What Is Lte+
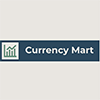
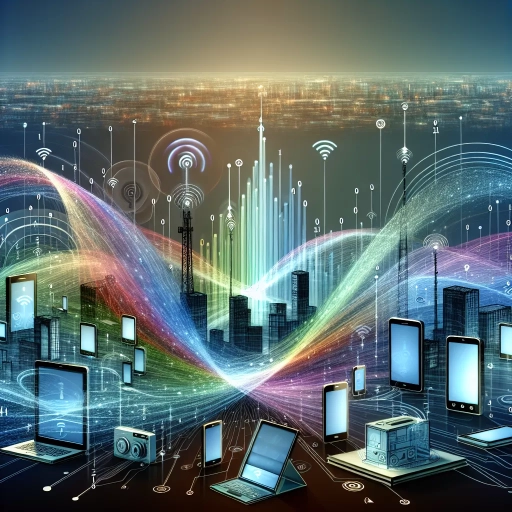
In the ever-evolving landscape of wireless communication, LTE+ (Long-Term Evolution Plus) stands as a pinnacle of technological advancement, offering unparalleled speed, reliability, and connectivity. This high-performance network standard has revolutionized the way we communicate, access data, and utilize mobile services. To fully appreciate the significance of LTE+, it is essential to delve into its underlying technology, operational mechanisms, and the profound impact it has on various aspects of our lives. This article will explore these facets in detail, beginning with an in-depth look at **Understanding LTE Technology**, which lays the foundation for comprehending the intricacies of this advanced network. From there, we will examine **How LTE Works**, uncovering the technical processes that enable its remarkable performance. Finally, we will discuss **Applications and Impact of LTE**, highlighting how this technology has transformed industries and daily life. By understanding these key components, readers will gain a comprehensive insight into the world of LTE+ and its transformative power. Let us start by **Understanding LTE Technology**.
Understanding LTE Technology
Understanding LTE (Long-Term Evolution) technology is crucial in today's fast-paced digital world, where high-speed data transmission and reliable connectivity are paramount. LTE represents a significant leap forward in wireless communication, offering substantial improvements over its predecessors. To fully grasp the intricacies of LTE, it is essential to delve into its definition and basic principles, which form the foundation of this advanced technology. Additionally, understanding the evolutionary journey from earlier networks such as 2G and 3G provides valuable context on how LTE has addressed previous limitations. Finally, exploring the key features and advantages of LTE highlights its capabilities and why it has become a cornerstone of modern telecommunications. By examining these aspects, we can appreciate the robustness and efficiency that LTE brings to our daily lives. Let us begin by defining and exploring the basics of LTE, which will set the stage for a deeper understanding of its evolution and benefits.
Definition and Basics of LTE
**Definition and Basics of LTE** Long-Term Evolution (LTE) is a wireless communication standard for mobile devices and data terminals, designed to provide high-speed data and voice services. It is the fourth generation (4G) of wireless mobile telecommunications technology, succeeding 3G networks. LTE was developed by the 3rd Generation Partnership Project (3GPP), an international collaboration of telecommunications companies and organizations. The primary goal of LTE is to offer faster data rates, lower latency, and greater capacity compared to its predecessors. At its core, LTE operates on a flat, all-IP (Internet Protocol) network architecture, which simplifies the infrastructure and enhances efficiency. This architecture eliminates the need for circuit-switched networks, allowing for more flexible and efficient use of bandwidth. LTE supports both Frequency Division Duplexing (FDD) and Time Division Duplexing (TDD) modes, enabling it to adapt to various frequency bands and deployment scenarios. Key features of LTE include its ability to support peak download speeds of up to 100 Mbps and upload speeds of up to 50 Mbps in its initial release, with subsequent releases like LTE-Advanced pushing these limits even further. For instance, LTE-Advanced can achieve peak download speeds of up to 1 Gbps. Additionally, LTE boasts low latency, typically around 50 ms, which is crucial for real-time applications such as video streaming and online gaming. The technology also introduces several innovative concepts such as Orthogonal Frequency Division Multiple Access (OFDMA) for downlink and Single Carrier Frequency Division Multiple Access (SC-FDMA) for uplink. These techniques enable efficient use of spectrum and reduce interference among users. Furthermore, LTE incorporates advanced antenna technologies like Multiple Input Multiple Output (MIMO), which significantly enhances data throughput and coverage. In terms of network architecture, LTE consists of two main components: the Evolved Universal Terrestrial Radio Access Network (E-UTRAN) and the Evolved Packet Core (EPC). E-UTRAN