What Is 180c In Fahrenheit
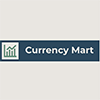
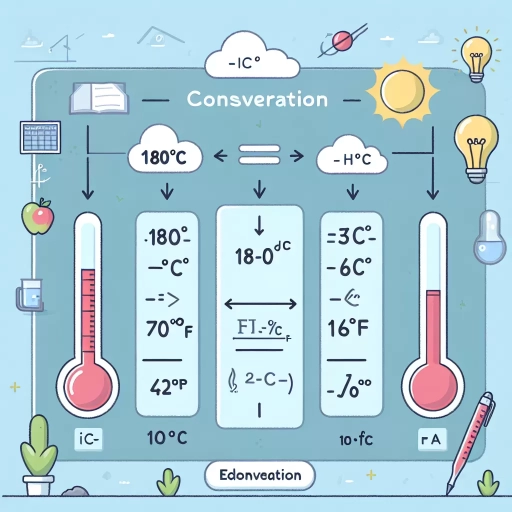
Understanding the temperature conversion from Celsius to Fahrenheit is a fundamental skill that has numerous practical applications in various fields, including science, cooking, and everyday life. One specific conversion that often arises is from 180 degrees Celsius to Fahrenheit. This article aims to provide a comprehensive overview of this conversion, starting with the basics of temperature conversion. We will delve into the mathematical process involved in converting 180°C to Fahrenheit, ensuring clarity and precision. Additionally, we will explore the practical applications and real-world examples where this conversion is crucial. By grasping these concepts, readers will gain a deeper understanding of how temperature conversions work and how they are applied in real-life scenarios. To begin, let's lay the groundwork by understanding the basics of temperature conversion.
Understanding the Basics of Temperature Conversion
Understanding the basics of temperature conversion is a fundamental skill that intersects various aspects of our daily lives, historical context, and scientific understanding. This article delves into three key areas: the introduction to Celsius and Fahrenheit scales, the importance of temperature conversion in daily life, and the historical context of temperature measurement. By grasping these concepts, readers will gain a comprehensive view of how temperature is measured and why it matters. The Celsius and Fahrenheit scales, for instance, are the two most commonly used temperature scales globally, each with its own set of applications and historical significance. In daily life, accurate temperature conversion is crucial for cooking, weather forecasting, and scientific research. Historically, the development of temperature measurement has been a gradual process involving significant contributions from scientists over the centuries. This article begins by introducing the basics of the Celsius and Fahrenheit scales, laying the groundwork for a deeper exploration of their importance and historical roots.
Introduction to Celsius and Fahrenheit Scales
Understanding the basics of temperature conversion begins with a solid grasp of the Celsius and Fahrenheit scales, two of the most widely used temperature measurement systems. The Celsius scale, named after Swedish astronomer Anders Celsius, is based on the freezing and boiling points of water. Water freezes at 0 degrees Celsius (°C) and boils at 100 °C under standard atmospheric pressure. This scale is widely used in scientific and everyday applications globally due to its simplicity and logical structure. In contrast, the Fahrenheit scale was developed by German physicist Gabriel Fahrenheit. On this scale, water freezes at 32 degrees Fahrenheit (°F) and boils at 212 °F under standard conditions. Although it is less commonly used in scientific contexts today, the Fahrenheit scale remains prevalent in everyday life in some countries, particularly the United States. To convert between these two scales, you can use specific formulas. To convert Celsius to Fahrenheit, you use the formula: \( \text{°F} = (\text{°C} \times \frac{9}{5}) + 32 \). Conversely, to convert Fahrenheit to Celsius, the formula is: \( \text{°C} = (\text{°F} - 32) \times \frac{5}{9} \). These formulas are essential for understanding how temperatures are translated from one scale to another. For instance, if you want to know what 180 °C is in Fahrenheit, you would apply the conversion formula: \( 180 \times \frac{9}{5} + 32 = 356 \). Therefore, 180 °C is equivalent to 356 °F. This conversion is crucial in various fields such as cooking, engineering, and meteorology where precise temperature measurements are vital. In summary, mastering the Celsius and Fahrenheit scales is fundamental for any temperature conversion task. Knowing how these scales are structured and how to convert between them ensures accuracy and efficiency in a wide range of applications. Whether you are a scientist, a chef, or simply someone interested in understanding weather forecasts, being able to convert between Celsius and Fahrenheit is an indispensable skill.
