What Are Somatic Cells
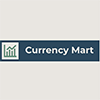
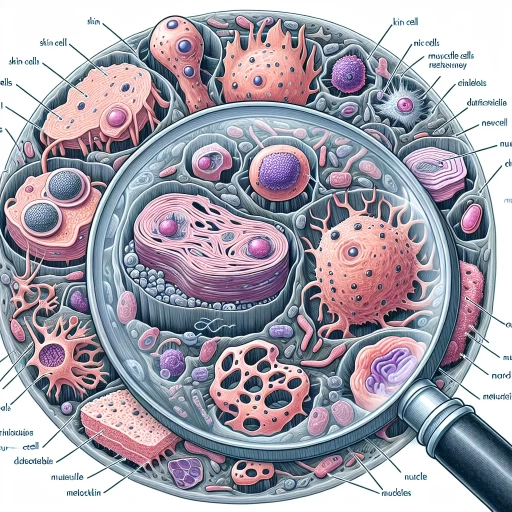
Somatic cells are the fundamental building blocks of the human body, playing a crucial role in its structure, function, and overall health. These cells, which are distinct from reproductive cells, make up the vast majority of our bodily tissues and organs. Understanding somatic cells is essential for grasping how our bodies operate and how various diseases manifest. This article delves into the world of somatic cells, exploring their definition and characteristics, the diverse types and functions they serve, and their importance in maintaining human health. By examining these aspects, we gain insight into the intricate mechanisms that govern our bodily functions. To begin, it is vital to understand the definition and characteristics of somatic cells, which will lay the groundwork for a deeper exploration of their roles and significance in our lives. --- **Definition and Characteristics of Somatic Cells**
Definition and Characteristics of Somatic Cells
Somatic cells, which comprise the majority of cells in the human body, are fundamental to understanding cellular biology and the intricate mechanisms that govern life. These cells are not involved in the reproductive process but play crucial roles in maintaining the structure and function of various tissues and organs. To delve into the world of somatic cells, it is essential to explore their origin and development, their unique cell structure and function, and how they differ from germ cells. Firstly, understanding the origin and development of somatic cells provides insights into how these cells arise from embryonic stem cells and differentiate into specialized types such as muscle, nerve, and epithelial cells. This process is pivotal in the formation and maintenance of tissues and organs. Secondly, examining the cell structure and function of somatic cells reveals their diverse roles, from contracting muscles to transmitting nerve signals, each tailored to specific physiological needs. Lastly, comparing somatic cells with germ cells highlights their distinct characteristics and purposes, underscoring the specialized nature of each cell type. By exploring these aspects, we can gain a comprehensive understanding of somatic cells and their vital contributions to human health and function. Let us begin by examining the origin and development of these essential cells, tracing their journey from embryonic beginnings to their specialized roles in the adult body.
1. Origin and Development
The origin and development of somatic cells are intricately linked to the early stages of embryonic development and the process of cell differentiation. Somatic cells, which comprise the majority of an organism's cells, originate from the fertilized egg, or zygote, through a series of complex cellular divisions and differentiations. Initially, after fertilization, the zygote undergoes several cleavage divisions without significant growth, resulting in a cluster of cells known as the blastula. This stage is followed by gastrulation, where the blastula folds inward to form three primary germ layers: ectoderm, mesoderm, and endoderm. These germ layers are the foundational tissues from which all somatic cells eventually derive. During gastrulation and subsequent developmental stages, cells within each germ layer begin to differentiate into various types of somatic cells. For instance, ectodermal cells give rise to skin, nervous tissue, and sensory organs; mesodermal cells develop into muscles, bones, and connective tissues; while endodermal cells form the lining of the digestive tract and other internal organs. This differentiation process is guided by a combination of genetic factors and environmental cues that direct cells towards specific lineage pathways. As development progresses, somatic cells continue to proliferate and mature under the influence of growth factors, hormones, and other signaling molecules. These signals ensure that cells acquire the appropriate morphology, function, and position within the organism. For example, neural stem cells in the ectoderm differentiate into neurons and glial cells through a series of tightly regulated steps involving transcriptional changes and cellular interactions. The development of somatic cells is also characterized by the establishment of tissue-specific gene expression profiles. Epigenetic modifications such as DNA methylation and histone acetylation play crucial roles in silencing or activating genes that are necessary for the specialized functions of different somatic cell types. This precise regulation ensures that each type of somatic cell performs its unique role within the organism while maintaining overall tissue homeostasis. In addition to their role in forming tissues and organs, somatic cells are integral to the maintenance and repair of these structures throughout an organism's life. They participate in processes such as wound healing, where they proliferate and differentiate to replace damaged tissue, and in immune responses, where they interact with immune cells to protect against pathogens. Understanding the origin and development of somatic cells provides valuable insights into how organisms grow, develop, and maintain their complex structures. This knowledge has significant implications for fields such as regenerative medicine, where researchers aim to harness the potential of somatic cells for tissue repair and replacement therapies. By elucidating the mechanisms underlying somatic cell differentiation and function, scientists can better address diseases related to cellular dysfunction and develop innovative treatments that restore normal cellular behavior. Thus, the study of somatic cell origin and development remains a cornerstone of modern biology and medicine.
