What Are Pure Substances
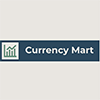
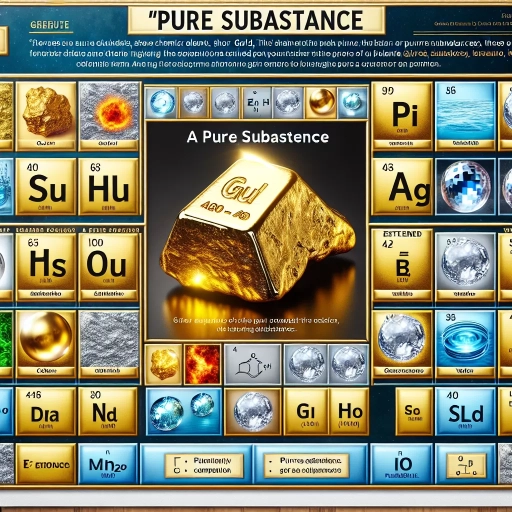
In the realm of chemistry, understanding pure substances is fundamental to grasping the building blocks of matter. Pure substances are materials that consist of only one type of compound or element, making them homogeneous in composition. This article delves into the essence of pure substances, exploring their definition and characteristics, the various types that exist, and their significant importance and applications in science and everyday life. By defining what constitutes a pure substance, we can better comprehend its unique properties and behaviors. We will also examine the different categories of pure substances, including elements and compounds, each with distinct features. Finally, we will discuss how these substances play crucial roles in various fields, from pharmaceuticals to materials science. To begin this journey, let's first establish a clear understanding of what pure substances are and what characteristics define them, setting the stage for a deeper exploration of their types and applications. --- **Definition and Characteristics of Pure Substances**
Definition and Characteristics of Pure Substances
In the realm of chemistry, understanding the nature of pure substances is fundamental to grasping the intricacies of matter. Pure substances, unlike mixtures, are composed of only one type of molecule or atom, making them homogeneous in both chemical and physical properties. This article delves into the definition and characteristics of pure substances, exploring three key aspects that define their uniqueness. First, we examine their **Chemical Composition**, highlighting how the uniformity of their molecular structure sets them apart from other forms of matter. Next, we discuss their **Physical Properties**, such as melting and boiling points, which are consistent and predictable due to their uniform composition. Finally, we differentiate pure substances from mixtures, elucidating how these distinctions are crucial in various scientific and industrial applications. By understanding these facets, we gain a comprehensive insight into the **Definition and Characteristics of Pure Substances**, enabling a deeper appreciation of their role in chemistry and beyond.
Chemical Composition
Chemical composition is a fundamental concept in understanding the nature of pure substances. It refers to the specific proportion and arrangement of elements within a substance, which defines its unique properties and characteristics. In the context of pure substances, chemical composition is crucial because it distinguishes one substance from another. For instance, water (H₂O) and carbon dioxide (CO₂) are both pure substances with distinct chemical compositions that dictate their physical and chemical properties. Water, composed of two hydrogen atoms and one oxygen atom, is a polar molecule that exhibits high surface tension and boiling point, whereas carbon dioxide, consisting of one carbon atom and two oxygen atoms, is a linear molecule that is colorless and odorless but can be harmful in high concentrations. The chemical composition of a pure substance is typically expressed through its molecular formula or structural formula. The molecular formula provides the number of atoms of each element present in a molecule, while the structural formula shows the arrangement of these atoms. For example, the molecular formula for glucose is C₆H₁₂O₆, indicating that one molecule of glucose contains six carbon atoms, twelve hydrogen atoms, and six oxygen atoms. This precise composition ensures that glucose has consistent properties such as melting point, solubility, and reactivity. Understanding the chemical composition also helps in predicting the behavior of pure substances under different conditions. For instance, knowing that sodium chloride (NaCl) is composed of sodium and chlorine ions allows us to predict its solubility in water and its ability to conduct electricity when dissolved. Similarly, the chemical composition of organic compounds like ethanol (C₂H₅OH) explains its volatility, flammability, and miscibility with water. Moreover, the concept of chemical composition is essential in various scientific and industrial applications. In chemistry labs, chemists use techniques such as mass spectrometry and nuclear magnetic resonance (NMR) spectroscopy to determine the chemical composition of unknown substances. In pharmaceuticals, the precise chemical composition of drugs ensures their efficacy and safety. In environmental science, understanding the chemical composition of pollutants helps in developing strategies for their removal and mitigation. In summary, the chemical composition of pure substances is a defining characteristic that underpins their unique properties and behaviors. It is a critical piece of information that scientists and engineers rely on to identify, analyze, and utilize substances effectively across diverse fields. By understanding and specifying the exact proportions and arrangements of elements within a substance, we can ensure consistency in its physical and chemical attributes, which is paramount for both theoretical understanding and practical applications.