handles the radio access part, while EPC manages the core network functions such as mobility management, session management, and bearer management. This separation allows for greater flexibility in network design and operation. The adoption of LTE has been widespread globally due to its robust performance, scalability, and the ability to support a wide range of services including voice over LTE (VoLTE), video conferencing, and IoT applications. As mobile networks continue to evolve with the advent of 5G technology, LTE remains a critical component in providing seamless connectivity and laying the groundwork for future advancements in wireless communication. Understanding these basics of LTE technology is essential for appreciating its role in modern telecommunications and how it has paved the way for subsequent generations of wireless networks. By leveraging its high-speed data capabilities and efficient network architecture, LTE has transformed the way we communicate and access information on the go.
Evolution from Previous Networks (3G, 2G)
The evolution of wireless communication networks from 2G and 3G to LTE (Long-Term Evolution) is a testament to the relentless pursuit of faster, more reliable, and more versatile mobile connectivity. The journey began with 2G (Second Generation) networks, which introduced digital voice services and basic data capabilities such as SMS and low-speed internet access. However, these networks were limited by their narrow bandwidth and lack of support for high-speed data transmission. The advent of 3G (Third Generation) networks marked a significant leap forward, offering higher data speeds and enabling multimedia services like video calling and mobile broadband. Despite these advancements, 3G networks still faced challenges related to latency, capacity, and the increasing demand for mobile data. The transition to LTE was driven by the need for even faster speeds, lower latency, and greater network efficiency. LTE technology leverages advanced radio access techniques such as Orthogonal Frequency Division Multiple Access (OFDMA) and Single-Carrier Frequency Division Multiple Access (SC-FDMA), which optimize spectrum usage and enhance overall network performance. Unlike its predecessors, LTE is designed to support a wide range of applications, from real-time video streaming to cloud computing, by providing peak download speeds that can exceed 100 Mbps. This capability is crucial in today's data-intensive environment where users expect seamless connectivity for various services including social media, online gaming, and IoT (Internet of Things) applications. Moreover, LTE's architecture is inherently more flexible and scalable compared to earlier generations. It adopts an all-IP (Internet Protocol) network architecture that simplifies network management and reduces operational costs. This design also facilitates easier integration with other technologies such as Wi-Fi and fixed broadband networks, enabling a more unified and robust communication ecosystem. Additionally, LTE's support for Quality of Service (QoS) mechanisms ensures that different types of traffic are prioritized appropriately, ensuring that critical applications receive the necessary bandwidth and latency guarantees. The evolution from 2G and 3G to LTE has also been influenced by regulatory and market pressures. As mobile devices became increasingly sophisticated and user expectations grew, telecommunications standards bodies like the 3GPP (3rd Generation Partnership Project) played a pivotal role in defining the technical specifications for LTE. This collaborative effort ensured that LTE technology could be widely adopted across different regions and operators, fostering global interoperability and economies of scale. In summary, the transition from 2G and 3G networks to LTE represents a significant milestone in the history of wireless communication. Driven by technological innovations and market demands, LTE offers unparalleled performance in terms of speed, latency, and capacity. As we continue to push the boundaries of what is possible with mobile technology, understanding the evolution from previous networks provides valuable insights into how LTE has become the cornerstone of modern mobile communication systems. This foundational knowledge is essential for appreciating the capabilities and limitations of LTE technology as we move forward into an era dominated by 5G and beyond.