2. Cell Structure and Function
Somatic cells, which comprise the majority of cells in an organism, exhibit a complex and highly organized structure that underpins their diverse functions. At the core of every somatic cell lies the nucleus, a membrane-bound organelle housing the genetic material essential for cellular operations. The nucleus is surrounded by the cytoplasm, a dynamic medium filled with various organelles, each specialized to perform specific tasks. Mitochondria, often referred to as the "powerhouses" of the cell, generate energy through the process of cellular respiration, ensuring that the cell has sufficient ATP to carry out its functions. The endoplasmic reticulum (ER), both rough and smooth, plays a crucial role in protein synthesis and lipid metabolism. Rough ER is studded with ribosomes, which are responsible for translating mRNA into proteins, while smooth ER is involved in detoxification and lipid synthesis. The Golgi apparatus, another key organelle, modifies, sorts, and packages proteins and lipids synthesized by the ER for transport to other parts of the cell or for secretion outside the cell. Lysosomes contain digestive enzymes that break down and recycle cellular waste and foreign substances. Peroxisomes are involved in the breakdown of fatty acids and amino acids, while chloroplasts in plant cells are responsible for photosynthesis. The cytoskeleton, comprising microtubules, microfilaments, and intermediate filaments, provides structural support, maintains cell shape, and facilitates cell division and movement. Cell membranes, composed of phospholipid bilayers with embedded proteins, regulate the movement of substances in and out of the cell through selective permeability. This selective barrier is crucial for maintaining cellular homeostasis and facilitating communication between cells. The plasma membrane also contains receptors that bind to signaling molecules, initiating various cellular responses. In addition to these structural components, somatic cells exhibit unique functional characteristics. They are capable of mitosis, allowing them to divide and produce daughter cells that are genetically identical to the parent cell. This process is essential for growth, repair, and maintenance of tissues. Somatic cells also engage in specialized functions based on their type; for example, muscle cells contract to facilitate movement, nerve cells transmit signals, and epithelial cells form protective barriers. The intricate interplay between these structural elements and functional capabilities enables somatic cells to perform a wide range of activities that are vital for the survival and proper functioning of an organism. Understanding the cell structure and function of somatic cells provides insights into how tissues and organs develop, how they respond to environmental changes, and how they contribute to overall health and disease. This knowledge is fundamental in fields such as medicine, where understanding cellular mechanisms can lead to the development of treatments for various diseases and injuries. In summary, the complex structure and specialized functions of somatic cells underscore their critical role in maintaining the integrity and functionality of an organism.