Physical Properties
Physical properties are intrinsic characteristics of a substance that can be observed or measured without altering its chemical composition. These properties are fundamental in defining and distinguishing pure substances from one another. For instance, **melting and boiling points** are critical physical properties that are unique to each pure substance, serving as reliable identifiers. A pure substance will always melt and boil at the same temperature under standard conditions, whereas mixtures exhibit a range of temperatures for these phase transitions. **Density**, another key physical property, is the mass per unit volume of a substance and is constant for a pure substance at a given temperature and pressure. This consistency allows for precise identification and differentiation between substances. **Solubility** is another important physical property that describes how well a substance dissolves in a solvent. Pure substances have specific solubility characteristics, which can be used to distinguish them from other substances. For example, sodium chloride (NaCl) is highly soluble in water, while calcium carbonate (CaCO3) is relatively insoluble. **Viscosity**, or the resistance to flow, and **surface tension**, the energy at the surface of a liquid, are also physical properties that vary among pure substances. These properties are crucial in various industrial and scientific applications. The **color** and **odor** of a substance can also be considered physical properties, although they are more subjective. However, these sensory attributes can often provide initial clues about the identity of a substance. For example, iodine has a distinctive purple color and pungent odor, making it easily recognizable. In addition to these properties, **magnetic behavior** and **electrical conductivity** are significant for certain substances. Some pure substances are ferromagnetic (strongly attracted to magnets), while others may be diamagnetic (weakly repelled by magnets). Similarly, substances can be conductors, insulators, or semiconductors based on their ability to conduct electricity. Understanding these physical properties is essential for the identification, classification, and application of pure substances in various fields such as chemistry, physics, engineering, and materials science. By measuring and comparing these properties, scientists can verify the purity of a substance and distinguish it from other substances or mixtures. This precision is critical in ensuring the reliability and reproducibility of scientific experiments and industrial processes. In summary, physical properties provide a comprehensive set of characteristics that define and distinguish pure substances, making them indispensable tools in scientific inquiry and practical applications.
Distinguishing from Mixtures
Distinguishing pure substances from mixtures is a fundamental concept in chemistry, crucial for understanding the properties and behaviors of various materials. Pure substances, as defined earlier, are composed of only one type of matter and have consistent properties throughout. In contrast, mixtures contain two or more different substances that are not chemically combined and can be separated by physical means. To distinguish between these two categories, several key characteristics and methods can be employed. Firstly, the composition of a pure substance is uniform throughout, meaning that every sample of the substance will have the same properties such as melting point, boiling point, and density. For instance, water (H₂O) always boils at 100°C at standard atmospheric pressure regardless of its source. On the other hand, mixtures do not have fixed melting or boiling points; instead, they exhibit a range of temperatures over which they melt or boil. Another distinguishing feature is the ability to separate components. Pure substances cannot be separated into simpler components by physical methods like filtration, distillation, or chromatography. However, mixtures can be separated into their constituent parts using these techniques. For example, air is a mixture of gases including nitrogen, oxygen, carbon dioxide, and others which can be separated through fractional distillation. Chemical properties also serve as a basis for differentiation. Pure substances undergo chemical reactions in predictable ways and produce consistent products. Mixtures may exhibit variable reactivity depending on the proportions of their components. Additionally, pure substances have a fixed chemical formula and molecular structure which is not the case with mixtures where the composition can vary widely. In practical terms, distinguishing between pure substances and mixtures often involves laboratory tests such as spectroscopy (e.g., IR or NMR spectroscopy) which provide detailed information about molecular structure and composition. Chromatographic techniques like gas chromatography (GC) or high-performance liquid chromatography (HPLC) are also invaluable tools for identifying and quantifying the components in a mixture. In summary, the distinction between pure substances and mixtures hinges on their uniformity in composition and properties versus variability in these aspects respectively. Understanding these differences is essential for accurate identification and analysis in various scientific fields including chemistry, biology, and materials science. By recognizing whether a sample is a pure substance or a mixture through careful observation of its physical and chemical properties along with appropriate analytical techniques, scientists can better comprehend its behavior under different conditions and utilize it effectively in various applications.
