What Is A Stable Octet
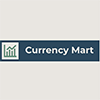
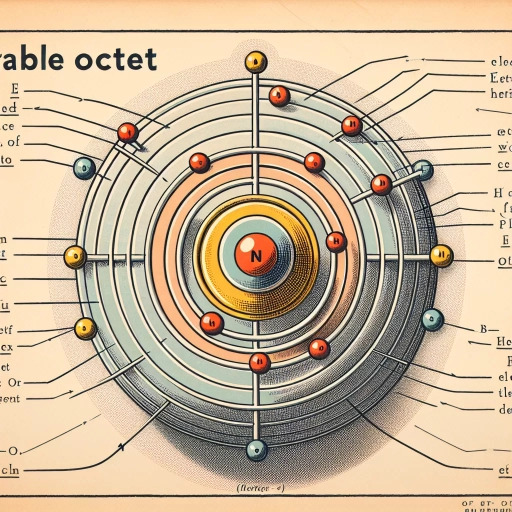
In the realm of chemistry, the concept of a stable octet is a fundamental principle that underpins the behavior of atoms and molecules. This idea revolves around the notion that atoms strive to achieve a full outer energy level, akin to the noble gas configuration, which confers stability. To delve into this concept, it is essential to first understand the underlying principles of what constitutes a stable octet. This involves grasping the theoretical framework behind it, which will be explored in the section "Understanding the Concept of a Stable Octet." Additionally, we will examine how chemical elements adhere to or deviate from the octet rule, as discussed in "Chemical Elements and the Octet Rule." Finally, we will explore the practical applications and broader implications of this concept in "Applications and Implications of a Stable Octet." By understanding these facets, we can gain a comprehensive insight into the significance and functionality of a stable octet in chemistry. Let us begin by understanding the concept of a stable octet.
Understanding the Concept of a Stable Octet
Understanding the concept of a stable octet is fundamental to grasping the principles of chemistry, particularly in the context of atomic structure and chemical bonding. This concept revolves around three key aspects: the definition and historical context, electronic configuration and stability, and the role in chemical bonding. Historically, the octet rule was first proposed by Gilbert N. Lewis and Walther Kossel in the early 20th century, laying the groundwork for modern atomic theory. The electronic configuration aspect delves into how atoms achieve a stable octet through the arrangement of electrons in their outermost shell, mirroring the noble gas configuration. This stability is crucial as it influences the chemical behavior of elements. Finally, the role in chemical bonding explains how atoms form bonds to attain this stable octet, either by sharing or transferring electrons. By exploring these facets, we gain a comprehensive understanding of why the stable octet is a cornerstone of chemical science. Let's begin by examining the definition and historical context of this pivotal concept.
Definition and Historical Context
The concept of a stable octet is deeply rooted in the historical development of atomic theory, particularly in the early 20th century. The term "octet" refers to the arrangement of eight electrons in the outermost shell of an atom, which is a key principle in understanding chemical bonding and stability. This idea was first introduced by Gilbert N. Lewis in 1916, who proposed that atoms tend to gain, lose, or share electrons to achieve a full outer shell of eight electrons, similar to the noble gas configuration. This theory was a significant departure from earlier models and laid the groundwork for modern valence theory. Historically, the concept evolved from earlier atomic models such as those proposed by J.J. Thomson and Ernest Rutherford. Thomson's "plum pudding" model suggested that atoms were composed of a positively charged sphere with embedded electrons, while Rutherford's nuclear model introduced the concept of a small, dense nucleus surrounded by electrons. However, these models did not fully explain how atoms formed bonds. Lewis's octet rule provided a clear and simple explanation for chemical reactivity and the formation of molecules. According to this rule, atoms strive to achieve an octet by forming covalent bonds with other atoms. This principle is evident in the formation of molecules like water (H₂O) and methane (CH₄), where hydrogen and carbon atoms share electrons to complete their outer shells. The historical context also involves the contributions of other scientists such as Linus Pauling, who further developed the theory of covalent bonding and introduced the concept of electronegativity. Electronegativity explains how different atoms have varying tendencies to attract shared electrons, which affects the stability and polarity of covalent bonds. In summary, the definition of a stable octet is closely tied to its historical context within the development of atomic theory. From Lewis's initial proposal to subsequent refinements by other scientists, this concept has been instrumental in understanding chemical bonding and atomic stability. It remains a fundamental principle in chemistry, guiding our understanding of molecular structure and reactivity.
