gold and platinum are highly resistant to corrosion and chemical reactions due to their full outer electron shell configuration. This inertness makes them ideal for use in jewelry, catalytic converters in vehicles, and laboratory equipment where chemical purity is critical. In terms of classification, non-magnetic metals can be broadly categorized into several groups based on their primary uses and properties. The noble metals (like gold, silver, and platinum) are known for their chemical inertness and high ductility. The transition metals (such as titanium and zirconium) are valued for their strength and corrosion resistance. The post-transition metals (like tin and lead) are often used in soldering and as protective coatings due to their low melting points. In conclusion, non-magnetic metals offer a unique set of properties that make them indispensable across various industries. Their lack of magnetism, combined with other attributes such as high electrical conductivity, mechanical strength, and chemical inertness, ensures their widespread application in modern technology. Understanding these properties is essential for selecting the appropriate metal for specific applications where magnetic interference must be avoided or minimized.
Classification Based on Atomic Structure
Classification based on atomic structure is a fundamental approach in understanding the properties of metals, including their magnetic behavior. This method delves into the arrangement of electrons within an atom, which significantly influences a metal's magnetic characteristics. Non-magnetic metals, for instance, typically have a full outer energy level or a specific electron configuration that results in no unpaired electrons. In the context of atomic structure, metals are classified based on their electron shell configurations. Non-magnetic metals often belong to the s-block and p-block elements of the periodic table. For example, metals like copper (Cu), silver (Ag), and gold (Au) are part of the d-block but have fully filled d subshells in their ground state, leading to no unpaired electrons and thus no magnetic moment. Similarly, metals such as zinc (Zn) and cadmium (Cd) from the d-block also exhibit non-magnetic behavior due to their electron configurations. The atomic structure classification also involves understanding the concept of electron spin and orbital angular momentum. In non-magnetic metals, the electrons are paired, meaning their spins are aligned in opposite directions, resulting in no net magnetic moment. This pairing is a direct consequence of the Pauli exclusion principle and Hund's rule, which dictate how electrons occupy orbitals. Furthermore, the crystal structure of metals can influence their magnetic properties. However, for non-magnetic metals, the crystal structure does not introduce significant magnetic interactions because the atomic magnetic moments are inherently zero or cancel each other out. This is why metals like aluminum (Al) and tin (Sn), despite having different crystal structures, remain non-magnetic. Understanding the classification based on atomic structure is crucial for predicting and explaining the physical properties of metals. It provides a clear rationale for why certain metals are non-magnetic while others exhibit strong magnetic behavior. This knowledge is essential in various fields such as materials science, where selecting the appropriate metal for specific applications depends on its magnetic properties among other characteristics. In summary, the classification of metals based on their atomic structure offers a comprehensive framework for understanding their magnetic behavior. Non-magnetic metals, characterized by their electron configurations and lack of unpaired electrons, are a prime example of how atomic structure dictates physical properties. This classification not only aids in the identification of non-magnetic metals but also underscores the importance of electron configuration in determining a metal's overall behavior.