Key Features and Advantages
**Key Features and Advantages** Long-Term Evolution (LTE) technology, a cornerstone of modern wireless communication, boasts several key features that underpin its widespread adoption and success. One of the most significant advantages of LTE is its **high data transfer speeds**, enabling users to enjoy seamless video streaming, rapid file downloads, and real-time online gaming. This is achieved through the use of advanced modulation techniques such as OFDMA (Orthogonal Frequency Division Multiple Access) and SC-FDMA (Single Carrier Frequency Division Multiple Access), which optimize spectral efficiency and reduce interference. Another critical feature of LTE is its **low latency**, which is crucial for applications requiring real-time communication, such as voice over LTE (VoLTE) and mission-critical communications. The latency in LTE networks can be as low as 10 milliseconds, significantly improving the user experience compared to earlier technologies. Additionally, LTE's **scalability** allows it to support a large number of users and devices, making it an ideal choice for densely populated urban areas and IoT (Internet of Things) applications. The **flexibility** of LTE is another key advantage. It supports various frequency bands and can operate in different modes, including FDD (Frequency Division Duplexing) and TDD (Time Division Duplexing), allowing operators to choose the best configuration based on their specific needs. This flexibility also extends to the support for different bandwidths, ranging from 1.4 MHz to 20 MHz, which helps in optimizing network performance according to available spectrum. Moreover, LTE's **robust security features** ensure that data transmitted over the network is secure. It uses advanced encryption algorithms and secure authentication protocols to protect user data from unauthorized access. This is particularly important in today's digital age where data privacy is a top concern. From an operational standpoint, LTE offers **cost-effective deployment** options. The technology allows for the reuse of existing infrastructure, reducing the capital expenditure required for network rollout. Furthermore, LTE's **energy efficiency** helps in lowering operational costs by minimizing power consumption, which is beneficial both economically and environmentally. In summary, LTE's combination of high data speeds, low latency, scalability, flexibility, robust security, cost-effective deployment, and energy efficiency makes it a superior choice for modern wireless communication needs. These features collectively contribute to an enhanced user experience and provide a solid foundation for the evolution of wireless technology into 5G and beyond. Understanding these key features and advantages is essential for appreciating the full potential of LTE technology and its role in shaping the future of telecommunications.
How LTE Works
Long-Term Evolution (LTE) is a cutting-edge wireless communication standard that has revolutionized the way we access and utilize mobile data. At its core, LTE's efficiency and reliability are rooted in three key aspects: its robust network architecture and components, the sophisticated mechanisms of data transmission and signal processing, and the stringent quality of service (QoS) and resource allocation protocols. Understanding how LTE works begins with a deep dive into its **Network Architecture and Components**. This includes the Evolved Universal Terrestrial Radio Access Network (E-UTRAN) and the Evolved Packet Core (EPC), which together form the backbone of LTE's infrastructure. The E-UTRAN consists of eNodeBs that manage radio resources, while the EPC handles tasks such as mobility management and bearer establishment. Beyond architecture, **Data Transmission and Signal Processing** play crucial roles in ensuring high-speed data delivery. LTE employs advanced techniques like Orthogonal Frequency Division Multiple Access (OFDMA) and Single-Carrier Frequency Division Multiple Access (SC-FDMA) to optimize data transmission. Additionally, signal processing algorithms enhance the quality and reliability of data streams. Finally, **Quality of Service (QoS) and Resource Allocation** are essential for maintaining the performance and fairness of the network. QoS policies ensure that different types of traffic receive appropriate priority, while resource allocation mechanisms dynamically manage bandwidth to meet user demands. To delve deeper into these critical components, let's start by examining the **Network Architecture and Components** that underpin LTE's operational framework.