Electronic Configuration and Stability
Electronic configuration and stability are intricately linked concepts in chemistry, particularly when understanding the principle of a stable octet. The electronic configuration of an atom describes how its electrons are arranged in various energy levels or shells around the nucleus. According to the Aufbau principle, electrons occupy the lowest available energy levels, while the Pauli exclusion principle states that each orbital can hold a maximum of two electrons with opposite spins. The stability of an atom is significantly influenced by its electronic configuration, especially when it achieves a full outer energy level, akin to the noble gas configuration. A stable octet refers to the arrangement where an atom has eight electrons in its outermost shell, mimicking the electronic configuration of noble gases like neon, argon, and xenon. This configuration is highly stable because it maximizes the number of paired electrons in the outer shell, minimizing the energy state of the atom. Atoms tend to gain, lose, or share electrons to achieve this stable octet configuration through chemical bonding. For instance, sodium (Na) loses one electron to form a positive ion (Na+), while chlorine (Cl) gains one electron to form a negative ion (Cl-), both achieving a stable octet. The stability provided by a full outer shell is due to the symmetrical distribution of electrons and the low reactivity associated with this configuration. Noble gases, with their complete outer shells, are chemically inert under normal conditions because they do not readily react with other elements to form compounds. This inertness underscores the stability inherent in achieving an octet. In molecules, covalent bonds are formed when atoms share pairs of electrons to achieve a stable octet. For example, in a hydrogen molecule (H₂), each hydrogen atom shares its single electron with the other, resulting in both atoms having a full outer shell with two electrons. Similarly, in water (H₂O), oxygen shares its six valence electrons with two hydrogen atoms through covalent bonds, achieving an octet. The concept of electronic configuration and stability also extends to ions and ionic compounds. When atoms form ions by gaining or losing electrons, they often do so to achieve a noble gas configuration. For instance, magnesium (Mg) loses two electrons to form Mg²⁺, which has the same electronic configuration as neon. Conversely, oxygen gains two electrons to form O²⁻, achieving the electronic configuration of neon. In summary, the electronic configuration of an atom plays a crucial role in determining its stability, with the achievement of a stable octet being a key factor. Atoms strive to attain this configuration through various chemical processes, including ionic and covalent bonding, which underscores the fundamental principle that a full outer shell is a hallmark of chemical stability. Understanding this concept is essential for grasping the broader principles of chemical reactivity and bonding.
Role in Chemical Bonding
In the context of chemical bonding, the role of achieving a stable octet is paramount. This concept, rooted in the octet rule, posits that atoms tend to gain, lose, or share electrons to attain a full outer energy level, mimicking the noble gas configuration. This stable arrangement typically involves eight electrons in the outermost shell, hence the term "octet." The driving force behind this phenomenon is the quest for stability and lower energy states. When atoms bond, they seek to fill their outermost energy level to achieve this stable octet. For instance, in ionic bonding, atoms transfer electrons to form ions with full outer shells. Sodium (Na) loses one electron to become a positively charged ion (Na+), while chlorine (Cl) gains one electron to become a negatively charged ion (Cl-). Both ions now have a stable octet, leading to the formation of sodium chloride (NaCl). In covalent bonding, atoms share pairs of electrons to achieve the desired octet. For example, in the formation of hydrogen gas (H₂), each hydrogen atom shares its single electron with another hydrogen atom, resulting in both atoms having a full outer shell with two electrons. Similarly, in methane (CH₄), carbon shares its four valence electrons with four hydrogen atoms, each contributing one electron to form four covalent bonds. This sharing ensures that both carbon and hydrogen atoms achieve a stable octet. The significance of achieving a stable octet extends beyond simple molecules. It underpins the stability of more complex compounds and even influences the reactivity of elements. Elements that are close to achieving a full outer shell are highly reactive because they readily participate in chemical reactions to complete their octet. Conversely, elements with a full outer shell are generally inert, as seen in the noble gases. Understanding the role of the stable octet in chemical bonding also helps explain various chemical properties and behaviors. For instance, it clarifies why certain compounds exhibit specific geometries and why some elements form multiple bonds. The octet rule provides a fundamental framework for predicting the types of bonds that will form between atoms and the resulting molecular structures. In summary, the pursuit of a stable octet is a fundamental principle guiding chemical bonding. By gaining, losing, or sharing electrons to achieve this configuration, atoms minimize their energy and maximize stability. This concept is central to understanding both simple and complex chemical interactions, making it a cornerstone of chemistry.