3. Comparison with Germ Cells
In the realm of cellular biology, somatic cells and germ cells represent two distinct categories that play crucial roles in the life cycle of an organism. While somatic cells are the building blocks of tissues and organs, responsible for the overall structure and function of the body, germ cells are specialized for reproduction. A comparison between these two types of cells highlights their unique characteristics and functions. **Proliferation and Differentiation**: Somatic cells, which include skin cells, muscle cells, and blood cells, undergo mitosis—a process of cell division that results in two genetically identical daughter cells. This process allows for tissue repair, growth, and maintenance. In contrast, germ cells (sperm and egg cells) undergo meiosis—a specialized form of cell division that reduces the chromosome number by half to produce haploid gametes. This ensures genetic diversity through the shuffling of chromosomes during meiosis. **Genetic Stability**: Somatic cells typically have a diploid number of chromosomes (46 in humans), which remains consistent throughout their lifespan. This stability is crucial for maintaining the integrity of bodily functions. Germ cells, however, start as diploid but become haploid through meiosis. This reduction in chromosome number is essential for sexual reproduction, allowing for the combination of genetic material from two parents to form a new diploid zygote. **Cellular Longevity**: The lifespan of somatic cells varies widely depending on their type and function. For example, red blood cells have a relatively short lifespan of about 120 days, while some neurons can last a lifetime. Germ cells, particularly those in females (oocytes), can remain dormant for many years before being activated for ovulation. Sperm cells, on the other hand, are continuously produced throughout a male's reproductive life. **Environmental Influence**: Somatic cells are more susceptible to environmental influences such as mutations caused by UV radiation or chemical exposure. These mutations can lead to diseases like cancer. Germ cells are generally more protected from such influences due to their seclusion within reproductive organs and the presence of mechanisms like DNA repair pathways that help maintain their genetic integrity. **Function and Role**: The primary function of somatic cells is to perform specific roles within tissues and organs to sustain life. For instance, muscle cells contract to facilitate movement, while epithelial cells form barriers against external pathogens. Germ cells have a singular purpose: to participate in fertilization and the transmission of genetic information from one generation to the next. In summary, the comparison between somatic and germ cells underscores their distinct biological roles and characteristics. While somatic cells are essential for the day-to-day functioning of the body through their diverse functions and ability to proliferate, germ cells are specialized for reproduction, ensuring genetic diversity and continuity across generations. Understanding these differences is fundamental to appreciating the intricate complexity of cellular biology and its implications for human health and reproduction.
Types and Functions of Somatic Cells
Somatic cells, which comprise the majority of cells in the human body, play crucial roles in maintaining overall health and function. These cells are divided into several types, each with distinct functions that contribute to the body's intricate systems. This article will delve into three primary types of somatic cells: epithelial cells, connective tissue cells, and muscle cells. Epithelial cells form the lining of various organs and glands, acting as barriers and facilitating the exchange of substances. Connective tissue cells, including those found in bone, cartilage, and fat, provide structural support and connect other tissues and organs. Muscle cells, which include skeletal, smooth, and cardiac muscles, are responsible for movement and maintaining the body's posture. Understanding these cell types is essential for grasping how the body functions and how diseases can affect different systems. Let's begin by exploring the critical role of epithelial cells in maintaining the body's integrity and facilitating essential biological processes.
1. Epithelial Cells
Epithelial cells are a fundamental type of somatic cell that form the lining of various organs, glands, and other bodily structures. These cells are tightly packed together to create a barrier that protects the underlying tissues from external damage, regulates the exchange of substances, and facilitates the secretion of hormones and other essential molecules. There are several types of epithelial cells, each with distinct functions tailored to their specific locations within the body. **Squamous Epithelial Cells**, for instance, are flat and plate-like, often found in areas where filtration and diffusion are crucial, such as the walls of blood vessels and the alveoli of the lungs. **Cuboidal Epithelial Cells** are cube-shaped and typically line glands and ducts, playing a key role in secretion and absorption processes. **Columnar Epithelial Cells** are taller and more column-like, often found in the lining of the digestive tract where they aid in absorption and secretion of digestive enzymes. In addition to these morphological types, epithelial cells also form specialized structures such as **Pseudostratified Epithelium**, where all cells are in contact with the basement membrane but appear layered due to differences in cell height, and **Stratified Epithelium**, where multiple layers of cells provide enhanced protection against mechanical stress and chemical damage. The functions of epithelial cells are multifaceted. They act as a physical barrier against pathogens and toxins, preventing them from entering the body or reaching deeper tissues. In the skin, epithelial cells form the epidermis, which shields the body from environmental hazards. In the respiratory tract, they help filter dust particles from inhaled air. Epithelial cells also play a critical role in sensory perception; for example, taste buds on the tongue are composed of specialized epithelial cells that detect different flavors. Furthermore, epithelial cells are involved in the transport of substances across their membranes. In the kidneys, they form part of the nephrons where waste products are filtered out of the blood and excreted as urine. In the intestines, they facilitate nutrient absorption by forming microvilli that increase the surface area for absorption. Another vital function of epithelial cells is their role in secretion. Glandular epithelial cells produce hormones, enzymes, and other substances that are essential for various bodily functions. For example, pancreatic islet cells secrete insulin and glucagon to regulate blood sugar levels. In summary, epithelial cells are indispensable components of the human body, providing protection, facilitating substance exchange, aiding in sensory perception, and contributing to secretion processes. Their diverse forms and functions underscore their importance as a type of somatic cell that maintains homeostasis and supports overall health.