Network Architecture and Components
Network architecture and components are the backbone of any telecommunications system, including LTE (Long-Term Evolution). Understanding these elements is crucial for grasping how LTE works. At its core, LTE network architecture is designed to provide high-speed data and voice services efficiently. The architecture can be broadly divided into two main components: the Evolved Universal Terrestrial Radio Access Network (E-UTRAN) and the Evolved Packet Core (EPC). **E-UTRAN** is responsible for the radio access part of the network. It consists of eNodeBs (eNBs), which are essentially base stations that manage multiple cells. Each eNB connects directly to the EPC, eliminating the need for a separate Radio Network Controller (RNC) as seen in older 3G networks. This simplification reduces latency and enhances performance. The eNB handles tasks such as radio resource management, mobility control, and scheduling of user data. The **Evolved Packet Core (EPC)** is the central component that manages the core network functions. It includes several key entities: the Mobility Management Entity (MME), the Serving Gateway (S-GW), and the Packet Data Network Gateway (P-GW). The MME handles signaling and control functions like user authentication, bearer setup, and mobility management. The S-GW acts as a local mobility anchor for inter-eNB handovers and also performs lawful interception. The P-GW provides connectivity to external networks such as the internet and other IP networks, managing Quality of Service (QoS) policies. Another critical component is the **Home Subscriber Server (HSS)**, which acts as a central database containing user subscription information. It interacts with the MME to authenticate users and authorize access to network services. Additionally, there are other supporting elements like the Policy and Charging Rules Function (PCRF) that define QoS policies and charging rules based on subscriber profiles. In operation, when a user device connects to an LTE network, it first communicates with an eNB to establish a connection. The eNB then interacts with the MME in the EPC to authenticate the user using information from the HSS. Once authenticated, the MME sets up bearers through the S-GW and P-GW to allocate necessary resources for data transmission. This streamlined architecture ensures efficient data transfer with low latency, making LTE capable of delivering high-speed internet services such as video streaming and online gaming. In summary, LTE's network architecture is designed for efficiency and performance. The E-UTRAN handles radio access while the EPC manages core functions like user authentication, mobility control, and data routing. Each component works in harmony to provide seamless connectivity and high-speed data services that are characteristic of LTE technology. This robust architecture underpins how LTE works, enabling it to support a wide range of applications that demand reliable and fast connectivity.
Data Transmission and Signal Processing
Data transmission and signal processing are crucial components in the functioning of LTE (Long-Term Evolution) networks, ensuring efficient and reliable communication. At the heart of LTE's operation lies the complex interplay between these two processes. Data transmission in LTE involves the transfer of information from the user equipment (UE) to the base station (eNodeB) and vice versa. This process begins with data encoding, where raw data is converted into a format suitable for transmission. The encoded data is then modulated onto a carrier wave using techniques such as Quadrature Amplitude Modulation (QAM) or Quadrature Phase Shift Keying (QPSK), depending on the channel conditions and required data rate. Once modulated, the signal is processed through various stages of signal processing. This includes channel coding, which adds redundancy to the data to correct errors that may occur during transmission. The coded data is then mapped onto the available frequency resources using techniques like Orthogonal Frequency Division Multiple Access (OFDMA) for downlink and Single Carrier Frequency Division Multiple Access (SC-FDMA) for uplink. These techniques allow multiple users to share the same bandwidth efficiently by allocating different subcarriers to each user. Signal processing plays a pivotal role in enhancing the quality and reliability of the transmitted signal. In LTE, advanced signal processing algorithms are employed to mitigate interference and improve signal-to-noise ratio (SNR). Techniques such as beamforming and Multiple Input Multiple Output (MIMO) are used to focus the signal in specific directions, thereby increasing the signal strength and reducing interference. Additionally, adaptive modulation and coding (AMC) dynamically adjusts the modulation scheme and coding rate based on the current channel conditions to optimize throughput and minimize errors. Furthermore, LTE employs sophisticated receiver techniques like turbo decoding and iterative decoding to correct errors introduced during transmission. These techniques involve complex algorithms that iteratively refine the received signal to achieve high accuracy. The use of cyclic prefix in OFDMA helps in mitigating inter-symbol interference (ISI) caused by multipath propagation, ensuring that the received signal remains coherent. In summary, the seamless integration of data transmission and signal processing in LTE networks enables high-speed, low-latency communication. By leveraging advanced modulation schemes, efficient resource allocation methods, and robust signal processing algorithms, LTE ensures reliable data transfer even in challenging wireless environments. This synergy is what makes LTE a powerful technology for modern wireless communication, supporting a wide range of applications from voice calls and text messaging to high-definition video streaming and mobile broadband services. Understanding these underlying mechanisms provides a deeper appreciation for how LTE works and its capabilities in delivering high-quality mobile connectivity.
