What Is Static Pressure
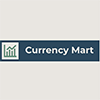
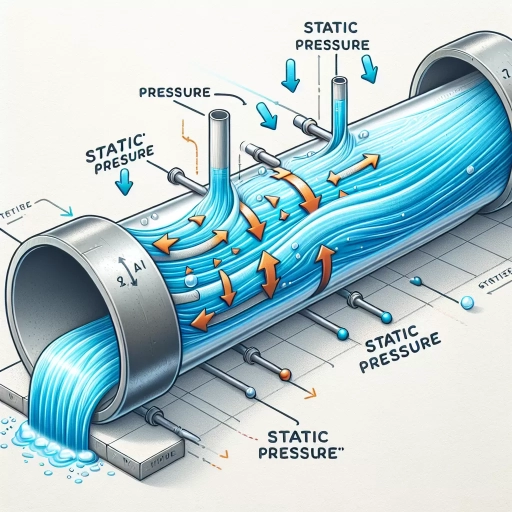
Static pressure is a fundamental concept in various fields, including engineering, physics, and everyday life. It is the pressure exerted by a fluid at equilibrium at any point of the fluid due to the force of gravity. Understanding static pressure is crucial for designing and operating systems that involve fluids, such as water supply networks, HVAC systems, and industrial processes. This article delves into the intricacies of static pressure, starting with the basics of what it is and how it is calculated. We will then explore its applications and importance across different sectors, highlighting its role in ensuring efficient and safe operations. Finally, we will discuss methods for measuring and managing static pressure to optimize system performance. By grasping these key aspects, readers will gain a comprehensive understanding of static pressure and its significance. Let's begin by understanding the basics of static pressure.
Understanding Static Pressure Basics
Understanding static pressure is crucial for various fields, including engineering, architecture, and HVAC systems. Static pressure, a fundamental concept in fluid dynamics, refers to the pressure exerted by a fluid at equilibrium at any point of the fluid due to the force of gravity. To delve into this topic comprehensively, it is essential to explore three key aspects: the definition and concept of static pressure, the units of measurement used to quantify it, and the key factors that influence its magnitude. Starting with the **Definition and Concept**, it is vital to grasp what static pressure entails and how it differs from other types of pressure. This foundational understanding sets the stage for more advanced discussions. Next, **Units of Measurement** are critical as they standardize how static pressure is expressed, allowing for consistent communication and calculation across different contexts. Finally, **Key Factors Influencing Static Pressure** highlight the variables that affect its value, such as elevation, fluid density, and external forces. By examining these three components, one can gain a thorough understanding of static pressure and its practical applications. Let us begin by exploring the **Definition and Concept** of static pressure in more detail.
Definition and Concept
**Definition and Concept** Static pressure, a fundamental concept in fluid dynamics and HVAC systems, refers to the pressure exerted by a fluid (liquid or gas) at equilibrium, meaning when it is not moving. This type of pressure is a measure of the force exerted per unit area on an object or surface due to the weight of the fluid above it. Unlike dynamic pressure, which is associated with the motion of fluids, static pressure is present even when there is no flow. In practical terms, static pressure is what you would measure if you were to insert a pressure gauge into a stationary fluid. Understanding static pressure is crucial in various engineering fields. For instance, in heating, ventilation, and air conditioning (HVAC) systems, static pressure plays a key role in determining the performance and efficiency of air handlers, fans, and ductwork. It helps engineers design systems that can effectively distribute air throughout buildings without causing undue strain on the equipment. In hydraulic systems, static pressure is essential for calculating the head (vertical distance) that a pump must overcome to move fluid from one point to another. The concept of static pressure can also be applied to atmospheric conditions. Atmospheric pressure, which is the pressure exerted by the weight of the air in the Earth's atmosphere, is a form of static pressure. This pressure decreases with altitude because there is less air above to exert weight. Understanding these principles allows meteorologists to predict weather patterns and engineers to design structures that can withstand various environmental conditions. In scientific terms, static pressure can be calculated using the formula \(P = \rho \times g \times h\), where \(P\) is the static pressure, \(\rho\) is the density of the fluid, \(g\) is the acceleration due to gravity, and \(h\) is the height of the fluid column above the point of measurement. This formula highlights how static pressure increases linearly with depth in a fluid. In summary, static pressure is a critical concept that underpins many engineering and scientific applications. It provides a baseline understanding of how fluids behave under equilibrium conditions and is essential for designing efficient systems in HVAC, hydraulic engineering, and other fields. By grasping this fundamental principle, professionals can ensure that their designs are robust, efficient, and capable of withstanding various operational demands.