Types of Pure Substances
In the realm of chemistry, pure substances are fundamental building blocks that underpin our understanding of the physical world. These substances, devoid of impurities, exhibit consistent properties and compositions, making them crucial for scientific inquiry and practical applications. This article delves into the diverse types of pure substances, exploring their definitions, characteristics, and distinctions. We will examine **Elements**, which are the simplest form of pure substances, consisting of a single type of atom. Next, we will discuss **Compounds**, which are formed when different elements combine in fixed ratios. Additionally, we will explore **Isotopes and Isomers**, variations within elements and compounds that arise from differences in atomic structure and molecular arrangement. By understanding these categories, we can better grasp the intricate world of pure substances. This journey through the types of pure substances will ultimately lead us to a deeper appreciation of their **Definition and Characteristics**, highlighting their significance in both theoretical and applied chemistry.
Elements
Elements are the fundamental building blocks of matter, comprising the simplest form of pure substances. These substances consist of only one type of atom, distinguished by their unique atomic number. The periodic table, a cornerstone of chemistry, organizes elements based on their atomic number and recurring chemical properties. Each element has a distinct set of physical and chemical characteristics that set it apart from others. For instance, hydrogen is the lightest element and is highly reactive, while carbon forms the basis of organic chemistry due to its ability to form complex molecules. The diversity of elements is vast, with over 100 naturally occurring and several synthetic ones. Metals, nonmetals, and metalloids are the primary categories into which elements are classified. Metals, such as iron and copper, are typically malleable, conductive, and often shiny. Nonmetals, including oxygen and nitrogen, are generally brittle, poor conductors, and may exist in various states at room temperature. Metalloids, like silicon and germanium, exhibit intermediate properties between metals and nonmetals. Elements play crucial roles in various fields. In biology, elements such as carbon, oxygen, hydrogen, and nitrogen form the backbone of life as they are the primary components of biomolecules like proteins, carbohydrates, fats, and nucleic acids. In technology, silicon is pivotal in the production of semiconductors for electronic devices. In medicine, radioactive elements like iodine-131 are used for diagnostic imaging and therapeutic purposes. The study of elements has led to significant advancements in science and technology. The discovery of new elements continues to expand our understanding of the periodic table and its predictive power. For example, the synthesis of superheavy elements has pushed the boundaries of nuclear physics and chemistry. Understanding the properties and behaviors of elements is essential for developing new materials with specific properties, such as superconductors or nanomaterials. In conclusion, elements are foundational pure substances that underpin all matter. Their unique properties and diverse applications make them indispensable in various scientific disciplines and technological innovations. The ongoing exploration and characterization of elements continue to enrich our knowledge of the natural world and drive progress in multiple fields. As a subset of pure substances, elements highlight the complexity and beauty inherent in the simplest forms of matter.
Compounds
**Compounds** Compounds are a fundamental type of pure substance, distinguished by their composition of two or more different elements chemically bonded together in a fixed ratio. Unlike mixtures, where the components can be separated by physical means, compounds require chemical reactions to break the bonds between their constituent elements. This characteristic stability and uniformity make compounds essential in various fields such as chemistry, biology, and materials science. The formation of a compound involves the sharing or transfer of electrons between atoms, resulting in the creation of molecules with unique properties that differ significantly from those of the individual elements. For instance, water (H₂O) is a compound composed of hydrogen and oxygen atoms; its properties, such as its boiling point and solubility, are distinct from those of hydrogen gas and oxygen gas. Similarly, sodium chloride (NaCl), commonly known as table salt, is a compound formed from sodium and chlorine atoms, exhibiting properties like high melting point and solubility in water that are not present in its elemental form. Compounds can be classified into several types based on their chemical structure and properties. Molecular compounds, such as carbon dioxide (CO₂) and ammonia (NH₃), consist of discrete molecules held together by covalent bonds. Ionic compounds, like sodium chloride (NaCl) and calcium carbonate (CaCO₃), are formed through ionic bonds between positively charged cations and negatively charged anions. Additionally, there are intermetallic compounds which involve metallic bonds between different metals. The diversity of compounds is vast, ranging from simple molecules like hydrogen peroxide (H₂O₂) to complex biological molecules such as proteins and nucleic acids. These substances play critical roles in biological processes; for example, DNA (deoxyribonucleic acid) is a compound that stores genetic information in living organisms. In industrial applications, compounds like silicon dioxide (SiO₂) are used in the production of semiconductors and glass. Understanding compounds is crucial for advancing scientific knowledge and technological innovations. Their unique properties make them indispensable in various industries including pharmaceuticals, where compounds like aspirin (acetylsalicylic acid) are synthesized for medicinal use. In environmental science, studying compounds helps in understanding pollution and developing strategies for remediation. The study of compounds also underpins our understanding of chemical reactions and the principles of stoichiometry, which are foundational concepts in chemistry. In summary, compounds represent a vital category of pure substances characterized by their chemical composition and the fixed ratios of their constituent elements. Their diverse range and unique properties make them essential components in both natural systems and human-made technologies, underscoring their importance across multiple scientific disciplines.
