What Is Kinetic Friction
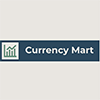
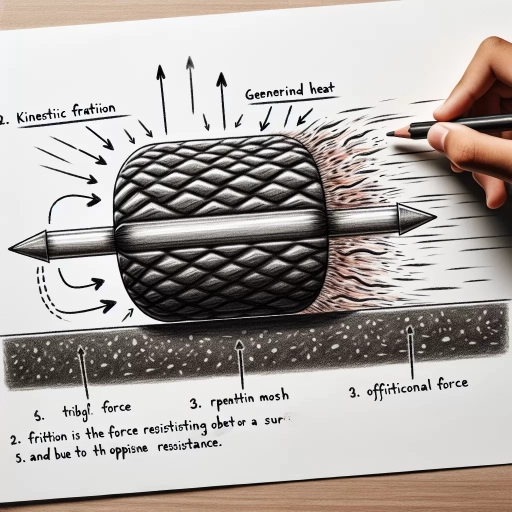
Kinetic friction, a fundamental concept in physics, plays a crucial role in our daily lives and various industrial applications. It is the force that opposes the motion of two surfaces sliding against each other. Understanding kinetic friction is essential for grasping how objects move and interact with their environment. This article delves into the intricacies of kinetic friction, starting with a detailed explanation of what it is and how it works. We will explore the causes and mechanisms behind kinetic friction, shedding light on the physical processes that drive this phenomenon. Additionally, we will examine real-world examples and applications of kinetic friction, highlighting its significance in engineering, transportation, and everyday activities. By the end of this article, you will have a comprehensive understanding of kinetic friction, beginning with the foundational concepts discussed in the section titled "Understanding Kinetic Friction."
Understanding Kinetic Friction
Understanding kinetic friction is crucial for grasping the fundamental principles of physics and its practical applications in various fields. Kinetic friction, the force opposing the motion of two surfaces in contact, is a complex phenomenon influenced by several key factors. This article delves into the definition and basic principles of kinetic friction, exploring how it arises and the underlying mechanics. Additionally, it examines the factors that influence kinetic friction, such as surface texture, normal force, and velocity, providing a comprehensive understanding of how these variables impact the frictional force. Furthermore, the article compares kinetic friction with static friction, highlighting their differences and similarities to clarify the distinct roles each plays in real-world scenarios. By understanding these aspects, readers can gain a deeper insight into the dynamics of moving objects and the forces at play. Let's begin by defining kinetic friction and its basic principles to lay the groundwork for a thorough exploration of this important concept.
Definition and Basic Principles
**Understanding Kinetic Friction** Kinetic friction, a fundamental concept in physics, is the force that opposes the motion of an object when it is already moving. To grasp this phenomenon, it is crucial to understand its definition and basic principles. **Definition and Basic Principles** Kinetic friction, also known as sliding friction, arises when two surfaces are in contact and one surface is moving relative to the other. Unlike static friction, which prevents an object from starting to move, kinetic friction acts to slow down or stop a moving object. The magnitude of kinetic friction depends on two primary factors: the normal force (the force perpendicular to the surface) and the coefficient of kinetic friction (a constant that varies depending on the materials in contact). Mathematically, kinetic friction can be expressed as \( F_k = \mu_k \times N \), where \( F_k \) is the force of kinetic friction, \( \mu_k \) is the coefficient of kinetic friction, and \( N \) is the normal force. The coefficient of kinetic friction (\( \mu_k \)) is generally lower than the coefficient of static friction (\( \mu_s \)), meaning it takes less force to keep an object moving than it does to get it moving in the first place. This coefficient is determined experimentally and can vary significantly depending on the materials involved; for example, rubber on concrete has a higher coefficient than ice on ice. Another key principle is that kinetic friction is independent of the velocity of the moving object. This means that as long as the normal force and the coefficient of kinetic friction remain constant, the force opposing motion will be the same regardless of how fast or slow the object is moving. However, in real-world scenarios, other factors such as air resistance and surface roughness can influence this relationship. Understanding these principles is essential for predicting and analyzing various phenomena in everyday life and engineering applications. For instance, in automotive engineering, knowing how kinetic friction affects braking distances is critical for designing safe vehicles. Similarly, in manufacturing processes involving moving parts, minimizing kinetic friction can improve efficiency and reduce wear on components. In summary, kinetic friction is a predictable force governed by simple yet powerful principles. By recognizing its dependence on normal force and material properties, we can better understand and manipulate this force in various contexts to achieve desired outcomes. This foundational knowledge serves as a cornerstone for more advanced studies in mechanics and engineering.