Chemical Elements and the Octet Rule
Chemical elements, the building blocks of matter, are governed by various principles that dictate their behavior and interactions. One of the most fundamental concepts in chemistry is the Octet Rule, which states that atoms tend to gain, lose, or share electrons to achieve a full outer shell with eight electrons, mimicking the noble gas configuration. This rule is crucial for understanding chemical reactivity and stability. In this article, we will delve into the intricacies of the Octet Rule by examining three key aspects: elements that typically follow this rule, exceptions to it, and the impact on chemical reactivity. We will begin by exploring **Elements That Typically Follow the Octet Rule**, highlighting how these elements achieve stability through electron configuration and bonding. This foundational understanding will set the stage for discussing exceptions and the broader implications on chemical behavior. By grasping these concepts, we can better appreciate the complex interactions that underpin the chemical world.
Elements That Typically Follow the Octet Rule
Elements that typically follow the octet rule are primarily those in the main group (s and p blocks) of the periodic table. These elements, also known as nonmetals and metalloids, tend to gain, lose, or share electrons to achieve a full outer energy level, which resembles the noble gas configuration. This rule is particularly applicable to elements in groups 1, 2, 13, 14, 15, 16, and 17. For instance, group 1 elements like sodium (Na) and potassium (K) lose one electron to form a positive ion with an octet in their outermost shell. Group 2 elements such as magnesium (Mg) and calcium (Ca) lose two electrons to achieve this stable configuration. In contrast, group 17 elements like chlorine (Cl) and bromine (Br) gain one electron to complete their outer shell, while group 16 elements like oxygen (O) and sulfur (S) gain two electrons. Group 15 elements such as nitrogen (N) and phosphorus (P) typically gain three electrons to achieve an octet. Similarly, group 14 elements like carbon (C) and silicon (Si) often form four bonds to complete their outer shell. These bonding patterns are crucial for understanding the chemical behavior and reactivity of these elements. The octet rule is also observed in molecules where atoms share pairs of electrons to achieve a stable configuration. For example, in water (H₂O), oxygen shares two pairs of electrons with hydrogen atoms, resulting in an octet for oxygen and a duet for each hydrogen atom. Similarly, in ammonia (NH₃), nitrogen shares three pairs of electrons with hydrogen atoms, achieving an octet. While the octet rule provides a useful framework for predicting chemical behavior, it is not universally applicable. Some elements, particularly transition metals and certain nonmetals, can form ions or molecules that do not follow this rule due to factors like d-orbital involvement or multiple bonding. Nonetheless, for main group elements, adhering to the octet rule is a reliable way to predict their chemical properties and reactions. This fundamental principle underpins much of inorganic and organic chemistry, making it a cornerstone in understanding the stability and reactivity of chemical compounds.
