What Is Wood Made Of
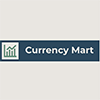
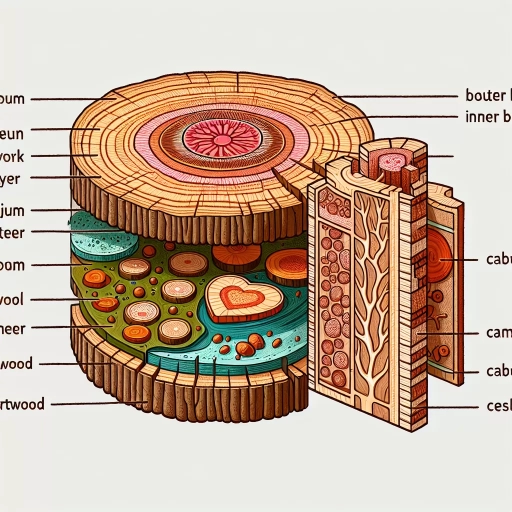
Chemical Composition of Wood
Wood, universally renowned as a vital resource, is a complex mixture of assorted chemicals. Knowledge of its chemical composition is crucial as it influences its properties, durability, use, and the methods of its preservation or modification. This article elucidates on the chemical composition of wood by primarily focusing on three fundamental constituents: Cellulose and Hemicellulose, Lignin, and other notable chemicals present. Initially, we will explore Cellulose and Hemicellulose, which are the key structuring polymers in plant biochemistry that contribute to the strength of wood. They are the plant's powerhouse, driving its growth and influencing its hardness. Following this, we'll delve into Lignin, essentially the binding agent that holds these structural components together, and creates the rigidity of wood thus playing an integral role in its functionality. Lastly, we will discuss the presence of other chemicals that exist in lesser amounts but significantly affect the wood's properties and processing techniques. Join us as we traverse this fascinating journey to understand wood's composition, beginning with the role of cellulose and hemicellulose.
Cellulose and Hemicellulose
Cellulose and hemicellulose are crucial components of wood, comprising about 65-75% of its total chemical composition. They significantly confer the distinct characteristics observed in wood, especially its strength and flexibility. Cellulose, the primary component, makes up about 40-50% of wood matter. It is a complex carbohydrate that organizes into fibrous, crystalline structures, giving wood its rigid framework and enabling it to bear significant weight. The strong intermolecular hydrogen bonds within cellulose molecules bestow it with high resistance to chemical degradation, thus contributing to wood's durability. Furthermore, cellulose's insolubility in water enhances its resistance against decay caused by microorganisms and moisture, increasing the lifespan of wooden structures. Complementing cellulose's functions is hemicellulose, accounting for about 25-35% of the wood's chemical composition. Although not as crystalline or resistant as cellulose, hemicellulose serves a vital role in interconnecting the cellulose fibers, resembling a glue that holds wood constituents together. This provides flexibility to the wood, preventing it from becoming too brittle due to the rigidity of cellulose fibers. Hemicelluloses are also more chemically diverse, as they include several types of polysaccharides, including xylan, mannan, and glucomannan. Their capability to hold a significant amount of water contributes to the wood's mechanical properties and helps regulate its moisture content. Moreover, cellulose and hemicellulose also play crucial roles during chemical interactions with other wood components, such as lignin. For example, hemicelluloses act as mediators between cellulose and lignin, forming covalent bonds that aid in maintaining the structural stability of the wood. These interplays between cellulose, hemicellulose, and lignin form the composite material that we know as wood, shaping its physical and chemical properties that make it a resourceful material in multiple industries, including construction, papermaking, and biofuel production. Thus, understanding the role of cellulose and hemicellulose in wood chemistry is not only essential in comprehending wood's unique characteristics but also crucial in optimizing its utilization in different fields. As we continue to innovate and push boundaries in sustainable practices, the potentials within the simple components of wood, cellulose, and hemicellulose, are more enlightening and promising than ever.