Units of Measurement
Understanding the units of measurement is crucial for grasping static pressure basics, as it allows for accurate calculations and comparisons in various engineering and scientific contexts. Static pressure, a fundamental concept in fluid dynamics and HVAC systems, is typically measured in units that reflect the force exerted per unit area. The most common units include Pascals (Pa), pounds per square inch (psi), and millimeters of mercury (mmHg). **Pascals (Pa)** are part of the International System of Units (SI) and are defined as one Newton per square meter. This unit is widely used in scientific and engineering applications due to its simplicity and coherence with other SI units. For instance, atmospheric pressure at sea level is approximately 101,325 Pa, which provides a baseline for understanding static pressure in different environments. **Pounds per square inch (psi)** is a unit commonly used in the United States and other countries that still employ imperial measurements. One psi is equivalent to 6894.76 Pa, making it a practical unit for many industrial and commercial applications. In HVAC systems, psi is often used to measure the pressure drop across filters or the static pressure within ducts. **Millimeters of mercury (mmHg)**, also known as millimeters of Hg, is another unit that measures pressure based on the height of a mercury column. This unit is frequently used in medical contexts, such as blood pressure measurements, but it can also be applied to static pressure calculations. One mmHg is equivalent to 133.322 Pa, providing a precise way to express small changes in pressure. Understanding these units is essential because static pressure can vary significantly depending on the context. For example, in HVAC systems, static pressure might range from a few hundred Pascals to several thousand Pascals, while in medical settings, blood pressure is typically measured in mmHg. The ability to convert between these units ensures that engineers and technicians can communicate effectively and make accurate assessments. Moreover, the choice of unit can influence the design and operation of systems. For instance, when designing an HVAC system, engineers must consider the static pressure losses due to ductwork, fittings, and filters to ensure optimal airflow and energy efficiency. Using consistent units throughout these calculations helps in predicting performance and troubleshooting issues. In summary, mastering the units of measurement for static pressure—whether it be Pascals, psi, or mmHg—is vital for understanding and working with static pressure basics. These units provide a common language across different fields, enabling precise calculations and effective communication among professionals. By recognizing the significance of each unit and how they interrelate, one can better comprehend the complexities of static pressure and its applications in various engineering and scientific disciplines.
Key Factors Influencing Static Pressure
Static pressure, a fundamental concept in fluid dynamics and HVAC systems, is influenced by several key factors that determine its magnitude and impact. **Density of the Fluid** is one of the primary factors; the higher the density of the fluid, the greater the static pressure. For instance, in HVAC systems, air density varies with temperature and humidity, affecting the overall static pressure within ducts. **Elevation** also plays a crucial role, as static pressure increases with altitude due to the weight of the fluid above. This is particularly relevant in tall buildings or mountainous regions where atmospheric pressure changes significantly with height. **Temperature** is another critical factor, as it affects the density of gases. In HVAC systems, warmer air is less dense than cooler air, which can alter static pressure readings. For example, in summer months when air is warmer, static pressure might be lower compared to winter months when air is cooler and denser. **Humidity** also impacts static pressure by altering air density; higher humidity levels make air less dense, thereby reducing static pressure. The **shape and size of ducts** in HVAC systems are additional influential factors. Narrower or more complex ducts can increase static pressure due to increased resistance to airflow. Similarly, **obstructions** within ducts, such as bends, elbows, or filters, can significantly elevate static pressure by creating turbulence and resistance. The **fan or blower characteristics**, including speed and efficiency, also play a crucial role in determining static pressure. A more powerful fan can generate higher static pressure to overcome resistance in the system. Furthermore, **external environmental conditions** such as wind and atmospheric pressure can affect static pressure in outdoor applications. For example, high winds can increase the static pressure on building exteriors, while changes in atmospheric pressure can influence indoor static pressures if there are leaks or openings in the building envelope. Understanding these factors is essential for designing and maintaining efficient HVAC systems and ensuring optimal performance. By considering these variables, engineers and technicians can accurately calculate and manage static pressure to achieve better airflow, reduce energy consumption, and enhance overall system reliability. This comprehensive approach not only ensures that systems operate within specified parameters but also helps in troubleshooting issues related to static pressure imbalances. In summary, recognizing the interplay between these key factors is vital for mastering static pressure basics and optimizing system performance across various applications.