2. Connective Tissue Cells
Connective tissue cells are a diverse group of somatic cells that play a crucial role in supporting, binding, and protecting other tissues and organs within the body. These cells are integral to the structure and function of connective tissue, which is one of the four primary types of tissue in the human body. The main types of connective tissue cells include fibroblasts, adipocytes, chondrocytes, osteocytes, and hematopoietic cells. **Fibroblasts** are the most abundant type of connective tissue cells and are responsible for producing the extracellular matrix (ECM), a complex network of proteins and polysaccharides that provides structural support to tissues. Fibroblasts secrete collagen and other matrix components, which are essential for wound healing and tissue repair. They also play a role in regulating inflammation and immune responses. **Adipocytes**, or fat cells, are specialized for storing energy in the form of lipids. There are two main types: white adipocytes, which store energy as triglycerides, and brown adipocytes, which are involved in thermogenesis, generating heat to help regulate body temperature. Adipocytes also produce hormones such as leptin and adiponectin, which influence metabolism and insulin sensitivity. **Chondrocytes** are found in cartilage and are responsible for its maintenance and repair. Cartilage serves as a cushioning material in joints and provides support to various parts of the body, such as the ears, nose, and trachea. Chondrocytes produce cartilage matrix components like collagen and proteoglycans, ensuring the integrity of cartilaginous tissues. **Osteocytes** are mature bone cells embedded within the bone matrix. They are derived from osteoblasts and play a critical role in maintaining bone health by regulating mineral ion homeostasis and responding to mechanical stress. Osteocytes communicate with other bone cells through a network of canaliculi, helping to coordinate bone remodeling processes. **Hematopoietic cells**, or blood-forming cells, are located primarily in the bone marrow and are responsible for producing all types of blood cells, including red blood cells, white blood cells, and platelets. These cells undergo differentiation into various lineages to replace old or damaged blood cells, ensuring the continuous supply of functional blood components necessary for oxygen transport, immune defense, and clotting. In summary, connective tissue cells are vital for maintaining tissue structure, facilitating repair processes, storing energy, supporting movement through cartilage and bone functions, and producing essential blood components. Their diverse roles underscore their importance in overall bodily health and function. Understanding these cells is crucial for appreciating the complex interplay between different somatic cell types that work together to sustain life.
3. Muscle Cells
Muscle cells, also known as muscle fibers, are a crucial type of somatic cell that play a pivotal role in the body's ability to move, maintain posture, and regulate various physiological processes. These cells are specialized for contraction, which allows them to generate force and move the body's skeleton, as well as facilitate the movement of substances within the body. There are three main types of muscle cells: skeletal, smooth, and cardiac. **Skeletal Muscle Cells** are the most abundant type and are responsible for voluntary movements. These cells are multinucleated, meaning they contain multiple nuclei, and are characterized by their striated appearance under a microscope due to the organized arrangement of actin and myosin filaments. Skeletal muscles are attached to bones via tendons and work in conjunction with the skeletal system to enable actions such as walking, running, and lifting. **Smooth Muscle Cells** are non-striated and lack the organized structure seen in skeletal muscles. They are found in the walls of hollow organs like the digestive tract, blood vessels, and airways. Smooth muscles are involved in involuntary movements, such as peristalsis in the digestive system and the regulation of blood pressure through vessel constriction or dilation. Unlike skeletal muscles, smooth muscles operate without conscious control. **Cardiac Muscle Cells**, or cardiomyocytes, are specialized for the heart and are essential for maintaining a consistent heartbeat throughout life. These cells are also striated but have unique features such as intercalated discs that allow them to function as a single unit, ensuring synchronized contractions necessary for efficient heart function. Cardiac muscles are involuntary, meaning they operate independently of conscious control, ensuring that the heart continues to pump blood even during sleep or periods of inactivity. The functions of muscle cells extend beyond movement; they also contribute to maintaining body temperature through shivering, supporting metabolic processes by consuming energy during contraction, and aiding in the circulation of blood and lymph. The health and integrity of muscle cells are critical for overall bodily function, and their dysfunction can lead to various diseases and conditions such as muscular dystrophy, heart failure, and hypertension. In summary, muscle cells are vital components of the somatic cell repertoire, each with distinct structures and functions tailored to specific roles within the body. Understanding these differences is essential for appreciating how these cells contribute to our overall health and ability to perform daily activities.