Factors Influencing Kinetic Friction
Kinetic friction, the force opposing motion between two surfaces in contact, is influenced by several key factors. **Surface Roughness** plays a significant role; smoother surfaces generally result in lower kinetic friction because there are fewer points of contact where the surfaces can "catch" and resist movement. Conversely, rougher surfaces increase the number of contact points, thereby increasing friction. **Normal Force**, or the force pressing the two surfaces together, also affects kinetic friction. Greater normal force typically leads to higher kinetic friction because it increases the pressure at each point of contact, making it harder for the surfaces to slide past each other. **Material Properties** of the surfaces are another crucial factor. Different materials have inherent coefficients of kinetic friction that determine how easily they slide against each other. For example, rubber on concrete has a higher coefficient of kinetic friction compared to steel on steel, meaning rubber on concrete will experience more resistance to motion. **Temperature** can also influence kinetic friction; in some cases, higher temperatures can soften materials, reducing friction, while in others, it may increase friction by altering the material's surface properties. Additionally, **Lubrication** significantly impacts kinetic friction. The presence of lubricants such as oils or greases can dramatically reduce kinetic friction by filling in microscopic gaps between the surfaces and reducing direct contact points. This is why lubricated surfaces often exhibit much lower kinetic friction compared to dry surfaces. **Velocity** of the moving object can also have an effect; for many materials, kinetic friction remains relatively constant with changes in velocity, but for some materials like rubber, friction can decrease with increasing speed due to the viscoelastic properties of the material. Understanding these factors is essential for predicting and controlling kinetic friction in various applications, from engineering design to everyday life. By manipulating these variables—such as using lubricants, selecting appropriate materials, or adjusting surface roughness—engineers and scientists can optimize systems for efficiency and performance. For instance, in automotive engineering, reducing kinetic friction between moving parts can improve fuel efficiency and extend the lifespan of components. Similarly, in sports equipment design, minimizing kinetic friction can enhance performance by allowing smoother motion and reducing wear on materials. By grasping the intricacies of kinetic friction and its influencing factors, we can better design and interact with our physical world.
Comparison with Static Friction
When comparing kinetic friction to static friction, several key differences emerge that are crucial for understanding the dynamics of frictional forces. **Static friction** is the force that opposes the initiation of motion between two surfaces that are in contact but not yet moving relative to each other. It acts to prevent an object from starting to move when a force is applied, and its magnitude can vary depending on the normal force and the coefficient of static friction. On the other hand, **kinetic friction** (also known as sliding or dynamic friction) comes into play once the object begins to move. This type of friction opposes the motion of an object that is already sliding over another surface. A primary distinction between the two is their coefficients: the coefficient of static friction (\(\mu_s\)) is generally higher than the coefficient of kinetic friction (\(\mu_k\)). This means that it typically takes more force to initiate movement than to maintain it. For instance, pushing a heavy box across the floor requires more effort initially to overcome static friction, but once it starts moving, less force is needed to keep it sliding due to the lower kinetic friction. Another significant difference lies in their behavior under varying conditions. Static friction can increase up to a certain limit (the maximum static frictional force), beyond which it fails and motion begins. Once this threshold is crossed, kinetic friction takes over and remains relatively constant as long as the velocity remains steady. This constancy makes kinetic friction easier to predict and model in engineering applications compared to static friction. In practical scenarios, understanding these differences is vital for designing systems where friction plays a critical role. For example, in automotive engineering, knowing how kinetic and static frictions interact helps in optimizing tire design for better traction and braking performance. Similarly, in robotics and mechanical systems, precise control over motion requires a deep understanding of both types of friction to ensure smooth operation and prevent unwanted slipping or sticking. In summary, while both static and kinetic frictions are essential components of an object's interaction with its environment, they exhibit distinct characteristics that influence how objects start moving and how they continue to move. Recognizing these differences is fundamental for grasping the broader concept of kinetic friction and its practical applications across various fields.
Causes and Mechanisms of Kinetic Friction
Kinetic friction, the force opposing motion between two surfaces in contact, is a complex phenomenon influenced by several key factors. Understanding these causes and mechanisms is crucial for optimizing performance in various engineering and scientific applications. This article delves into three primary aspects that contribute to kinetic friction: Surface Roughness and Contact Points, Normal Force and Pressure Distribution, and Material Properties and Adhesion. Surface roughness plays a significant role as it affects the number and nature of contact points between surfaces, thereby influencing the frictional force. The interaction at these contact points can significantly alter the friction coefficient. Normal force and pressure distribution also impact kinetic friction by determining the load on the contact points, which in turn affects the frictional resistance. Additionally, material properties such as hardness, elasticity, and surface energy influence adhesion between surfaces, further modifying the kinetic friction experienced. By examining these interrelated factors, we can gain a comprehensive understanding of how kinetic friction arises and how it can be manipulated. This knowledge is essential for designing efficient systems in fields ranging from automotive engineering to nanotechnology. In the following sections, we will explore each of these aspects in detail, starting with the critical role of Surface Roughness and Contact Points.