Common Applications of Non-Magnetic Metals
Non-magnetic metals, which do not exhibit magnetic properties, find a wide range of applications across various industries due to their unique characteristics. One of the most significant applications is in the aerospace industry, where non-magnetic metals like aluminum, titanium, and certain alloys are preferred for constructing aircraft and spacecraft. These metals are lightweight, corrosion-resistant, and maintain their structural integrity under extreme conditions, making them ideal for reducing weight while ensuring safety and performance. In the medical field, non-magnetic metals such as titanium and certain stainless steel alloys are crucial for medical implants and surgical instruments. Titanium, for instance, is widely used in orthopedic implants like hip and knee replacements due to its biocompatibility, strength-to-weight ratio, and resistance to corrosion. Additionally, non-magnetic properties are essential for MRI compatibility, ensuring that patients with implants can safely undergo magnetic resonance imaging without any adverse effects. The electronics industry also relies heavily on non-magnetic metals. Copper, for example, is a primary material in electrical wiring and circuits due to its excellent conductivity and non-magnetic nature. Silver and gold are used in electronic components and connectors because of their high conductivity and resistance to oxidation. These metals help in maintaining the integrity of electronic signals without interference from magnetic fields. In construction and architecture, non-magnetic metals are used for various purposes. Aluminum is a popular choice for building facades and structural components due to its light weight, corrosion resistance, and ease of fabrication. Stainless steel, another non-magnetic metal, is used in high-rise buildings and bridges for its strength, durability, and resistance to environmental degradation. Furthermore, non-magnetic metals play a critical role in the automotive sector. Aluminum alloys are increasingly used in vehicle bodies to reduce weight and improve fuel efficiency while maintaining structural strength. This shift towards lighter materials helps in reducing emissions and enhancing overall vehicle performance. In addition to these industrial applications, non-magnetic metals are also used in everyday consumer products. For instance, kitchen utensils and cookware often use stainless steel or aluminum due to their non-reactive nature with food, ease of cleaning, and resistance to corrosion. These materials ensure that food preparation remains safe and hygienic. Lastly, the marine industry benefits significantly from non-magnetic metals. Aluminum and certain stainless steel alloys are used in boat construction because they resist seawater corrosion better than magnetic metals like iron. This extends the lifespan of marine vessels and reduces maintenance costs over time. In summary, the applications of non-magnetic metals span multiple sectors, leveraging their unique properties such as light weight, corrosion resistance, high conductivity, and biocompatibility. These characteristics make them indispensable in modern technology and everyday life, contributing significantly to advancements in various fields while ensuring safety, efficiency, and durability.
Examples of Non-Magnetic Metals
Non-magnetic metals are a diverse group of materials that do not exhibit magnetic properties, making them crucial in various industrial and everyday applications. These metals are often chosen for their unique characteristics, such as high conductivity, strength, and resistance to corrosion. For instance, aluminum stands out for its lightweight yet robust nature, making it a staple in aerospace and automotive industries. Copper, on the other hand, is renowned for its exceptional electrical conductivity, which is vital for electrical wiring and electronics. Titanium, with its remarkable strength-to-weight ratio and biocompatibility, is frequently used in medical implants and high-performance sports equipment. Each of these metals has distinct uses and properties that make them indispensable in modern technology. This article will delve into the specific uses and characteristics of these non-magnetic metals, starting with aluminum, which has become a cornerstone in modern manufacturing due to its versatility and efficiency. By exploring the uses and characteristics of aluminum, we can better understand why it is such a valuable resource in today's world.
Aluminum: Uses and Characteristics
Aluminum, a versatile and widely used metal, stands out for its unique characteristics and diverse applications, making it an exemplary example of non-magnetic metals. With an atomic number of 13, aluminum is the most abundant metal in the Earth's crust, primarily found in the form of bauxite ore. Its non-magnetic nature is due to its electronic configuration, which results in a zero net magnetic moment. This property, combined with its high strength-to-weight ratio, excellent corrosion resistance, and good electrical conductivity, makes aluminum an indispensable material in various industries. One of the most significant uses of aluminum is in the aerospace industry. Its lightweight yet strong properties make it ideal for aircraft and spacecraft components, reducing overall weight while maintaining structural integrity. Additionally, aluminum's high thermal conductivity and corrosion resistance are crucial for heat sinks and other components in electronic devices. In the automotive sector, aluminum alloys are increasingly used to manufacture vehicle bodies and engine parts, contributing to fuel efficiency and reduced emissions. In construction, aluminum is favored for its durability and resistance to weathering. It is commonly used in building facades, roofing materials, and structural components due to its ability to withstand harsh environmental conditions without significant degradation. The food and beverage industry also relies heavily on aluminum for packaging materials such as cans and foil, which offer excellent barrier properties against moisture and oxygen. Aluminum's electrical conductivity is another key characteristic that makes it valuable in power transmission lines and electrical wiring. Although it has about 60% of the electrical conductivity of copper, its lower cost and lighter weight often make it a more economical choice for long-distance power transmission. Furthermore, aluminum's recyclability is a significant environmental advantage. It can be recycled repeatedly without losing its properties, making it a sustainable choice for many applications. This recyclability also reduces the energy required for primary production, contributing to a lower carbon footprint. In terms of health and safety, aluminum is generally considered non-toxic and safe for use in cookware and food packaging. However, it is important to note that high levels of aluminum exposure have been linked to certain health issues, so proper handling and disposal are essential. In conclusion, aluminum's unique combination of physical properties—non-magnetic nature, high strength-to-weight ratio, excellent corrosion resistance, good electrical conductivity, and recyclability—makes it a versatile metal with a wide range of applications across various industries. Its importance extends from aerospace and automotive to construction and consumer goods, solidifying its position as one of the most valuable non-magnetic metals in modern society.