Importance and Role in Human Health
The importance and role of various biological processes in human health cannot be overstated, as they underpin our body's ability to maintain homeostasis, respond to threats, and recover from injuries. Three critical aspects that highlight this significance are tissue repair and regeneration, immune response and defense, and the impact on diseases and disorders. Tissue repair and regeneration are fundamental processes that enable our bodies to heal from injuries and maintain tissue integrity, ensuring that our organs and systems function optimally. The immune response and defense mechanisms protect us against pathogens and foreign substances, safeguarding our health by preventing infections and diseases. Furthermore, understanding these processes helps us comprehend the impact on diseases and disorders, allowing for the development of targeted treatments and preventive measures. By delving into these areas, we can gain a deeper appreciation for how our bodies work to keep us healthy. Let us begin by exploring the crucial role of tissue repair and regeneration in maintaining our overall health.
1. Tissue Repair and Regeneration
Tissue repair and regeneration are fundamental processes that play a crucial role in maintaining human health. These mechanisms allow the body to heal from injuries, replace damaged cells, and restore tissue function. At the heart of this process are somatic cells, which are non-reproductive cells that make up the majority of the body's tissues. When tissue damage occurs, whether due to injury, disease, or wear and tear, the body initiates a complex series of events to repair or regenerate the affected area. The initial response involves inflammation, where immune cells rush to the site to clear debris and pathogens. Following this, a cascade of cellular signals orchestrates the recruitment of stem cells and progenitor cells, which are specialized somatic cells capable of differentiating into various cell types. These cells proliferate and differentiate into the specific cell types needed to replace damaged tissue, a process guided by a precise interplay of growth factors, hormones, and other signaling molecules. In some tissues, such as the skin and gut lining, regeneration can occur rapidly and efficiently due to the presence of resident stem cells. For instance, skin stem cells in the basal layer of the epidermis continuously produce new skin cells to replace those lost through normal turnover or injury. Similarly, intestinal stem cells in the crypts of Lieberkühn ensure the constant renewal of the gut epithelium. However, not all tissues have this capacity for rapid regeneration. In organs like the heart and brain, where cell turnover is slower, repair mechanisms often involve scarring rather than complete regeneration. Here, fibroblasts and other somatic cells produce collagen and other extracellular matrix components to form scar tissue that provides structural support but lacks the full functionality of the original tissue. Despite these limitations, advances in medical science are uncovering new ways to enhance tissue repair and regeneration. For example, stem cell therapies aim to harness the regenerative potential of these cells to treat a range of conditions from heart disease to neurological disorders. Additionally, biomaterials and bioengineering techniques are being developed to create scaffolds that support cell growth and differentiation, facilitating more effective tissue repair. In summary, tissue repair and regeneration are vital processes that rely on the coordinated action of somatic cells. Understanding these mechanisms not only highlights their importance in maintaining health but also opens avenues for innovative therapeutic strategies to improve human well-being. By leveraging the regenerative capabilities of somatic cells, we can develop new treatments for a variety of diseases and injuries, ultimately enhancing our quality of life.