Lignin and Its Role
Lignin, a defining and vital constituent of wood, plays an important role in the chemical composition of wood. It is an intricate, highly branched polymer that gives the wood its structural stability and resilience. Its part is as crucial as cellulose, another fundamental element defining wood's chemistry. Lignin is a three-dimensional network of phenolic polymers that fills the spaces in the cellulose network, strengthening and hardening it. This helps protect the wood from environmental, chemical, and microbial degradation, safeguarding its overall integrity and extending its functional lifespan. Moreover, lignin contributes significantly to wood's unique features, such as its rigidity and resistance against decay and is distinctive in the plant kingdom. Accounting for about 20-35% of the composition in hardwoods and 25-35% in softwoods, its content can considerably affect the fundamental characteristics of the wood. This includes the color, hardness, and specific gravity of the wood. Furthermore, it is lignin that enables wood to resist compression without snapping. It acts as a binding agent, holding cellulose fibers together and imparting toughness to the wood. By doing so, it provides the necessary structure and stiffness, allowing the tree to stand tall and strong despite wind and weathering forces. Additionally, the role of lignin extends beyond providing structural integrity. It also plays an integral part in the water transportation system within the tree. It waterproofs the conducting vessels, allowing water to move from the roots to the topmost parts of the tree without risks of leakage. This is crucial for plants' sustenance and survival, thus reinforcing the importance of lignin in trees. Notably, lignin's chemistry is complex due to the way it forms during wood growth. It is created through a series of enzymatically controlled reactions, forming a web of linked molecules with a diverse range of structures, which contribute to its chemical complexity. Understanding lignin's composition and the intricacies of how it forms within a tree serve as a key component in many fields such as wood processing, pulping, paper manufacturing, and even biofuel production. In conclusion, lignin's role is undeniably integral in understanding the chemical composition of wood. Its vast contribution - from providing structural integrity, enabling water transportation, to affecting the wood's characteristics - illustrates its function as a fundamental component of wood.
Other Chemical Components
Apart from cellulose, hemicellulose, and lignin, which make up bulk of the composition, wood also contains other chemical components in smaller proportions. These components, although minor, still play significant roles in the characteristics and functionality of the wood. These components include extracts such as resins, tannins, and oils, proteins, pectic substances, organic acids, inorganic elements, and more. Resins, found prominently in softwoods like pine, are hydrocarbons that are soluble in organic solvents but not water. They are responsible for the pleasant aroma of certain types of wood and also contribute to the wood's waterproofing and preservation properties. Some resins are used commercially, such as rosin and turpentine, which are derived from the resin of pine trees. Tannins, common in hardwoods, offer natural protection against insects and fungi. This chemical component also plays a pivotal role in the process of leather tanning and the manufacture of ink. Similarly, different types of oils, found in specific woods like teak or cedar, serve varying purposes. Some oils contribute to the durability and water-resistance of the wood, while others are commercially valuable, like the fragrant oils from sandalwood. Proteins in the wood are relatively negligible in terms of quantity but nevertheless, play a role in the growth and metabolism of the tree. Pectic substances are involved in facilitating the movement of water in the tree, contributing to its overall health. Organic acids, such as acetic acid, are present in the wood, influencing the strength and stability of the cellulose and hemicellulose structures. Additionally, even the inorganic elements found in wood have their place and function. These trace minerals and salts, although present in minute amounts, participate in vital physiological processes of the tree, including its metabolism and growth. In light of all this, it becomes evident that the chemical composition of wood is incredibly versatile and complex, with each component contributing its unique properties to the overall structure. More than just cellulose, hemicellulose, and lignin, the additional chemical components of wood are crucial in determining the wood's characteristics, functionalities, commercial value, and its interaction with the environment. Therefore, understanding these intricate chemical compositions is crucial for those working with wood, from forestry to carpentry and industries beyond, optimizing the use and value derived from this remarkable natural resource.
Structural Components of Wood
Wood is a versatile and durable material used worldwide for a plethora of construction and design purposes. This article will delve deep into the intrinsic structural components of wood that give it this remarkable adaptability and resilience. We will first delve into the microcosmic ‘Cell Structure and Types’ that make up wood, understanding the heterogeneity and diversity of cells that give wood its fundamental strength. The article’s second focus will be on ‘Wood Fibers and Their Functions' where we will journey inside the heart of wood to elucidate the role of fibers in granting wood its flexibility and robustness. Thereafter, we will traverse into the fascinating domain of 'Wood Grain and Texture' to shed light on how different grains and textures not only affect the aesthetic of wood but also its suitability for different uses. So, hold tight as we begin this journey right at the roots, at the very cellular level of wood, to uncover the power and potency of this natural wonder. Stay tuned as we delve into our first topic: ‘Cell Structure and Types’.