Copper: Properties and Industrial Applications
Copper, with its distinctive reddish-orange hue, is one of the most versatile and widely used metals in various industrial applications. Its unique properties make it an indispensable material in modern technology. Copper is an excellent conductor of electricity and heat, second only to silver in terms of electrical conductivity. This property makes it a cornerstone in electrical wiring, circuits, and electronic devices. Additionally, copper has high ductility and malleability, allowing it to be easily shaped into wires, sheets, and other forms without losing its structural integrity. In the realm of industrial applications, copper plays a pivotal role. It is extensively used in the construction of electrical grids due to its ability to efficiently transmit power over long distances with minimal energy loss. The automotive industry also relies heavily on copper for wiring and electrical components in vehicles. Furthermore, copper's antimicrobial properties make it a preferred choice for medical equipment and hospital fixtures, reducing the risk of bacterial and viral infections. Copper alloys, such as brass (copper-zinc) and bronze (copper-tin), are equally valuable. Brass is often used in musical instruments due to its acoustic properties, while bronze is utilized in bearings and other mechanical parts because of its strength and resistance to corrosion. The architectural sector benefits from copper's aesthetic appeal and durability; it is commonly used in roofing, cladding, and decorative fixtures. Moreover, copper's role in renewable energy systems is becoming increasingly significant. It is a key component in solar panels and wind turbines, facilitating the efficient conversion of renewable energy into electrical power. The recycling of copper is also a critical aspect of its industrial application, as it can be reused multiple times without losing its properties, making it a sustainable choice for various industries. In the context of non-magnetic metals, copper stands out as a prime example. Unlike ferromagnetic metals like iron and nickel, copper does not exhibit magnetic properties, making it ideal for applications where magnetic interference needs to be minimized. This characteristic is particularly important in electronic devices where magnetic fields could disrupt functionality. Overall, copper's unique combination of physical and chemical properties, coupled with its extensive range of industrial applications, underscores its importance in modern society. From electrical infrastructure to medical equipment and renewable energy systems, copper continues to play a vital role in advancing technology and improving daily life. Its non-magnetic nature further enhances its utility across diverse sectors, solidifying its position as one of the most valuable non-magnetic metals available.
Titanium: Strengths and Common Uses
Titanium, known for its exceptional strength-to-weight ratio, is a versatile and highly sought-after non-magnetic metal. Its unique combination of properties makes it an ideal material for a wide range of applications. One of the primary strengths of titanium is its high tensile strength, which is comparable to that of steel but with a significantly lower density. This makes titanium particularly valuable in industries where weight reduction is crucial, such as aerospace and defense. For instance, titanium alloys are extensively used in aircraft and spacecraft components due to their ability to withstand extreme temperatures and stresses while maintaining structural integrity. Another significant advantage of titanium is its excellent corrosion resistance. It forms a thin oxide layer on its surface when exposed to air, which protects it from further corrosion and makes it highly durable in harsh environments. This characteristic is particularly beneficial in marine and chemical industries, where exposure to seawater or corrosive substances is common. For example, titanium is often used in desalination plants, offshore drilling equipment, and chemical processing facilities because it can withstand the corrosive effects of saltwater and various chemicals. In addition to its mechanical and chemical properties, titanium also has a high degree of biocompatibility, making it an essential material in medical applications. It is widely used in surgical implants such as hip and knee replacements, dental implants, and surgical instruments due to its non-toxic nature and ability to integrate well with human tissue. The biocompatibility of titanium also extends to its use in consumer products like eyeglass frames and jewelry, where it is valued for its hypoallergenic properties. Furthermore, titanium's non-magnetic nature makes it an attractive choice for applications where magnetic interference needs to be minimized. This is particularly important in fields such as electronics and medical imaging, where magnetic fields could interfere with sensitive equipment. For instance, titanium is used in MRI (Magnetic Resonance Imaging) machines because it does not react to magnetic fields, ensuring accurate imaging without interference. In summary, titanium's unique blend of high strength, low weight, excellent corrosion resistance, biocompatibility, and non-magnetic properties makes it a versatile and indispensable metal across various industries. From aerospace and marine applications to medical devices and consumer products, titanium continues to play a critical role in modern technology due to its exceptional performance characteristics. As one of the most prominent non-magnetic metals available today, titanium stands out as a material that offers unparalleled benefits in terms of durability, functionality, and safety.