2. Immune Response and Defense
The immune response and defense mechanisms are pivotal components of human health, serving as the body's primary line of defense against pathogens, foreign substances, and aberrant cells. This intricate system involves a complex interplay between various cell types, tissues, and organs that work in harmony to protect the body from harm. At its core, the immune response is divided into two main branches: the innate immune system and the adaptive immune system. The **innate immune system** provides immediate defense against infections through physical barriers such as the skin and mucous membranes, which prevent pathogens from entering the body. Additionally, innate immune cells like neutrophils, macrophages, and dendritic cells recognize and eliminate pathogens through mechanisms such as phagocytosis and the release of antimicrobial peptides. This system also includes the complement system, a series of proteins that enhance the ability of antibodies and phagocytic cells to clear pathogens. In contrast, the **adaptive immune system** offers a more specific and long-lasting defense mechanism. It involves lymphocytes—T cells and B cells—that recognize and remember specific pathogens. T cells, including cytotoxic T cells and helper T cells, directly kill infected cells or assist in activating other immune responses. B cells produce antibodies that bind to pathogens, marking them for destruction by other immune cells. This adaptive response is highly specific and can remember past infections, providing immunity against future exposures to the same pathogen. The coordination between these two systems is crucial for effective immune defense. For instance, dendritic cells from the innate system can present antigens to T cells, activating the adaptive response. Similarly, antibodies produced by B cells can enhance the phagocytic activity of innate immune cells. In addition to these cellular components, various cytokines and chemokines play essential roles in orchestrating the immune response. These signaling molecules facilitate communication between different immune cells, directing them to sites of infection and modulating their activities to ensure an appropriate response. The importance of the immune response in human health cannot be overstated. It protects against a wide range of diseases caused by bacteria, viruses, fungi, and other pathogens. Moreover, it plays a critical role in surveillance against cancer cells, recognizing and eliminating aberrant cells before they can form tumors. Dysregulation or failure of the immune system can lead to immunodeficiency disorders such as HIV/AIDS or autoimmune diseases like rheumatoid arthritis and lupus, where the immune system mistakenly attacks healthy tissues. In summary, the immune response is a multifaceted defense mechanism that is essential for maintaining human health. Its ability to recognize, respond to, and remember pathogens ensures that the body remains protected against a myriad of threats, highlighting its critical role in our overall well-being.
3. Impact on Diseases and Disorders
The impact of somatic cells on diseases and disorders is multifaceted and profound, highlighting their crucial role in human health. Somatic cells, which comprise the majority of cells in the human body, are integral to various physiological processes and are often at the forefront of disease pathology. For instance, in the context of cancer, somatic mutations—genetic alterations that occur in non-reproductive cells—can lead to uncontrolled cell division and tumor formation. These mutations can arise from environmental factors, errors during DNA replication, or viral infections, underscoring the importance of maintaining genomic integrity in somatic cells to prevent oncogenesis. In autoimmune diseases such as rheumatoid arthritis and lupus, somatic cells play a pivotal role. Here, the immune system mistakenly targets and damages healthy somatic cells, leading to chronic inflammation and tissue destruction. Understanding the mechanisms by which these cells are recognized and attacked by the immune system is essential for developing targeted therapies to mitigate disease progression. Neurodegenerative disorders like Alzheimer's disease and Parkinson's disease also involve significant alterations in somatic cells. In these conditions, neuronal somatic cells undergo degeneration, leading to cognitive decline and motor dysfunction. Research into the molecular pathways involved in neuronal health and death has identified potential therapeutic targets, such as tau protein in Alzheimer's and alpha-synuclein in Parkinson's, which could help in halting or reversing disease progression. Furthermore, somatic cells are critical in the context of infectious diseases. For example, during viral infections like COVID-19, somatic cells such as epithelial cells in the respiratory tract serve as primary targets for viral entry and replication. Understanding how viruses interact with these cells is vital for developing effective antiviral therapies and vaccines. In addition to these examples, somatic cells are involved in metabolic disorders such as diabetes. Here, pancreatic beta cells—a type of somatic cell—play a crucial role in insulin production. Dysfunction or loss of these cells leads to hyperglycemia and the development of diabetes. Advances in regenerative medicine aim to replace or repair damaged beta cells, offering new hope for managing this condition. In summary, the health and function of somatic cells are central to preventing and managing a wide range of diseases and disorders. Their role in maintaining tissue integrity, responding to infections, and regulating metabolic processes underscores their importance in overall human health. As research continues to elucidate the complex interactions between somatic cells and disease processes, it opens up new avenues for therapeutic interventions aimed at preserving or restoring cellular function, thereby enhancing human well-being.