Cell Structure and Types
Perplexing yet captivating, the structure and types of cells that constitute wood are an intricate tapestry of nature's ingenuity. Wood, predominantly from trees and shrubs, is comprised of multifarious cell types, each bearing distinct characteristics that bestow upon the wood its unique properties. Cells within the wood, morphologically distinct yet harmonious, form a heterogeneous ensemble, coordinating to furnish the wood with robust strength, resilience, and versatility that is exploited globally for various purposes. At the core of a tree's cell structure lie three main types of cells: parenchyma, fibers, and vessel elements or tracheids. The majority of these cells, known as tracheary elements, serve the essential purpose of conduction, allowing water and nutrients to traverse through the plant body. Parenchyma cells, with their thin walls and primordial design, act as the tree's nutrient warehouses, storing carbohydrates, and other nutrients. Intriguingly, these cells also assist in healing wounds to the tree, attributing an element of curative ability to the tree. Fibers, the skeletal warriors, confer structural tenacity to wood. These elongated cells, characterized by their thickened secondary walls and slender form, provide the wood with its rigidity and density - features that contribute significantly to its appeal as a construction material. Thirdly, vessel elements and tracheids play a pivotal role in water conduction. Vessel elements, forming longitudinal pipes, are characteristic to angiosperms, whereas tracheids, less efficient yet more supportive with their tapering ends, are predominant in gymnosperms. A tree's growth rings and their conspicuity are attributable to alternating periods of fast growth, marked by larger vessel elements or tracheids, and slower growth, underscored by their smaller counterparts. Further diverse cell types include ray cells that aid in lateral conduction and add to the visual appeal of wood, and axillary cells that contribute to the radial conduction of water and nutrients. This seamless collaboration between different cell types imbues wood with resilience, strength, and remarkable utility. The matrices of these cells, complex in their design yet simplistic in their function, construct the potent scaffold of wood. Furthermore, the biochemical composition of these cells adds to the chemical thoroughfare of wood, making it a sustainable and eco-friendly resource. This orchestration of cell structures within the wood determines not only the usage of wood but also its aesthetic appeal, durability, and resilience. Understanding this intricacy of cell architecture enables us to appreciate the value of wood beyond its apparent simplicity and motivates innovation in its utilization. The structure and types of cells in wood, thus, illuminate the essence of the very fabric of wood, beautifully entwining the complex science beneath with the tangible utility above.
Wood Fibers and Their Functions
Wood fibers, a versatile and essential byproduct of the forestry industry, play a key role in the wood structure. These elongated cells, abundant in hardwood and softwood species, grant wood its inherent strength and resilience. Effectively, wood fibers function as the backbone of the wood, providing it with its renowned durability and flexibility. The wood fibers are mainly composed of cellulose, hemicelluloses, and lignin, embedded in a matrix that gives the wood its composite structure. Cellulose, a complex carbohydrate, provides the essential strength to the wood fiber. It's essentially a biopolymer composed of glucose units linked together to form a rigid, linear structure which equips the wood with its inherent hardness. The hemicelluloses, albeit similar to cellulose in composition, are comparatively shorter and branched. They function as the link between cellulose and lignin, enabling the three components to bind together tightly and create the wood's firm structure. Lignin, an intricate organic polymer, fills the spaces in the cell wall between cellulose, hemicelluloses, and pectin components. While it is more resistant to degradation and offers rigidity to the wood fibers, it also bestows the wood with its remarkable resistance to rot and pests. Moreover, its nuanced interaction with cellulose and hemicelluloses impacts the wood's mechanical properties, yielding a matrix that can withstand considerable stress and strain before breaking or deforming. The arrangement of wood fibers in a tree plays a key role in determining the wood's overall toughness. In softwoods, the fibers are often arranged uniformly, creating a desirable homogenous grain that allows for predictable strength properties. Hardwoods typically contain a more complex cell structure, including variations in fiber density and arrangement, resulting in distinctive grain patterns that can be structurally advantageous or aesthetically pleasing. The moisture content of the wood also influences the behavior of these fibers. As wood dries, shrinkage occurs primarily in the wood fiber cells. This can lead to changes in the dimensional stability as well as the mechanical properties of the wood. Therefore, understanding wood's fiber orientation and moisture content is crucial when working with wood, whether in construction, woodworking, or pulp and paper industries. One must not disregard the impact of wood fibers on the environment. Since they are derived from a renewable source and decompose naturally over time, they considerably help in reducing the carbon footprint. Therefore, the exploration of applications of wood fibers, such as in textile development, packaging, and even innovative food applications, signifies a step towards more sustainable practices. In sum, wood fibers, through their intricate structure and versatile composition, lend wood its unique combination of strength, toughness, and aesthetic appeal. Understanding the nature of these fibers allows for their thoughtful manipulation and use in various industries, promoting both technological advancements and environmental sustainability.