Importance of Temperature Conversion in Daily Life
Temperature conversion is a crucial aspect of daily life, impacting various facets of our routines and industries. Understanding how to convert between different temperature scales, such as Celsius to Fahrenheit or vice versa, is essential for accurate communication and application in diverse contexts. In cooking, for instance, knowing that 180°C is equivalent to 356°F ensures that dishes are prepared at the correct temperature, which is vital for food safety and quality. This precision prevents undercooking or overcooking, which can lead to health risks or unpalatable meals. In scientific research and engineering, temperature conversion is fundamental for data accuracy and consistency. Scientists often need to compare data collected in different parts of the world where different temperature scales are used. For example, in meteorology, converting weather data from Celsius to Fahrenheit helps in predicting weather patterns and issuing accurate forecasts. Similarly, in medical settings, precise temperature readings are critical for diagnosing and treating patients; a slight miscalculation could have serious consequences. In industrial processes, temperature control is paramount. For instance, in manufacturing, materials like metals and plastics have specific melting points that must be adhered to for quality production. Knowing the exact temperature in both Celsius and Fahrenheit ensures that these materials are processed correctly, avoiding defects or damage. Additionally, in automotive and aerospace industries, temperature conversions are vital for engine performance and safety checks. Even in everyday activities such as travel and outdoor activities, understanding temperature conversions can enhance safety and comfort. Travelers need to know how to interpret weather forecasts in different regions to pack appropriately and stay safe. For outdoor enthusiasts like hikers or skiers, knowing the temperature in both scales helps them prepare for extreme weather conditions. Moreover, temperature conversion plays a significant role in international trade and commerce. Products often come with instructions or specifications in different temperature scales depending on their country of origin. Being able to convert these temperatures accurately ensures compliance with safety standards and regulatory requirements. In conclusion, the ability to convert temperatures between different scales is not just a mathematical exercise but a practical necessity that permeates various aspects of our lives. It enhances safety, accuracy, and efficiency across multiple domains, making it an indispensable skill in today's interconnected world. Whether you are a chef, scientist, engineer, or simply an informed individual, mastering temperature conversion is key to navigating the complexities of modern life effectively.
Historical Context of Temperature Measurement
The historical context of temperature measurement is a rich and evolving narrative that spans centuries, reflecting human curiosity and the need to understand and quantify thermal phenomena. The earliest recorded attempts at measuring temperature date back to ancient civilizations, such as the Greeks and Romans, who used simple devices like water-filled vessels to observe changes in temperature. However, it wasn't until the 16th century that the first thermoscopes were developed, primarily by Italian scientist Santorio Santorio. These early thermoscopes were based on the principle that gases expand when heated, but they lacked a calibrated scale. The breakthrough came in the 17th century with the invention of the first mercury thermometer by Italian physicist Ferdinando II de' Medici in 1654. This innovation allowed for more accurate measurements and paved the way for further advancements. In 1701, German physicist Ole Rømer developed a temperature scale that divided the range between the freezing and boiling points of water into 60 degrees, laying some groundwork for modern temperature scales. The most significant milestones were achieved in the 18th century. In 1724, Gabriel Fahrenheit introduced the Fahrenheit scale, which became widely used in English-speaking countries. Fahrenheit's scale set the freezing point of water at 32 degrees and the boiling point at 212 degrees. Around the same time, Swedish astronomer Anders Celsius proposed the Celsius scale in 1742, which is now the standard metric system scale used globally. Celsius set the freezing point of water at 0 degrees and the boiling point at 100 degrees. These developments were crucial not only for scientific research but also for practical applications in fields such as medicine, chemistry, and engineering. The precision and reliability of these scales enabled scientists to conduct more accurate experiments and make significant discoveries. For instance, Antoine Lavoisier's work on the composition of air and water was heavily reliant on precise temperature measurements. In the 19th century, Lord Kelvin introduced the Kelvin scale in 1848, which is based on absolute zero (the theoretical temperature at which all molecular motion ceases). This scale is essential for scientific and engineering applications where very low temperatures are involved. Understanding these historical developments is crucial for appreciating the complexity and precision involved in modern temperature conversion. Knowing how different scales were developed and why they are used can help in converting temperatures accurately, such as converting 180 degrees Celsius to Fahrenheit. This conversion involves understanding that 180 degrees Celsius is equivalent to 356 degrees Fahrenheit, a calculation that relies on the historical foundations laid by pioneers in thermometry. In summary, the evolution of temperature measurement from primitive thermoscopes to sophisticated thermometers and calibrated scales has been a journey marked by significant scientific contributions. These advancements have not only enhanced our understanding of thermal phenomena but also facilitated precise conversions between different temperature scales, making it possible to communicate and apply thermal data accurately across various fields.