Applications and Importance of Static Pressure
Static pressure plays a crucial role in various aspects of modern engineering and architecture, making it a fundamental concept to understand for both practitioners and enthusiasts. This article delves into the multifaceted applications and importance of static pressure, highlighting its significance in three key areas: HVAC systems, building design and construction, and industrial processes. In HVAC systems, static pressure is essential for ensuring the efficient distribution of air throughout a building, directly impacting the comfort and health of occupants. The design and construction of buildings also rely heavily on static pressure to optimize ventilation, insulation, and overall structural integrity. Additionally, industrial processes utilize static pressure to maintain precise environmental conditions necessary for manufacturing and production. By exploring these dimensions, we gain a comprehensive understanding of how static pressure underpins many critical systems. Let us begin by examining its pivotal role in HVAC systems, where the precise management of static pressure is crucial for maintaining optimal indoor air quality and system performance.
Role in HVAC Systems
In the realm of Heating, Ventilation, and Air Conditioning (HVAC) systems, static pressure plays a pivotal role in ensuring the efficient and effective operation of these systems. Static pressure, which is the measure of the pressure exerted by a fluid (air or water) at rest, is crucial for maintaining optimal airflow and system performance. Within HVAC systems, static pressure influences several key aspects: **air distribution**, **system design**, **energy efficiency**, and **overall system reliability**. Firstly, static pressure is essential for **air distribution**. It determines how air moves through ducts and vents to reach various parts of a building. Properly managed static pressure ensures that air is delivered uniformly, preventing areas from becoming too hot or too cold. This balance is particularly important in commercial and industrial settings where consistent indoor climate conditions are vital for comfort and productivity. Secondly, static pressure significantly impacts **system design**. Engineers must consider static pressure when designing ductwork and selecting fans or blowers. Incorrectly calculated static pressure can lead to oversized or undersized components, resulting in inefficiencies and potential system failures. For instance, if the static pressure is too high, it may necessitate more powerful fans, which could increase energy consumption and operational costs. Thirdly, static pressure directly affects **energy efficiency**. High static pressure can cause HVAC systems to work harder, leading to increased energy consumption and higher utility bills. Conversely, optimizing static pressure through proper system design and maintenance can reduce energy usage, making the system more sustainable and cost-effective. This is particularly relevant in modern buildings where energy efficiency is a key consideration due to environmental concerns and regulatory requirements. Lastly, static pressure contributes to **overall system reliability**. Imbalances in static pressure can lead to premature wear on system components, such as fans and compressors, reducing their lifespan and increasing maintenance needs. By monitoring and adjusting static pressure, HVAC technicians can identify potential issues before they become major problems, ensuring that the system operates smoothly and reliably over its intended lifespan. In summary, static pressure is a fundamental parameter in HVAC systems that affects air distribution, system design, energy efficiency, and overall reliability. Understanding and managing static pressure is essential for maintaining optimal performance, reducing operational costs, and ensuring the longevity of HVAC components. As such, it remains a critical aspect of HVAC system design and operation, underscoring its importance in both residential and commercial applications.