Surface Roughness and Contact Points
Surface roughness and contact points play a crucial role in understanding the causes and mechanisms of kinetic friction. When two surfaces are in contact, the actual area of contact is significantly smaller than the apparent area due to the inherent roughness of the surfaces. This roughness creates numerous micro-asperities and valleys, leading to a limited number of real contact points. These contact points are where the frictional forces are concentrated, as they resist the relative motion between the surfaces. The rougher the surfaces, the fewer and more localized these contact points become, resulting in higher frictional forces. This is because rough surfaces have more pronounced asperities that interlock and resist sliding. Conversely, smoother surfaces have fewer asperities, leading to fewer contact points and lower frictional forces. The real contact area is also influenced by the normal force applied to the surfaces; increased pressure can cause the asperities to deform, increasing the real contact area and thus affecting the frictional behavior. The adhesion theory of friction suggests that at these contact points, molecular bonds form between the surfaces, which must be broken for motion to occur. The strength of these bonds and the number of contact points directly influence the kinetic friction coefficient. Additionally, the plowing theory contributes to kinetic friction by considering the energy expended in deforming and plowing through surface asperities as one surface moves over another. In practical terms, understanding surface roughness and its impact on contact points is essential for various engineering applications. For instance, in automotive engineering, optimizing tire tread patterns to balance traction (which requires some level of surface roughness) with rolling resistance (which is minimized by smoother surfaces) is critical. Similarly, in manufacturing processes like machining and grinding, controlling surface roughness can significantly affect product quality and performance. In summary, the interplay between surface roughness and contact points is fundamental to comprehending kinetic friction. By recognizing how these factors influence real contact area and adhesion/plowing mechanisms, engineers can design more efficient systems and materials that either enhance or reduce friction depending on the application requirements. This nuanced understanding not only aids in improving existing technologies but also drives innovation in fields where friction plays a pivotal role.
Normal Force and Pressure Distribution
Normal force and pressure distribution are crucial components in understanding the mechanisms of kinetic friction. The normal force, often denoted as \(N\), is the force exerted by a surface on an object in contact with it, perpendicular to the surface. This force is a result of the interaction between the object and the surface, typically due to gravity or other external forces. Pressure distribution, on the other hand, refers to how this normal force is spread across the contact area between the object and the surface. When an object is placed on a surface, it experiences a normal force that is equal in magnitude to the weight of the object (assuming no other vertical forces are acting on it). However, this force is not uniformly distributed over the entire contact area. Instead, it varies depending on factors such as the shape of the object, its material properties, and how it is positioned on the surface. For instance, if an object has a flat bottom, the pressure will be more evenly distributed compared to an object with an irregular shape where pressure may be concentrated at certain points. The distribution of pressure significantly influences kinetic friction because frictional forces are directly proportional to the normal force. According to Coulomb's Law of Friction, kinetic friction (\(F_k\)) is given by \(F_k = \mu_k N\), where \(\mu_k\) is the coefficient of kinetic friction and \(N\) is the normal force. Therefore, any variation in pressure distribution affects not only the magnitude of the normal force but also the resulting kinetic frictional force. In practical scenarios, understanding normal force and pressure distribution can help in optimizing designs to minimize or maximize friction as needed. For example, in engineering applications like bearing design or tire tread patterns, careful consideration of how forces are distributed can lead to improved performance and efficiency. Similarly, in everyday life, recognizing how different surfaces interact with objects can explain why certain materials or surfaces provide more traction than others. In summary, normal force and pressure distribution play pivotal roles in determining kinetic friction by influencing both its magnitude and variability across different contact areas. By grasping these concepts, one can better comprehend why kinetic friction behaves differently under various conditions and how it can be manipulated for specific purposes. This understanding is essential for designing systems that require precise control over frictional forces, thereby enhancing overall performance and safety.