Exceptions to the Octet Rule
The Octet Rule, a fundamental principle in chemistry, states that atoms tend to gain, lose, or share electrons to achieve a full outer shell with eight electrons, mimicking the noble gas configuration. However, there are several exceptions to this rule that highlight the complexity and variability of chemical bonding. One primary exception involves molecules with an odd number of electrons, such as NO (nitric oxide) and NO2 (nitrogen dioxide). In these cases, it is impossible for all atoms to achieve an octet because there are not enough electrons to go around. Another exception is seen in molecules where an atom has more than eight electrons in its valence shell, known as expanded octets. This occurs in molecules containing phosphorus, sulfur, or chlorine, such as PCl5 (phosphorus pentachloride) and SF6 (sulfur hexafluoride), where these atoms can accommodate more than eight electrons due to the availability of d-orbitals in their valence shells. Additionally, some molecules exhibit incomplete octets, where an atom has fewer than eight electrons. Examples include BF3 (boron trifluoride) and BeCl2 (beryllium chloride), where the central atom does not have enough electrons to form additional bonds necessary for an octet. These exceptions underscore that while the Octet Rule provides a useful guideline for predicting molecular structure, it is not universally applicable and must be considered alongside other factors such as molecular geometry and the availability of d-orbitals. Understanding these exceptions is crucial for accurately predicting and explaining the behavior of various chemical compounds.
Impact on Chemical Reactivity
The impact of chemical reactivity is a fundamental aspect of understanding how elements interact and form compounds, particularly in the context of achieving a stable octet. According to the octet rule, atoms tend to gain, lose, or share electrons to achieve a full outer shell of eight electrons, mimicking the noble gas configuration. This drive for stability significantly influences chemical reactivity. For instance, highly reactive elements like sodium (Na) and chlorine (Cl) readily participate in reactions because they are far from achieving an octet. Sodium, with one electron in its outer shell, easily loses this electron to form a positive ion (Na+), while chlorine, with seven electrons in its outer shell, readily gains an electron to form a negative ion (Cl-). When these two elements react, sodium donates its single electron to chlorine, resulting in the formation of sodium chloride (NaCl), where both atoms now have a stable octet configuration. In contrast, noble gases such as neon (Ne) and argon (Ar) are chemically inert because their outer shells are already full, making them less reactive. The octet rule also explains why some elements form covalent bonds. For example, carbon (C) has four electrons in its outer shell and needs four more to achieve an octet. By sharing pairs of electrons with other atoms, such as hydrogen (H), carbon can form stable molecules like methane (CH4). Similarly, oxygen (O), which has six electrons in its outer shell, can share two pairs of electrons with hydrogen to form water (H2O). The octet rule also helps predict the reactivity of molecules. Molecules that have a full outer shell of electrons are generally more stable and less reactive. However, molecules with incomplete outer shells or those that can easily gain or lose electrons are more reactive. This principle is crucial in understanding various chemical processes, including combustion reactions, acid-base reactions, and redox reactions. Moreover, deviations from the octet rule can provide insights into the reactivity of certain elements. For example, some elements like phosphorus (P) and sulfur (S) can expand their octet by using d-orbitals, allowing them to form more than four bonds. This ability makes these elements more versatile in forming compounds and contributes to their higher reactivity compared to elements that strictly follow the octet rule. In summary, the octet rule is a cornerstone in understanding chemical reactivity. It explains why certain elements are highly reactive while others are inert, and it guides the formation of ions and molecules. By recognizing how atoms strive to achieve a stable octet, chemists can predict and explain a wide range of chemical behaviors, making this concept essential for understanding the fundamental principles of chemistry.
Applications and Implications of a Stable Octet
The concept of a stable octet is a cornerstone in chemistry, underpinning various fundamental principles and applications. This article delves into the multifaceted implications of the stable octet rule, exploring three key areas: predicting molecular structure and properties, understanding chemical reactions and bond formation, and its significance in both organic and inorganic chemistry. By grasping how atoms strive to achieve a full outer shell of eight electrons, chemists can accurately predict the shapes and reactivities of molecules. This understanding is crucial for anticipating how molecules will interact and form bonds, thereby elucidating the mechanisms behind chemical reactions. Furthermore, the stable octet principle plays a pivotal role in both organic and inorganic chemistry, guiding the synthesis of complex compounds and the interpretation of their behaviors. As we explore these dimensions, we begin by examining how the stable octet influences our ability to predict molecular structure and properties, setting the stage for a deeper dive into the intricate world of chemical interactions and compound synthesis.