The Mathematical Process of Converting 180°C to Fahrenheit
Converting temperatures from Celsius to Fahrenheit is a fundamental mathematical process that requires precision and understanding of the underlying formula. This article delves into the intricacies of this conversion, providing a comprehensive guide for those seeking to master this skill. We will explore **The Formula for Celsius to Fahrenheit Conversion**, which serves as the backbone of the process, ensuring accurate results. Additionally, we will walk through a **Step-by-Step Calculation Example** to illustrate how the formula is applied in practice. Finally, we will highlight **Common Mistakes to Avoid During Conversion**, helping readers steer clear of pitfalls that can lead to incorrect results. By grasping these key elements, individuals can confidently convert temperatures between these two scales. Let's begin by examining **The Formula for Celsius to Fahrenheit Conversion**, which is essential for any successful temperature conversion.
The Formula for Celsius to Fahrenheit Conversion
The formula for converting Celsius to Fahrenheit is a straightforward mathematical process that involves a simple linear transformation. The formula is given by \( F = \frac{9}{5}C + 32 \), where \( F \) represents the temperature in Fahrenheit and \( C \) represents the temperature in Celsius. This formula is derived from the fact that the freezing point of water is 0°C and 32°F, and the boiling point of water is 100°C and 212°F. By using these two reference points, the linear relationship between Celsius and Fahrenheit can be established. To convert a specific temperature, such as 180°C to Fahrenheit, you would substitute \( C = 180 \) into the formula. First, multiply 180 by \( \frac{9}{5} \), which gives \( 324 \). Then, add 32 to this result, yielding \( 356 \) degrees Fahrenheit. This step-by-step process ensures accuracy and consistency in temperature conversions, making it a crucial tool in various scientific, engineering, and everyday applications. Understanding this conversion formula is essential for comparing temperatures across different scales, facilitating communication and collaboration among individuals from diverse backgrounds. It also highlights the importance of precision in scientific measurements, as small errors in temperature can have significant implications in fields such as chemistry, physics, and meteorology. By mastering this simple yet powerful formula, one can effortlessly navigate between Celsius and Fahrenheit, enhancing their ability to interpret and apply temperature data effectively.
Step-by-Step Calculation Example
To convert 180°C to Fahrenheit, follow these step-by-step calculations: 1. **Start with the Celsius temperature**: You have 180°C. 2. **Apply the conversion formula**: The formula to convert Celsius to Fahrenheit is \( F = \frac{9}{5}C + 32 \), where \( C \) is the temperature in Celsius and \( F \) is the temperature in Fahrenheit. 3. **Plug in the value**: Substitute 180 for \( C \) in the formula: \( F = \frac{9}{5}(180) + 32 \). 4. **Perform the multiplication**: Calculate \( \frac{9}{5} \times 180 \): \( \frac{9}{5} \times 180 = 324 \). 5. **Add 32**: Add 32 to the result from step 4: \( 324 + 32 = 356 \). 6. **Final result**: The temperature 180°C is equal to 356°F. This process ensures a precise conversion from Celsius to Fahrenheit, making it clear and straightforward for anyone to follow. By breaking down the calculation into manageable steps, you can easily convert any Celsius temperature to its equivalent in Fahrenheit using this method.