Wood Grain and Texture
Wood grain and texture are vital elements in understanding the structural composition of wood. They give a glimpse into the inherent beauty and substance of this natural resource, providing invaluable insights to carpenters, manufacturers, and hobbyists. These two aspects, grain and texture, directly impact the aesthetic appeal and the functionality of wood, influencing everything from its durability and strength to its tactile quality and how it reacts to different treatments. The grain of the wood can be thought of as the alignment of its cellular structure - a distinct pattern formed by the growth rings of the tree, which is often considered the "fingerprint" of the wood. This grain can be straight, irregular, or cross, and its specific configuration modifies how the wood will respond under various crafting processes, including cutting, bending, or finishing. Straight-grained wood, for instance, is often more manageable to work with and, consequently, highly sought after in the furniture-making industry. On the other hand, irregular or cross-grained wood tends to produce visually captivating patterns, albeit with increased challenges during woodworking due to its brittle nature. No less important is the texture of the wood, which refers to the tactile quality of the wood's surface. This attribute is determined by the size and distribution of the wood's pores or cells. For example, hardwoods from slow-growing trees tend to have a fine texture due to their closely-packed cell structure, which lends itself beneficially to intricate detailing and painting. Conversely, fast-growing trees usually have a coarse texture, resulting from their larger and more unevenly distributed cells. This coarseness yields a more rustic and robust aesthetic, appealing in contexts like outdoor furniture or heavy-duty structures. The understanding of wood grain and texture is integral to unlocking the full potential of wood in various applications. Manufacturers rely on this knowledge to optimize the handling and processing of wood, ensuring that the final product aligns with the customer's requirements, both functionally and aesthetically. Meanwhile, for the end consumers, an appreciation of wood grain and texture deepens their connection to the product, enhancing their perception of its quality, heritage and natural charm. In conclusion, the intricate marriage of wood grain and texture forms an essential component of wood's structure. Behind their captivating designs lies a delicate balance of nature's artistry and pragmatic engineering, a testament to the versatile and enduring appeal of wood. Examining these characteristics is analogous to reading the life story of the tree itself, each ring and cell a vibrant chapter telling of seasons endured and growth triumphantly achieved. Thus, wood grain and texture serve as silent yet eloquent narrators of the wonderfully complex tale of what wood is made of.
Physical Properties and Characteristics
Physical properties and characteristics offer a lens to understand the essence of matter. This article will critically explore three integral physical properties which are fundamental to comprehend the nature of a material - Density and Specific Gravity, Moisture Content and Shrinkage, and Strength and Durability. First, we will study Density and Specific Gravity, both crucial in defining the substance's response under particular conditions. The higher the density, the heavier the material, while the specific gravity can shed light on the material's ability to float or sink in water. Furthermore, we will probe into Moisture Content and Shrinkage to understand the volume changes a material can undergo based on its water content. Lastly, we will focus on the Strength and Durability, primarily to assess a material's capacity to withstand stress and strain over time. The subject of discussion in the section to follow is Density and Specific Gravity, two closely related but distinct properties that establish a material's heaviness in relation to its volume and its relative density as compared to water.
Density and Specific Gravity
Density and Specific Gravity are key factors that significantly influence the physical properties and characteristics of wood. In essence, density is defined as the mass of an object divided by its volume, expressed in kilograms per cubic meter (kg/m3). In context, wood density refers to the amount of mass found in a specific volume of wood. It is a crucial metric as it directly influences the strength, hardness, and durability of the wood. High density woods, like oak or maple, are often more robust and resistant to damage, making them ideal for applications demanding strength and resilience, such as construction and furniture-making. Conversely, low-density woods, such as balsa or pine, are lighter and easier to manipulate, ideal for tasks requiring flexibility and weight efficiency, like crafting or model building. On the same note, we have Specific Gravity (SG), which is a unitless measure comparing the density of a substance to the density of a reference substance - commonly water for solids and liquids. In wood science, Specific Gravity is particularly useful as it provides information about the wood’s structure and composition, and thus its potential performance. Wood's specific gravity typically ranges between 0.3 (less dense than water) and 1.0 (as dense as water). Woods with high SG are typically harder, stronger, and more resistant to insects and decay. Examples include teak and mahogany, which make exquisite, durable outdoor furniture, and boat parts. Conversely, woods with low SG are typically softer, lighter, and more prone to damage. As you can see, understanding density and specific gravity of woods plays a vital role in selecting the appropriate type of wood for various applications. Whether you're a skilled carpenter, an amateur woodworker, or someone interested in the science behind this versatile material, knowledge about wood density and specific gravity enhances your ability to select and use wood effectively. It pays to know your wood's density and specific gravity – they're both instrumental in determining the wood's performance and end-use suitability under varying physical and environmental conditions.