Quality of Service (QoS) and Resource Allocation
In the context of LTE (Long-Term Evolution) networks, Quality of Service (QoS) and resource allocation are critical components that ensure efficient and reliable data transmission. QoS refers to the ability of a network to provide a guaranteed level of performance for specific types of traffic, such as voice, video, or data. This is particularly important in LTE networks, where diverse applications with varying requirements coexist. For instance, real-time services like video conferencing and VoLTE (Voice over LTE) demand low latency and high throughput, while non-real-time services such as file downloads can tolerate higher latency but require substantial bandwidth. Resource allocation in LTE is managed by the eNodeB (evolved Node B), which dynamically assigns resources to user equipment (UE) based on their QoS requirements. The eNodeB uses a combination of scheduling algorithms and traffic management policies to allocate bandwidth, power, and other resources efficiently. This process involves categorizing traffic into different bearers, each with its own QoS profile defined by parameters such as guaranteed bit rate, maximum bit rate, and packet delay budget. By prioritizing traffic based on these profiles, the network ensures that critical applications receive the necessary resources to maintain their performance standards. One key mechanism for achieving this is through the use of bearer services. In LTE, each UE can have multiple bearers, each associated with a specific QoS class identifier (QCI). The QCI defines the QoS characteristics for that bearer, allowing the network to differentiate between different types of traffic and allocate resources accordingly. For example, a QCI with a low latency requirement would be assigned to a bearer carrying real-time traffic, while a QCI with higher tolerance for latency would be used for non-real-time traffic. Additionally, LTE employs advanced scheduling techniques such as Proportional Fair Scheduling (PFS) and Round Robin Scheduling to optimize resource allocation. These algorithms balance fairness among users with the need to meet QoS requirements. PFS, for instance, allocates resources based on the ratio of the instantaneous channel quality to the average channel quality over a certain time window, ensuring that users with better channel conditions receive more resources while still providing a fair share to all users. Furthermore, LTE networks utilize techniques like Admission Control and Congestion Control to manage resource allocation during periods of high demand. Admission Control prevents new sessions from being established if the network does not have sufficient resources to meet their QoS requirements, thereby preventing network overload. Congestion Control mechanisms, on the other hand, adjust resource allocation dynamically in response to changes in network load, ensuring that existing sessions maintain their QoS levels even under heavy traffic conditions. In summary, the integration of QoS and resource allocation in LTE networks is essential for delivering high-quality services across diverse applications. By leveraging advanced scheduling algorithms, bearer services with defined QoS profiles, and robust traffic management policies, LTE ensures that each type of traffic receives the appropriate level of resources necessary to meet its performance requirements. This sophisticated management of network resources not only enhances user experience but also maximizes network efficiency and reliability.