Common Mistakes to Avoid During Conversion
When converting temperatures from Celsius to Fahrenheit, several common mistakes can lead to inaccurate results. One of the most frequent errors is misapplying the conversion formula. The correct formula to convert Celsius to Fahrenheit is \( \text{Fahrenheit} = (\text{Celsius} \times \frac{9}{5}) + 32 \). However, many people forget to add the 32 at the end or incorrectly multiply by 9/5, leading to significant discrepancies. Another mistake is rounding intermediate calculations too early, which can introduce rounding errors that propagate through the calculation. For instance, when converting 180°C to Fahrenheit, it is crucial to perform the multiplication and addition steps precisely without premature rounding. Additionally, some individuals may confuse the order of operations or forget to convert the entire expression correctly. For example, they might multiply 180 by 9 and then divide by 5 separately instead of performing the multiplication first and then adding 32. This can result in a miscalculation that yields an incorrect temperature in Fahrenheit. Moreover, using incorrect units or failing to check the units of the input and output can also lead to confusion. Ensuring that you are working with the correct units (degrees Celsius for input and degrees Fahrenheit for output) is essential for a valid conversion. To avoid these mistakes, it is advisable to double-check each step of the calculation process. Start by multiplying 180 by 9/5 accurately, which gives \( 180 \times \frac{9}{5} = 324 \). Then, add 32 to this result to get the final temperature in Fahrenheit: \( 324 + 32 = 356 \). By following this step-by-step approach and ensuring each calculation is precise, you can avoid common pitfalls and arrive at the correct answer. In summary, converting temperatures from Celsius to Fahrenheit requires careful attention to detail and adherence to the correct formula. Avoiding premature rounding, ensuring correct order of operations, and double-checking calculations are key strategies for achieving accurate results. By being meticulous in these steps, you can confidently convert temperatures without falling into common traps that lead to errors.
Practical Applications and Real-World Examples
In today's world, precise temperature control has become a cornerstone of various practical applications, each contributing significantly to different fields. From the kitchen to industrial settings and scientific laboratories, the ability to manage temperature with accuracy is crucial. In cooking and baking, precise temperature control ensures that dishes are prepared to perfection, enhancing both flavor and texture. Industrial processes rely on high temperatures to manufacture materials and products, such as steel and ceramics, which are essential for modern infrastructure. Additionally, scientific research and data analysis often require precise temperature control to conduct experiments and gather reliable data. Understanding these applications not only highlights their importance but also underscores the versatility of temperature control in real-world scenarios. Let's delve into the first of these critical areas: **Cooking and Baking with Precise Temperature Control**.
Cooking and Baking with Precise Temperature Control
Cooking and baking with precise temperature control is a crucial aspect of culinary arts, as it directly impacts the quality, texture, and safety of the final product. Achieving the exact temperature ensures that ingredients react as intended, whether it's the Maillard reaction in searing meat or the precise gelatinization of starches in baked goods. For instance, baking a cake at 180°C (356°F) is not just about following a recipe; it's about creating an environment where chemical reactions occur optimally. This temperature allows for even rising, proper browning, and a tender crumb. In contrast, a few degrees off can result in a dense or overcooked cake. In practical applications, precise temperature control is essential for food safety. For example, cooking chicken to an internal temperature of 74°C (165°F) ensures that harmful bacteria like Salmonella are killed, preventing foodborne illnesses. Similarly, in baking bread, maintaining a consistent oven temperature helps in achieving the right crust texture and crumb structure. Professional bakers often use thermometers to monitor oven temperatures accurately, as variations can affect yeast activity and dough rise. Real-world examples abound in commercial kitchens where precise temperature control is a norm. Chefs use sous vide machines to cook meats to exact internal temperatures, ensuring uniform doneness throughout. This method eliminates the risk of overcooking and retains the natural flavors and juices of the meat. In pastry kitchens, precise temperature control is critical for making delicate pastries like croissants or macarons. The butter must be at the right temperature to laminate dough correctly, and the oven must be at a precise temperature to achieve the desired flaky layers. Moreover, home cooks can benefit from precise temperature control using modern kitchen tools like instant-read thermometers and smart ovens. These tools help in achieving consistent results, whether it's roasting a perfect turkey or baking a flawless soufflé. By understanding and applying precise temperature control, cooks can elevate their culinary skills, ensuring that every dish is not only delicious but also safe to eat. In summary, precise temperature control is a fundamental principle in both cooking and baking. It ensures that chemical reactions occur as intended, leading to better textures, flavors, and food safety. Whether in professional kitchens or home cooking, the ability to control temperature accurately is a key factor in producing high-quality dishes consistently.