Why Some Metals Are Not Magnetic
The phenomenon of magnetism in metals is a complex and multifaceted topic, influenced by several key factors. Understanding why some metals are not magnetic requires a deep dive into the underlying principles that govern their magnetic properties. At the heart of this inquiry lies the atomic electron configuration, which plays a crucial role in determining whether a metal exhibits magnetism. Additionally, the crystal structure of a metal can significantly impact its magnetic behavior, as the arrangement of atoms within the lattice can either enhance or diminish magnetic interactions. Furthermore, the process of alloying—combining different metals—can alter the magnetic properties of the resulting material, sometimes rendering it non-magnetic. By examining these three aspects—atomic electron configuration, crystal structure, and the influence of alloying—we can gain a comprehensive understanding of why some metals lack magnetic properties. Let's begin by exploring the fundamental relationship between atomic electron configuration and magnetism, which sets the stage for understanding the broader mechanisms at play.
Atomic Electron Configuration and Magnetism
Atomic electron configuration plays a crucial role in determining the magnetic properties of metals. To understand why some metals are not magnetic, it is essential to delve into the arrangement of electrons within their atoms. In atomic physics, electrons are arranged in specific energy levels or shells around the nucleus. Each shell is further divided into subshells, and these subshells can hold a specific number of electrons. The magnetic behavior of an atom is primarily influenced by the unpaired electrons in its outermost shell. When electrons occupy orbitals in pairs, their spins are paired in opposite directions, resulting in no net magnetic moment. However, if there are unpaired electrons, their spins contribute to a net magnetic moment, making the atom paramagnetic or ferromagnetic under certain conditions. In metals like copper, silver, and gold, the outermost energy level is fully occupied with paired electrons, leading to no unpaired electrons and thus no net magnetic moment. This full occupancy explains why these metals are diamagnetic, meaning they are weakly repelled by magnetic fields but do not exhibit any significant magnetic properties themselves. Another factor influencing magnetism is the electron configuration of the d and f subshells. Transition metals, which include many ferromagnetic materials like iron, nickel, and cobalt, have partially filled d subshells. These unpaired d electrons are responsible for their strong magnetic properties. In contrast, metals like zinc and cadmium have fully filled d subshells with no unpaired electrons, rendering them non-magnetic. The crystal structure of metals also affects their magnetic behavior. For instance, certain metals may exhibit different magnetic properties depending on their allotropes or crystal forms. However, the fundamental reason for non-magnetism in metals like aluminum and tin lies in their atomic electron configuration where all outer electrons are paired. In summary, the absence of unpaired electrons in the outermost energy levels of certain metals is the primary reason they do not exhibit significant magnetic properties. Understanding atomic electron configuration provides a clear insight into why some metals remain non-magnetic while others display strong ferromagnetic or paramagnetic behaviors. This knowledge is crucial for selecting materials with specific properties for various technological applications, from electrical wiring to advanced magnetic storage devices.