Material Properties and Adhesion
Material properties and adhesion play crucial roles in understanding the causes and mechanisms of kinetic friction. Kinetic friction, the force opposing motion between two surfaces in contact, is significantly influenced by the inherent characteristics of these surfaces. **Surface Roughness** is a key material property; rougher surfaces tend to have higher kinetic friction due to the increased number of contact points and the resulting mechanical interlocking. **Surface Energy**, another important property, affects adhesion by determining how strongly molecules on one surface interact with those on another. Higher surface energy typically leads to greater adhesion and, consequently, higher kinetic friction. **Adhesion** itself is a critical factor. It arises from intermolecular forces such as van der Waals forces, hydrogen bonding, and electrostatic interactions between the surfaces. When two surfaces are in close contact, these forces can create strong bonds that resist relative motion, thereby increasing kinetic friction. The **coefficient of friction**, which quantifies the ratio of frictional force to normal force, is often used to describe this relationship. Materials with high coefficients of friction (like rubber on concrete) exhibit strong adhesion and thus higher kinetic friction compared to materials with low coefficients (such as ice on ice). The **elastic and plastic properties** of materials also impact kinetic friction. Elastic materials deform reversibly under stress, which can lead to temporary increases in contact area and thus higher friction. Plastic materials, on the other hand, deform permanently and may create new surface features that alter the frictional behavior over time. Additionally, **viscoelastic properties**—the combination of viscous and elastic behaviors—can influence how materials respond to dynamic loading conditions, affecting the kinetic friction experienced during motion. **Chemical Composition** and **Surface Treatments** further influence material properties and adhesion. For instance, applying lubricants or coatings can significantly reduce surface energy and adhesion, thereby lowering kinetic friction. Conversely, certain chemical treatments can enhance surface roughness or alter the chemical nature of the surface, leading to increased adhesion and higher kinetic friction. In summary, understanding the interplay between material properties such as surface roughness, surface energy, elastic/plastic/viscoelastic behavior, chemical composition, and surface treatments is essential for comprehending the causes and mechanisms of kinetic friction. These factors collectively determine the strength of adhesion between surfaces and thus dictate the magnitude of kinetic friction encountered during motion. By manipulating these properties through various engineering techniques, it is possible to control and optimize the frictional behavior in various applications ranging from automotive engineering to biomedical devices.
Applications and Real-World Examples of Kinetic Friction
Kinetic friction, a fundamental force in physics, plays a crucial role in various aspects of our daily lives and industrial processes. This article delves into the applications and real-world examples of kinetic friction, highlighting its significance in three key areas: Transportation and Vehicle Dynamics, Industrial Processes and Machinery, and Everyday Life and Safety Considerations. In the realm of transportation, kinetic friction is essential for vehicle braking systems and tire traction, ensuring safety and control on the road. In industrial settings, it affects the efficiency and reliability of machinery, influencing production processes and equipment design. Additionally, in everyday life, kinetic friction impacts our safety and comfort, from the grip of shoes on different surfaces to the functioning of household appliances. By understanding kinetic friction, we can optimize performance, enhance safety, and improve overall efficiency across these diverse domains. This article will explore each of these areas in depth, starting with the critical role of kinetic friction in Transportation and Vehicle Dynamics.
Transportation and Vehicle Dynamics
Transportation and vehicle dynamics are intricately linked with the concept of kinetic friction, which plays a crucial role in the performance, safety, and efficiency of vehicles. Kinetic friction, the force opposing motion between two surfaces in contact, is a fundamental aspect of vehicle dynamics. In the context of transportation, kinetic friction affects various components such as tires, brakes, and gearboxes. For instance, the interaction between tires and the road surface is a prime example of kinetic friction. The coefficient of kinetic friction between the tire and the road determines the vehicle's traction, braking distance, and cornering ability. Higher kinetic friction coefficients generally provide better grip but can also lead to increased wear on tires and higher fuel consumption. In real-world applications, automotive engineers strive to optimize kinetic friction for different driving conditions. For example, tire manufacturers design tires with specific tread patterns and rubber compounds to maximize grip on various road surfaces while minimizing wear. Anti-lock braking systems (ABS) rely on the principles of kinetic friction to prevent wheels from locking up during hard braking, thereby maintaining control and reducing stopping distances. Additionally, the design of brake pads and rotors is influenced by kinetic friction to ensure effective braking performance without excessive wear. In the realm of racing, understanding and managing kinetic friction is critical for performance. Race teams often fine-tune their vehicles' suspension and tire setups to optimize the balance between grip and speed. The choice of tire compounds can significantly impact a car's handling and lap times due to variations in kinetic friction under different conditions. Moreover, kinetic friction is essential in the development of advanced driver-assistance systems (ADAS) such as traction control and electronic stability control. These systems monitor wheel speed and adjust engine power or apply brakes to individual wheels to maintain optimal traction, leveraging the principles of kinetic friction to enhance vehicle stability and safety. In summary, kinetic friction is a pivotal factor in transportation and vehicle dynamics, influencing everything from tire design and braking performance to overall vehicle safety and efficiency. By understanding and optimizing kinetic friction, engineers can create vehicles that are not only safer but also more efficient and responsive to driver input. This underscores the importance of kinetic friction in real-world applications, making it a crucial element in the design and operation of modern vehicles.