Industrial Uses of High Temperatures
High temperatures play a crucial role in various industrial processes, driving efficiency, quality, and innovation across multiple sectors. In the steel industry, high temperatures are essential for smelting and refining metals. For instance, blast furnaces operate at temperatures around 2000°C (3632°F) to produce pig iron from iron ore, while electric arc furnaces reach temperatures up to 1800°C (3272°F) for steel production. These extreme conditions ensure the removal of impurities and the achievement of desired alloy properties. In the chemical industry, high-temperature reactions are fundamental for synthesizing a wide range of products. For example, the Haber-Bosch process, which produces ammonia (NH3) from nitrogen and hydrogen, requires temperatures around 450°C (842°F) and high pressures. This process is critical for the production of fertilizers and other chemicals. Similarly, in the petroleum industry, high-temperature cracking processes are used to break down complex hydrocarbons into lighter, more valuable fuels and petrochemicals. The aerospace and automotive industries also rely heavily on high-temperature technologies. In aerospace, materials like titanium and advanced composites are processed at high temperatures to achieve the necessary strength and durability for aircraft components. In automotive manufacturing, high-temperature treatments such as heat hardening and tempering are applied to steel alloys to enhance their mechanical properties, ensuring safety and performance in vehicle parts. Additionally, high temperatures are integral to energy production. In nuclear power plants, reactors operate at temperatures around 300°C (572°F) to generate steam that drives turbines, producing electricity. In solar thermal power plants, concentrated solar energy heats fluids to high temperatures, which then drive steam turbines or Stirling engines to produce electricity. In the field of materials science, high-temperature sintering is used to create advanced ceramics and composites with unique properties. This process involves heating powders to near-melting points (often above 1000°C or 1832°F) to form dense, strong materials used in applications ranging from dental implants to aerospace components. Lastly, waste management benefits from high-temperature processes such as incineration and plasma gasification. These methods involve heating waste to extremely high temperatures (up to 2000°C or 3632°F) to break down organic materials into ash and gases, reducing waste volume significantly and producing energy as a byproduct. These examples illustrate the pervasive and critical role that high temperatures play in modern industrial processes, enabling the production of high-quality materials, efficient energy generation, and innovative solutions across diverse sectors.
Scientific Research and Data Analysis
Scientific research and data analysis are the cornerstone of advancing knowledge and solving real-world problems. These processes involve systematic observation, measurement, and experimentation to formulate hypotheses and draw conclusions. In the context of practical applications, scientific research often begins with a clear question or problem statement. For instance, in understanding the conversion between Celsius and Fahrenheit, researchers might investigate the thermodynamic properties of materials at different temperatures. Data analysis then plays a crucial role in interpreting the collected data, identifying patterns, and making informed decisions. For example, in medical research, scientists use data analysis to understand the efficacy of new treatments. They collect data from clinical trials, analyze it using statistical methods, and draw conclusions about the safety and effectiveness of the treatment. Similarly, in environmental science, researchers analyze climate data to understand trends in global temperatures and predict future changes. This analysis informs policy decisions and helps in developing strategies to mitigate climate change. In business, data analysis is used to optimize operations and improve customer satisfaction. Companies collect data on consumer behavior, sales trends, and market dynamics. By analyzing this data, they can identify areas for improvement, predict future market conditions, and make strategic decisions. For instance, a company might use data analysis to determine the optimal pricing strategy for a new product or to identify the most effective marketing channels. Moreover, scientific research and data analysis are integral in technological advancements. In fields like artificial intelligence and machine learning, researchers rely heavily on data to train algorithms and improve their performance. This involves collecting large datasets, preprocessing the data, and using statistical models to train the algorithms. The insights gained from these analyses are then applied in real-world applications such as autonomous vehicles, personal assistants, and predictive analytics. In conclusion, scientific research and data analysis are essential tools for solving complex problems across various disciplines. By systematically collecting and analyzing data, researchers can uncover valuable insights that inform practical applications and drive innovation. Whether it is understanding temperature conversions, developing new medical treatments, optimizing business operations, or advancing technology, the combination of scientific research and data analysis is pivotal in making meaningful contributions to society.