Moisture Content and Shrinkage
The visible and hidden complexity of wood goes beyond its basic cellulose and lignin composition. One of the significant aspects to consider in the physical properties and characteristics of wood is its moisture content and the effect it has on shrinkage. The moisture content of wood refers to the weight of water found in the wood, compared to the weight of the dry wood itself. This measurement exhibits considerable influence on the physical properties of wood, impacting its weight, density, thermal conductivity, and even its strength. Depending on the nature of work, the ideal moisture content can vary, however, for construction purposes, the desired moisture level falls in the range of 15-19%. Due to its hygroscopic nature, wood tends to absorb or lose moisture until it reaches equilibrium with the surrounding air’s humidity. That's why the moisture content of wood changes with environmental conditions, affecting its size and shape, a phenomenon known as shrinkage. Shrinkage occurs when wood dries below its Fiber Saturation Point (FSP), a principal property that signifies the maximum amount of water hemicelluloses could accommodate without entering the cell wall's microfibrils. Beyond the FSP, wood begins to shrink, posing challenges like warp and checking. Shrinkage is not uniform throughout the wood and tends to vary along different dimensions. For instance, radial shrinkage (along the radius of the tree) is often half that of tangential shrinkage (along the circumference), resulting in unusual distortions and stresses in wood. This differential shrinkage directly impacts the dimensional stability of the wood, a crucial factor in applications like construction, furniture making, and joinery. Understanding and managing moisture content and shrinkage, therefore, become vital for maximizing the efficiency and lifespan of wooden materials. It offers insight into the behaviour of wood in varied environments, providing crucial information to manufacturers, builders, and consumers to anticipate and counter potential issues related to wood movement. Proper drying techniques, sealing, and conditioning of wood are typical strategies employed to control moisture content and minimize shrinkage. Knowledge of these aspects can significantly enhance the wood's performance, reinforcing its position as a preferred material in numerous disciplines. In conclusion, the moisture content and shrinkage are not just inherent aspects of wood's physical properties, but they are also indicative of its interaction with the environment. It emphasises the dynamic nature of this natural material, enhancing our understanding of its strengths and limitations. It underlines the reason why one must treat wood as 'living' matter, responding to environmental stimuli even after harvesting and processing.
Strength and Durability
Strength and durability are among the crucial physical properties and characteristics of wood that contribute significantly to its usefulness in various contexts, including construction, furniture manufacture, and for artistic purposes. Wood's strength is fundamentally a result of its cellular composition - its natural composition of fibres bound together through an organic compound known as lignin. This intricate natural architecture makes wood remarkably strong for its weight, allowing it to support heavy loads without fracturing. The tensile strength of wood, or its ability to resist breaking under tension, is notably high, especially in the direction parallel to the grain. Moreover, wood demonstrates considerable compressive strength, making it resistant to crushing. Meanwhile, the durability of wood is a testament to its capacity to withstand various forms of deterioration over time, including physical wear and tear, environmental influences, and biological attacks such as decay and pests. Various factors influence the durability of wood; these include the type of tree species from which the wood derives, the specific part of the tree from where the wood has been taken, and the treatment processes that the wood has undergone. For instance, heartwood – the inner section of the tree - is generally more durable than sapwood, the tree’s outer section. This is due to the presence of extractives in the heartwood that act as natural preservatives, enhancing the wood's resistance to decay. However, it is crucial to note that while wood's inherent strength and durability are impressive, these properties can be further optimized through various treatments and procedures. Techniques such as the application of chemical preservatives, thermal modification, and acetylation can significantly increase the durability of wood. Additionally, wood's strength can be improved via the lamination process, where thin layers of wood are bonded together to create a material that's stronger than natural wood. Thus, the fundamental uniqueness of wood originates not solely from its innate beauty or its renewability as a resource but from its remarkable strength and durability. These characteristics, inherent in its cellular structure and capable of augmentation through numerous treatment processes, define wood's primary utility in human society. This unrivaled combination of strength and durability underscores why wood continues to serve as an indispensable and versatile material in numerous applications spanning across centuries.