Predicting Molecular Structure and Properties
Predicting molecular structure and properties is a cornerstone of modern chemistry, deeply rooted in the concept of a stable octet. The octet rule, which states that atoms tend to gain, lose, or share electrons to achieve a full outer shell of eight electrons, provides a fundamental framework for understanding molecular stability. By applying this principle, chemists can predict the likely structure of molecules and their associated properties. For instance, in covalent bonding, atoms share pairs of electrons to form bonds until each atom achieves an octet, leading to stable molecules like methane (CH₄) and water (H₂O). In ionic compounds, atoms transfer electrons to form ions with full outer shells, resulting in stable salts such as sodium chloride (NaCl). The predictive power of the octet rule extends beyond simple molecules. It helps in understanding the reactivity of elements and compounds. For example, elements in Group 17 (halogens) are highly reactive because they need only one electron to complete their octet, making them strong oxidizing agents. Similarly, elements in Group 1 (alkali metals) readily lose one electron to achieve an octet, making them highly reactive and prone to forming positive ions. Moreover, the octet rule is crucial in predicting molecular geometry. According to VSEPR (Valence Shell Electron Pair Repulsion) theory, electron pairs around a central atom arrange themselves to minimize repulsion, leading to specific geometries such as tetrahedral for methane and bent for water. These geometries influence physical properties like boiling points and solubility, as well as chemical properties such as reactivity and polarity. In addition, understanding molecular structure through the lens of the octet rule has significant implications for various fields. In pharmaceuticals, predicting the structure of drug molecules helps in designing drugs that can effectively interact with biological targets. In materials science, knowing the structure of materials at the molecular level allows for the development of new materials with tailored properties, such as conductive polymers and nanomaterials. The applications of predicting molecular structure are also evident in environmental science. For example, understanding the structure of pollutants like chlorofluorocarbons (CFCs) helped scientists recognize their potential to deplete the ozone layer, leading to international agreements to phase out these substances. Similarly, knowledge of molecular structure aids in developing more efficient catalysts for industrial processes, reducing energy consumption and environmental impact. In conclusion, predicting molecular structure and properties using the octet rule is a powerful tool that underpins many areas of chemistry and its applications. It enables chemists to understand and predict the behavior of molecules, leading to advancements in fields ranging from drug design to environmental protection. The stability conferred by a full outer shell of electrons is a fundamental principle that guides our understanding of molecular interactions and reactivity, making it an indispensable concept in modern chemistry.