Applications and Impact of LTE
The advent of Long-Term Evolution (LTE) has revolutionized the telecommunications landscape, offering a plethora of applications that have significantly impacted various sectors. This article delves into the multifaceted uses and implications of LTE, highlighting three key areas: Mobile Broadband and High-Speed Data Services, IoT, Smart Cities, and Industrial Applications, and Future Developments and Integration with 5G. Firstly, LTE's role in enhancing mobile broadband and high-speed data services has transformed the way we access and utilize internet on-the-go. Secondly, its integration into IoT, smart cities, and industrial applications has enabled efficient data exchange and automation, driving innovation in these fields. Lastly, as we look towards the future, LTE's seamless integration with 5G technology promises even greater speeds and capabilities, setting the stage for a new era of connectivity. By exploring these dimensions, we can fully appreciate the profound impact of LTE on modern society. Transitioning to the first of these critical areas, we examine how LTE has revolutionized mobile broadband and high-speed data services, providing unparalleled connectivity and performance.
Mobile Broadband and High-Speed Data Services
Mobile broadband and high-speed data services have revolutionized the way we communicate, access information, and conduct daily activities. At the heart of this transformation is Long-Term Evolution (LTE), a wireless communication standard that has significantly enhanced mobile network capabilities. LTE's impact is multifaceted, influencing various aspects of modern life. **Applications of Mobile Broadband and High-Speed Data Services:** 1. **Enhanced Mobile Internet:** LTE provides fast and reliable internet access, enabling seamless browsing, streaming, and downloading. This has transformed the mobile internet experience, making it comparable to fixed-line broadband services. 2. **IoT and Smart Cities:** High-speed data services support the proliferation of Internet of Things (IoT) devices. Cities can now deploy smart infrastructure, such as intelligent traffic management systems and public safety networks, which rely on robust and efficient data connectivity. 3. **Remote Work and Education:** The pandemic has accelerated the adoption of remote work and online education. LTE's high-speed data capabilities ensure that professionals and students can stay connected and productive from anywhere. 4. **Healthcare:** Telemedicine has become increasingly viable with LTE, allowing for real-time consultations and remote monitoring of patients. This is particularly beneficial in rural or underserved areas where access to healthcare facilities is limited. 5. **Entertainment:** Streaming services like Netflix, Hulu, and YouTube rely on high-speed data to deliver content without buffering or lag. LTE ensures that users can enjoy their favorite shows and movies on the go. **Impact of LTE on Society:** 1. **Economic Growth:** By providing fast and reliable internet access, LTE has fostered economic growth by enabling businesses to operate more efficiently. It has also opened up new opportunities for entrepreneurship and innovation. 2. **Social Connectivity:** LTE has bridged geographical gaps by facilitating communication through social media, video calls, and messaging apps. This has strengthened social bonds and improved overall quality of life. 3. **Public Safety:** Emergency services benefit from LTE's low latency and high-speed data, enabling quicker response times and more effective crisis management. 4. **Environmental Benefits:** By supporting remote work and reducing the need for physical commuting, LTE contributes to lower carbon emissions and a more sustainable future. 5. **Digital Inclusion:** LTE networks have expanded internet access to more people worldwide, helping to bridge the digital divide and promote digital inclusion. In conclusion, mobile broadband and high-speed data services powered by LTE have had a profound impact on various sectors of society. From enhancing mobile internet experiences to supporting critical applications in healthcare, education, and public safety, LTE has become an indispensable part of modern life. As technology continues to evolve, the role of LTE in shaping our future will only continue to grow in importance.