Role of Crystal Structure in Magnetism
The role of crystal structure in magnetism is pivotal in understanding why some metals are not magnetic. In metals, magnetism arises from the alignment of electron spins, which can be influenced significantly by the arrangement of atoms within the crystal lattice. The crystal structure determines how electrons interact with each other and their surroundings, thereby affecting the magnetic properties of a material. For instance, ferromagnetic materials like iron and nickel exhibit strong magnetic behavior due to their specific crystal structures that allow for the alignment of electron spins. In contrast, metals with different crystal structures may not support such alignment, leading to non-magnetic or weakly magnetic behavior. In metals like copper and silver, which have face-centered cubic (FCC) crystal structures, the electron spins are not aligned in a way that would produce significant magnetism. This is because the FCC structure does not favor the parallel alignment of electron spins necessary for ferromagnetism. Instead, these metals tend to exhibit diamagnetic or paramagnetic behavior, where they are weakly repelled by magnetic fields or show no permanent magnetization, respectively. Another critical aspect is the concept of orbital overlap and hybridization in different crystal structures. In some metals, the overlap of atomic orbitals and the resulting hybridization can lead to a situation where electron spins are paired, reducing the net magnetic moment. For example, in metals with body-centered cubic (BCC) structures like chromium, the specific arrangement of atoms can result in antiferromagnetic behavior where adjacent spins are aligned antiparallel, canceling out the net magnetization. Furthermore, the presence of impurities or defects in the crystal structure can also disrupt the magnetic order, leading to non-magnetic behavior. In pure metals, even slight deviations from the ideal crystal structure due to impurities or lattice defects can significantly alter their magnetic properties. In summary, the crystal structure plays a crucial role in determining whether a metal is magnetic or not. The specific arrangement of atoms in the lattice influences electron spin alignment, orbital overlap, and hybridization, all of which are critical factors in the manifestation of magnetism. Understanding these relationships is essential for explaining why some metals exhibit strong magnetic properties while others do not. This fundamental knowledge also underpins the development of new materials with tailored magnetic properties for various technological applications.
Influence of Alloying on Magnetic Properties
The influence of alloying on magnetic properties is a critical factor in understanding why some metals are not magnetic. When pure metals are alloyed with other elements, their magnetic behavior can undergo significant changes. This is because the introduction of foreign atoms into the crystal lattice of the base metal can alter the electronic structure and the interactions between magnetic moments. For instance, iron, a ferromagnetic metal, loses its magnetic properties when alloyed with certain elements like chromium or nickel in specific proportions. In the case of stainless steel, which is primarily an iron-chromium alloy, the addition of chromium disrupts the long-range magnetic order necessary for ferromagnetism, resulting in a non-magnetic material. Similarly, nickel, when added to iron in sufficient amounts, can also suppress its magnetic properties due to changes in the electron spin alignment and exchange interactions. Alloying can also introduce defects and impurities that disrupt the magnetic domains within a metal, leading to a reduction or complete loss of magnetism. For example, the presence of carbon in iron can form carbides that act as non-magnetic inclusions, thereby weakening the overall magnetic field. Furthermore, some alloys exhibit antiferromagnetic or paramagnetic behavior due to the specific arrangement of their electron spins. In antiferromagnetic materials, the magnetic moments align in a way that cancels out the net magnetization, while in paramagnetic materials, the moments are randomly aligned and do not contribute to a net magnetic field. The impact of alloying on magnetic properties is not limited to ferromagnetic metals; it also affects other types of magnetic materials. For instance, certain rare-earth alloys are known for their unique magnetic characteristics, which can be tailored by adjusting the composition. However, these alloys often lose their magnetic properties when exposed to high temperatures or when alloyed with non-magnetic elements. Understanding the influence of alloying on magnetic properties is crucial for various technological applications. In engineering, the ability to control and manipulate the magnetic behavior of materials through alloying allows for the development of advanced magnetic materials with specific properties. For example, magnetic alloys used in electrical motors and generators must be carefully designed to optimize their performance under different operating conditions. Similarly, in biomedical applications, non-magnetic alloys are preferred for implants to avoid interference with MRI scans. In conclusion, the effect of alloying on magnetic properties is a complex phenomenon that significantly influences whether a metal exhibits magnetism or not. By carefully selecting and combining different elements, engineers and materials scientists can create alloys with tailored magnetic characteristics, which is essential for a wide range of industrial and technological applications. This understanding underscores why some metals, when alloyed, lose their inherent magnetic properties, contributing to the broader discussion on why some metals are not magnetic.