Understanding Chemical Reactions and Bond Formation
Understanding chemical reactions and bond formation is crucial for grasping the fundamental principles of chemistry, particularly in the context of a stable octet. A stable octet refers to the configuration where an atom has eight electrons in its outermost shell, mimicking the noble gas configuration. This stability is achieved through various types of chemical bonds—ionic, covalent, and metallic—each involving different mechanisms of electron sharing or transfer. **Ionic Bonds:** These form when one or more electrons are transferred from one atom to another, resulting in the formation of ions with opposite charges. For instance, when sodium (Na) reacts with chlorine (Cl), sodium loses an electron to become a positively charged ion (Na⁺), while chlorine gains an electron to become a negatively charged ion (Cl⁻). The electrostatic attraction between these oppositely charged ions forms an ionic bond, stabilizing both atoms by achieving a noble gas configuration. **Covalent Bonds:** In covalent bonding, atoms share one or more pairs of electrons to achieve a stable octet. This sharing can be equal (nonpolar covalent bonds) or unequal (polar covalent bonds), depending on the difference in electronegativity between the atoms involved. For example, in the formation of methane (CH₄), carbon shares its four valence electrons with four hydrogen atoms, each contributing one electron to form four single covalent bonds. This sharing allows both carbon and hydrogen atoms to achieve a stable octet configuration. **Metallic Bonds:** Metallic bonds occur in metals and involve the delocalization of electrons among a lattice of metal ions. In this type of bonding, electrons are not localized between specific pairs of atoms but are free to move throughout the metal lattice. This "sea of electrons" model explains how metals exhibit high electrical conductivity and malleability. The delocalized electrons ensure that each metal ion has a stable octet configuration by being surrounded by a cloud of negatively charged electrons. The understanding of these bond formations has significant implications across various fields. In materials science, knowing how to manipulate bond types allows for the creation of materials with specific properties—such as conductive materials for electronics or insulating materials for thermal applications. In biochemistry, the stability provided by covalent bonds in biomolecules like proteins and DNA is essential for their structure and function. Additionally, ionic compounds are widely used in pharmaceuticals and as catalysts in industrial processes. In summary, the concept of a stable octet underpins our understanding of chemical reactions and bond formation. By recognizing how atoms achieve this stable configuration through ionic, covalent, or metallic bonds, we can better comprehend the properties and behaviors of substances around us. This knowledge has far-reaching applications in fields ranging from materials science to biochemistry, highlighting the importance of understanding chemical reactions and bond formation in achieving a stable octet.
Significance in Organic and Inorganic Chemistry
The significance of the stable octet rule in both organic and inorganic chemistry cannot be overstated. This fundamental principle, which states that atoms tend to gain, lose, or share electrons to achieve a full outer shell of eight electrons, underpins the formation and stability of molecules across various chemical disciplines. In organic chemistry, the stable octet is crucial for understanding the structure and reactivity of carbon-based compounds. Carbon's ability to form four covalent bonds allows it to create complex molecules with diverse functionalities, such as alkanes, alkenes, and alkynes. The octet rule helps predict the geometry and hybridization of carbon atoms in these molecules, which in turn influences their chemical properties and biological activities. For instance, the stability of the benzene ring, a cornerstone of aromatic chemistry, is attributed to the delocalization of electrons that ensures each carbon atom maintains a stable octet. In inorganic chemistry, the stable octet is equally vital for explaining the behavior of ionic and covalent compounds. The formation of ionic bonds between metals and nonmetals often involves the transfer of electrons to achieve a stable octet configuration for both ions. This principle is evident in the structure of common salts like sodium chloride (NaCl) and calcium carbonate (CaCO3), where the ions arrange themselves to maximize stability by fulfilling the octet rule. Additionally, covalent compounds such as water (H2O) and ammonia (NH3) owe their stability to the sharing of electrons that allows each atom to achieve a full outer shell. The implications of this rule extend to the synthesis and properties of inorganic materials, including catalysts, semiconductors, and ceramics, where the electronic configuration plays a critical role in determining their performance. Moreover, deviations from the stable octet rule can lead to interesting and useful chemical phenomena. For example, molecules with expanded octets, such as phosphorus pentachloride (PCl5), exhibit unique reactivity patterns due to the presence of additional electrons beyond the typical eight. Similarly, radicals with unpaired electrons are highly reactive intermediates that play key roles in various organic and inorganic reactions. Understanding these exceptions helps chemists design new synthetic routes and tailor materials with specific properties. The applications of the stable octet rule are far-reaching and diverse. In pharmaceuticals, it guides the design of drugs by predicting the stability and reactivity of potential therapeutic agents. In materials science, it informs the development of new materials with tailored electronic and structural properties. In environmental science, it helps in understanding the fate and transport of pollutants, many of which are organic or inorganic compounds adhering to or deviating from the octet rule. In conclusion, the stable octet rule is a foundational concept that permeates both organic and inorganic chemistry, providing a framework for understanding molecular structure, reactivity, and stability. Its significance extends beyond theoretical understanding to practical applications across various scientific disciplines, making it an indispensable tool for chemists and researchers alike.