Impact on Building Design and Construction
The impact of static pressure on building design and construction is multifaceted and crucial, influencing various aspects from structural integrity to indoor air quality. Static pressure, the force exerted by a fluid (such as air or water) at equilibrium, plays a significant role in ensuring the stability and functionality of buildings. In terms of structural design, architects must consider static pressure to ensure that buildings can withstand external forces like wind and water. For instance, the design of roofs and walls must account for the static pressure exerted by wind, which can vary significantly depending on the building's height, location, and surrounding environment. This consideration helps in preventing structural failures and maintaining the overall safety of the building. In addition to structural considerations, static pressure is vital in the design of HVAC (heating, ventilation, and air conditioning) systems. Proper management of static pressure within these systems ensures efficient airflow, which is essential for maintaining a comfortable indoor environment. Incorrect static pressure can lead to reduced system performance, increased energy consumption, and compromised indoor air quality. For example, if the static pressure in an HVAC system is too high, it can cause the system to work harder than necessary, leading to increased energy costs and potential system failures. Conversely, if the static pressure is too low, it may result in inadequate airflow, affecting the distribution of heated or cooled air throughout the building. Furthermore, static pressure impacts the design of plumbing systems. In water supply systems, static pressure determines the water pressure at different points within the system. This is critical for ensuring that water flows adequately to all parts of the building without causing excessive pressure that could lead to pipe bursts or leaks. Similarly, in drainage systems, understanding static pressure helps in designing slopes and gradients that facilitate smooth flow of wastewater without causing backups or siphoning issues. From an energy efficiency perspective, managing static pressure is key to optimizing building performance. Buildings with well-designed HVAC systems that maintain optimal static pressure levels can achieve significant reductions in energy consumption. This not only helps in lowering operational costs but also contributes to a more sustainable built environment by reducing greenhouse gas emissions associated with energy use. In summary, the impact of static pressure on building design and construction is profound. It influences structural stability, HVAC system efficiency, plumbing system functionality, and overall energy performance. By understanding and effectively managing static pressure, architects and engineers can create buildings that are safer, more comfortable, and more sustainable. This underscores the importance of static pressure in the broader context of building design and construction, highlighting its role as a critical factor that must be carefully considered to achieve optimal building performance.
Relevance in Industrial Processes
In industrial processes, relevance is a critical factor that directly impacts efficiency, safety, and overall performance. Static pressure, a fundamental concept in fluid dynamics, plays a pivotal role in various industrial applications. Understanding and managing static pressure is essential for optimizing system design, ensuring reliable operation, and minimizing risks. For instance, in HVAC systems, static pressure determines the airflow distribution and system balance. Incorrect static pressure can lead to inadequate ventilation, reduced system efficiency, and increased energy consumption. In chemical processing plants, static pressure is crucial for maintaining the integrity of pipelines and vessels, preventing leaks or ruptures that could have catastrophic consequences. Similarly, in oil and gas industries, accurate measurement and control of static pressure are vital for drilling operations and pipeline management to avoid blowouts or other safety hazards. Additionally, in manufacturing facilities, static pressure is used to control the flow of materials through pneumatic conveyance systems, ensuring consistent product quality and reducing downtime. The importance of static pressure extends to water treatment plants where it affects the performance of pumps and filters, impacting water quality and public health. Furthermore, in aerospace engineering, static pressure measurements are critical for testing and validating aircraft designs under various environmental conditions. By accurately measuring and managing static pressure, industries can enhance operational reliability, reduce maintenance costs, and improve product quality. This underscores the significance of static pressure as a key parameter in industrial processes, highlighting its relevance across diverse sectors and applications.