IoT, Smart Cities, and Industrial Applications
The integration of IoT (Internet of Things), Smart Cities, and Industrial Applications is revolutionizing the way we live, work, and interact with our environment. At the heart of this transformation is the robust and reliable connectivity provided by LTE (Long-Term Evolution) technology. In Smart Cities, LTE enables the seamless communication between various urban infrastructure components such as traffic management systems, public safety networks, and energy grids. For instance, smart traffic lights can optimize traffic flow in real-time using data from sensors and cameras connected via LTE networks, reducing congestion and improving air quality. Similarly, public safety services can leverage LTE for high-speed data transmission during emergency responses, ensuring quicker and more effective interventions. In industrial settings, LTE plays a crucial role in enabling Industry 4.0 initiatives. It supports the deployment of IoT devices across manufacturing plants, allowing for real-time monitoring and control of production processes. Predictive maintenance, for example, can be facilitated through IoT sensors connected via LTE that detect anomalies in machinery before they lead to failures, thereby reducing downtime and increasing overall efficiency. Additionally, LTE's low latency and high throughput make it ideal for applications requiring precise control and data-intensive operations such as remote robotics and autonomous vehicles. The impact of LTE on these applications is multifaceted. It not only enhances operational efficiency but also drives innovation by enabling new use cases that were previously unfeasible with older technologies. For instance, in healthcare, LTE-connected medical devices can transmit patient data in real-time to healthcare providers, enabling remote monitoring and timely interventions. In agriculture, IoT sensors connected via LTE can provide farmers with detailed insights into soil moisture levels, temperature, and crop health, leading to more sustainable and productive farming practices. Moreover, the reliability and security of LTE networks are critical for mission-critical communications in industries like energy and utilities. Here, LTE supports the smart grid by enabling real-time monitoring of power distribution networks, allowing utilities to respond quickly to outages and optimize energy delivery. The security features inherent in LTE networks also protect against cyber threats, ensuring that sensitive data remains secure. In conclusion, the synergy between IoT, Smart Cities, and Industrial Applications is significantly amplified by the capabilities of LTE technology. Its high-speed data transmission, low latency, and robust security make it an indispensable tool for transforming urban infrastructure, industrial processes, and various other sectors. As we continue to evolve towards a more interconnected world, the role of LTE in facilitating these advancements will remain pivotal.
Future Developments and Integration with 5G
As we delve into the future developments and integration with 5G, it becomes evident that the evolution of wireless communication technologies is poised to revolutionize various aspects of our lives. Building on the robust foundation laid by LTE (Long-Term Evolution), 5G networks promise unprecedented speeds, lower latency, and greater connectivity. This next-generation technology is not merely an upgrade but a transformative force that will integrate seamlessly with existing LTE infrastructure to create a hybrid network ecosystem. One of the key future developments is the widespread adoption of 5G New Radio (NR) standards, which will enable faster data transfer rates and support a vast number of devices. This is particularly crucial for applications such as IoT (Internet of Things), smart cities, and autonomous vehicles, where real-time data processing is essential. The integration of 5G with LTE will ensure a smooth transition, allowing for dual-mode devices that can operate on both networks. This dual-mode capability will be instrumental in maintaining service continuity as 5G coverage expands. Another significant area of focus is the enhancement of network slicing, a feature that allows multiple independent networks to coexist on the same physical infrastructure. This will be particularly beneficial for industries requiring dedicated networks with specific performance characteristics, such as healthcare and finance. By integrating 5G network slicing with LTE's existing architecture, service providers can offer tailored solutions that meet the diverse needs of different sectors. The impact of this integration will be profound. For instance, in healthcare, 5G-enabled telemedicine services can leverage high-definition video streaming and real-time data transmission to enhance remote patient care. In the realm of smart cities, 5G's low latency and high capacity will support the efficient management of urban infrastructure, including traffic control systems and public safety networks. Additionally, the integration will facilitate the widespread adoption of edge computing, where data processing occurs closer to the user, reducing latency and improving overall network efficiency. From an economic perspective, the seamless integration of 5G with LTE is expected to drive significant growth. According to various forecasts, the global 5G market is projected to reach substantial figures by the mid-2020s, driven by increased demand for high-speed data services and IoT applications. This economic boon will also lead to job creation and innovation across multiple industries, as companies invest in developing new products and services that leverage these advanced technologies. In conclusion, the future developments and integration with 5G represent a pivotal moment in the evolution of wireless communication. By building on the strengths of LTE while introducing new capabilities, 5G networks will not only enhance existing applications but also open up new avenues for innovation and growth. As we move forward, it is clear that this integration will be a cornerstone of our increasingly connected world, driving technological advancements and societal improvements in ways we are only beginning to imagine.