Industrial Processes and Machinery
In the realm of industrial processes and machinery, kinetic friction plays a crucial role in determining efficiency, safety, and overall performance. Kinetic friction, the force opposing motion between two surfaces in contact, is omnipresent in various industrial applications. For instance, in manufacturing plants, conveyor belts and assembly lines rely on controlled levels of kinetic friction to ensure smooth and consistent movement of products. Too little friction can lead to slipping or misalignment, while excessive friction can cause overheating and wear on machinery components. In the automotive industry, kinetic friction is critical in the functioning of brakes and clutches. The frictional force between brake pads and rotors or between clutch plates and flywheels is essential for stopping vehicles safely and smoothly. Similarly, in aerospace engineering, kinetic friction affects the performance of aircraft landing gear and braking systems, where precise control over frictional forces is vital for safe takeoffs and landings. Industrial machinery such as gears, bearings, and pistons also experience kinetic friction. Lubricants are commonly used to reduce this friction, thereby increasing the lifespan of these components and enhancing overall machine efficiency. In textile manufacturing, kinetic friction between yarns and loom components can affect fabric quality; hence, optimizing friction levels is essential for producing consistent and high-quality textiles. Moreover, in the field of robotics and automation, understanding kinetic friction is crucial for designing grippers and manipulators that can handle objects with precision. The right amount of friction ensures that robots can grasp and move objects without dropping them or causing damage. In construction, kinetic friction is relevant in the operation of cranes and hoists. The frictional forces between cables and pulleys must be carefully managed to ensure stable lifting operations. Additionally, in the oil and gas industry, drilling operations involve managing kinetic friction between drill bits and rock formations to optimize drilling speed and reduce wear on equipment. Real-world examples abound where kinetic friction has been harnessed or mitigated to achieve better outcomes. For example, the development of advanced materials like Teflon and ceramic coatings has significantly reduced kinetic friction in various applications, leading to improved performance and longevity of machinery. Similarly, advancements in lubrication technology have enabled industries to operate more efficiently by minimizing the adverse effects of kinetic friction. In summary, kinetic friction is an integral aspect of industrial processes and machinery, influencing everything from manufacturing efficiency to safety in critical applications. Understanding and managing this force is essential for optimizing performance, reducing wear on equipment, and ensuring operational reliability across diverse industries.
Everyday Life and Safety Considerations
Everyday life is replete with instances where kinetic friction plays a crucial role, often impacting safety considerations in significant ways. For instance, when driving a vehicle, kinetic friction between the tires and the road surface is essential for maintaining control and preventing skidding. Proper tire pressure and tread condition are critical to ensure optimal friction, thereby enhancing safety on the road. Similarly, in industrial settings, kinetic friction is a key factor in machinery operation; for example, the friction between moving parts can affect the efficiency and reliability of equipment. However, excessive friction can lead to overheating and wear, necessitating regular maintenance to prevent accidents. In sports, athletes rely on kinetic friction to perform effectively. For example, in football, the friction between cleats and the ground allows players to accelerate, decelerate, and change direction quickly without slipping. In contrast, inadequate friction can result in injuries from falls or slips. At home, kinetic friction is crucial for safety in various activities; for instance, the grip of shoes on flooring helps prevent slips and falls, especially in wet or slippery conditions. This underscores the importance of using non-slip mats or coatings in areas like bathrooms and kitchens. Furthermore, kinetic friction is a vital consideration in workplace safety. In manufacturing environments, workers often handle heavy objects that require secure grip to avoid accidents. The use of gloves with textured palms enhances grip through increased friction, reducing the risk of dropping items. Additionally, in construction sites, the friction between scaffolding components ensures stability and prevents collapses. In emergency situations, understanding kinetic friction can be lifesaving. For example, during a fire evacuation, the friction between feet and stairs or floors can significantly impact escape time and safety. Ensuring that escape routes have non-slip surfaces is a critical safety measure. In summary, kinetic friction is not just a theoretical concept but a practical force that influences numerous aspects of everyday life and safety. Recognizing its role and managing it appropriately can significantly enhance safety across various domains, from transportation and industry to sports and home environments. By understanding and optimizing kinetic friction, we can mitigate risks and ensure smoother, safer interactions with our surroundings.