Measuring and Managing Static Pressure
Measuring and managing static pressure is a critical aspect of maintaining efficient and effective HVAC systems. Static pressure, the force exerted by air within a duct system, directly impacts airflow, energy consumption, and overall system performance. To ensure optimal operation, it is essential to understand the tools and techniques for accurate measurement, adhere to best practices for balancing systems, and address common challenges that may arise. Accurate measurement of static pressure is the foundation of any well-functioning HVAC system. This involves using specialized tools such as manometers and pressure sensors to gauge pressure levels at various points within the ductwork. By employing these tools effectively, technicians can identify areas of high or low pressure, which is crucial for system optimization. In addition to precise measurement, best practices for balancing systems are vital. This includes ensuring that air distribution is even throughout the system, adjusting dampers and fans to achieve optimal airflow, and regularly inspecting and maintaining components to prevent degradation. However, common challenges such as duct leaks, improper installation, and wear and tear on components can disrupt system balance. Understanding these challenges and implementing solutions like sealing leaks, recalibrating sensors, and performing routine maintenance can help mitigate these issues. By mastering these elements—tools and techniques for measurement, best practices for balancing systems, and solutions to common challenges—HVAC professionals can ensure that their systems operate at peak efficiency. Let's delve into the first of these critical components: **Tools and Techniques for Measurement**.
Tools and Techniques for Measurement
When it comes to measuring and managing static pressure, having the right tools and techniques is paramount. Static pressure, a critical parameter in various fields such as HVAC, aerospace, and industrial processes, requires precise measurement to ensure optimal performance and safety. Here are some of the key tools and techniques used for this purpose. **Tools:** 1. **Manometers:** These are primary instruments for measuring static pressure. Digital manometers offer high accuracy and ease of use, while analog manometers provide a visual gauge that can be read directly. 2. **Pressure Gauges:** These devices are mounted directly on systems to provide real-time readings of static pressure. They come in various types, including mechanical and electronic gauges. 3. **Data Loggers:** These devices record pressure readings over time, allowing for detailed analysis of pressure trends and fluctuations. 4. **Barometers:** While primarily used for atmospheric pressure measurement, barometers can also be adapted to measure static pressure in specific applications. **Techniques:** 1. **Direct Measurement:** This involves attaching a pressure gauge or manometer directly to the system being measured. It is straightforward but requires careful placement to avoid interference. 2. **Indirect Measurement:** This method uses secondary indicators such as flow rates or temperature changes to infer static pressure. It is useful when direct measurement is impractical. 3. **Calibration:** Regular calibration of measurement tools is essential to ensure accuracy. This involves comparing readings against known standards. 4. **Data Analysis:** Advanced software tools can analyze data from multiple sensors to provide a comprehensive view of static pressure across different parts of a system. **Best Practices:** - **Proper Installation:** Ensure that measurement devices are installed correctly to avoid errors due to misalignment or obstruction. - **Regular Maintenance:** Regularly inspect and maintain measurement tools to prevent drift or malfunction. - **Standardization:** Use standardized procedures and tools to ensure consistency in measurements across different locations and personnel. By leveraging these tools and techniques, professionals can accurately measure and manage static pressure, leading to improved system efficiency, reduced energy consumption, and enhanced safety. For instance, in HVAC systems, precise static pressure measurements help in optimizing airflow, which can significantly reduce energy costs and improve indoor air quality. Similarly, in aerospace applications, accurate static pressure measurements are crucial for ensuring the structural integrity and performance of aircraft components. By combining the right tools with best practices in measurement and analysis, professionals can achieve reliable and actionable data that supports informed decision-making and optimal system performance.
Best Practices for Balancing Systems
When it comes to measuring and managing static pressure, one of the critical components is ensuring that the HVAC system is properly balanced. Balancing systems involves adjusting the airflow, water flow, or other fluid dynamics within the system to optimize performance, efficiency, and comfort. Here are some best practices for balancing systems: 1. **Initial System Design**: Start with a well-designed system. Ensure that ductwork, piping, and other components are sized correctly to handle the intended load. This foundational step minimizes the need for extensive adjustments later on. 2. **Commissioning**: Commission the system thoroughly at the outset. This involves verifying that all components are installed correctly and functioning as intended. Commissioning includes checking for leaks, ensuring proper insulation, and validating that control systems are calibrated. 3. **Regular Maintenance**: Regular maintenance is crucial for maintaining balance. Schedule periodic checks to ensure that filters are clean, coils are free of debris, and fans are operating at their designed speeds. Dirty filters or clogged coils can significantly alter system performance. 4. **Airflow Measurement**: Use accurate tools to measure airflow. Balometers or anemometers can provide precise readings of airflow rates at various points in the system. This data helps in identifying imbalances and making necessary adjustments. 5. **Zone Control**: Implement zone control systems where possible. These allow for more precise control over different areas within a building, ensuring that each zone receives the appropriate amount of conditioned air or water without overloading the system. 6. **Dampers and Valves**: Use dampers and valves strategically to regulate airflow and fluid flow. These devices should be adjusted carefully to achieve the desired balance without causing undue pressure drops or energy losses. 7. **System Monitoring**: Install monitoring systems that provide real-time data on system performance. This includes pressure sensors, flow meters, and temperature sensors. Continuous monitoring helps in identifying any deviations from optimal conditions promptly. 8. **Training and Documentation**: Ensure that maintenance personnel are trained in balancing techniques and have access to detailed documentation of the system's design and operation. This knowledge base is essential for making informed adjustments and troubleshooting issues efficiently. 9. **Energy Efficiency**: Balance the system with energy efficiency in mind. Proper balancing can lead to significant reductions in energy consumption by ensuring that the system operates within its optimal parameters. This not only saves energy but also extends the lifespan of equipment. 10. **Feedback Loop**: Establish a feedback loop between the balancing process and system performance metrics. Use data from monitoring systems to adjust the balance as needed, creating a continuous cycle of improvement. By adhering to these best practices, you can ensure that your HVAC or fluid-based systems operate at peak efficiency, providing consistent comfort while minimizing energy costs and extending equipment life. Effective balancing is a key component of managing static pressure, as it directly impacts the overall performance and health of the system.
Common Challenges and Solutions
When it comes to measuring and managing static pressure, several common challenges often arise that can significantly impact the accuracy and efficiency of HVAC systems. One of the primary challenges is **inaccurate measurement**, which can stem from improper placement of pressure sensors or the use of low-quality equipment. To address this, it is crucial to ensure that sensors are strategically located to capture accurate readings and that they are calibrated regularly. Additionally, investing in high-quality, precision instruments can help mitigate errors. Another challenge is **leaks in the ductwork**, which can lead to significant losses in static pressure and overall system performance. Identifying and sealing these leaks is essential; this can be achieved through thorough inspections using tools such as smoke pens or duct leakage testers. Regular maintenance schedules should include duct inspections to prevent such issues from arising. **System imbalance** is another prevalent issue, where the airflow distribution is not evenly matched with the system's design specifications. This imbalance can cause uneven static pressure across different parts of the system, leading to reduced efficiency and increased energy consumption. To solve this, technicians should perform thorough system balancing by adjusting dampers and fans to ensure that airflow matches the design requirements. **Filter maintenance** also plays a critical role in managing static pressure. Dirty or clogged filters can increase resistance to airflow, thereby increasing static pressure and reducing system efficiency. Regular filter cleaning or replacement according to the manufacturer's guidelines can help maintain optimal airflow and reduce static pressure. Furthermore, **system design flaws** can be a significant challenge. If the system is not designed with proper considerations for static pressure, it may lead to persistent issues regardless of maintenance efforts. In such cases, consulting with experienced HVAC engineers to redesign or modify the system can be necessary to achieve optimal performance. Lastly, **lack of monitoring** can exacerbate these challenges by allowing issues to go undetected until they become severe. Implementing real-time monitoring systems that track static pressure and other key metrics can help identify potential problems early on, enabling proactive maintenance and minimizing downtime. In summary, addressing common challenges in measuring and managing static pressure involves a combination of accurate measurement techniques, regular maintenance practices, system balancing, proper filter maintenance, addressing design flaws, and continuous monitoring. By focusing on these areas, HVAC professionals can ensure that their systems operate efficiently, reducing energy costs and enhancing overall performance.