Isotopes and Isomers
Within the realm of pure substances, the concepts of isotopes and isomers are crucial for understanding the diversity and complexity of chemical elements and compounds. **Isotopes** are atoms of the same chemical element that have the same number of protons (atomic number) but differ in the number of neutrons in their nuclei. This variation in neutron count leads to differences in atomic mass while maintaining identical chemical properties due to the unchanged number of protons. For instance, carbon-12, carbon-13, and carbon-14 are isotopes of carbon, each with 6 protons but varying numbers of neutrons. Isotopes play significant roles in various fields such as medicine, where radioactive isotopes like carbon-14 are used for dating organic materials, and in environmental science, where stable isotopes help trace the origins of water and nutrients. **Isomers**, on the other hand, refer to molecules that have the same molecular formula but differ in their structural arrangement of atoms. This distinction can be further categorized into two main types: **structural isomers** and **stereoisomers**. Structural isomers have different bond connections between atoms, leading to distinct physical and chemical properties. For example, ethanol (CH₃CH₂OH) and dimethyl ether (CH₃OCH₃) are structural isomers with the same molecular formula (C₂H₆O) but different structures. Stereisomers, including enantiomers and diastereomers, differ in the spatial arrangement of their atoms without altering the bond connections. This spatial difference can significantly affect their biological activity; for instance, the enantiomers of thalidomide exhibit vastly different effects on the human body. Understanding isotopes and isomers is essential for chemists and researchers as it allows them to identify and characterize substances accurately. In pharmaceuticals, knowing the specific isomer of a drug can be critical for its efficacy and safety. Similarly, in environmental studies, distinguishing between isotopes helps scientists track nutrient cycles and understand ecological processes. The precise identification of these variations underpins many scientific advancements and applications, making them fundamental concepts in the study of pure substances. By recognizing how isotopes and isomers differ, scientists can better comprehend the intricate world of chemical substances and their diverse roles in various fields. This nuanced understanding not only enriches our knowledge but also drives innovation and discovery in chemistry and related disciplines.
Importance and Applications of Pure Substances
Pure substances are fundamental components in various scientific, industrial, and environmental contexts, playing a crucial role in advancing our understanding and application of chemistry. The importance of pure substances cannot be overstated, as they form the basis for scientific research and analysis, enabling precise experiments and reliable data collection. In industrial and pharmaceutical settings, pure substances are essential for the development and production of high-quality products, ensuring consistency and efficacy. Additionally, the environmental and health implications of pure substances are significant, as their purity can impact both ecological balance and human well-being. This article delves into these critical aspects, exploring the scientific research and analysis that rely on pure substances, their industrial and pharmaceutical uses, and the environmental and health implications associated with them. By understanding these applications and implications, we can better appreciate the definition and characteristics of pure substances, which are the cornerstone of chemical science and its practical applications.
Scientific Research and Analysis
Scientific research and analysis play a pivotal role in understanding the importance and applications of pure substances. At the heart of scientific inquiry lies the meticulous process of isolating, characterizing, and studying these substances. Pure substances, whether elements or compounds, are crucial because they exhibit consistent and predictable properties, which are essential for reliable scientific experimentation. Through rigorous analytical techniques such as chromatography, spectroscopy, and mass spectrometry, researchers can verify the purity and composition of substances. This precision is vital in various fields like chemistry, biology, and pharmacology, where even trace impurities can significantly alter outcomes. In chemistry, pure substances serve as standards for chemical reactions and synthesis. For instance, in organic chemistry, the synthesis of complex molecules often requires starting materials that are highly pure to ensure the desired product is formed without unwanted side reactions. Similarly, in analytical chemistry, pure substances are used as reference materials to calibrate instruments and validate analytical methods. This ensures that the data obtained from experiments are accurate and reproducible. In biological research, pure substances are indispensable for understanding cellular processes and developing therapeutic agents. For example, the study of enzymes and their substrates requires highly purified samples to elucidate their mechanisms of action accurately. Moreover, pharmaceutical research relies heavily on pure substances to develop drugs with consistent efficacy and minimal side effects. The purity of active pharmaceutical ingredients (APIs) is critical to ensure patient safety and drug effectiveness. Furthermore, the applications of pure substances extend beyond laboratory settings. In industrial processes, pure substances are used to manufacture high-quality products such as semiconductors, catalysts, and specialty chemicals. For instance, silicon wafers used in electronics must be extremely pure to function correctly in microchips. Additionally, in environmental science, pure substances help in the development of sensors and analytical tools that monitor water and air quality, enabling precise detection of pollutants. The importance of scientific research and analysis in the context of pure substances is also reflected in their role in advancing technological innovations. For example, the development of new materials like nanomaterials and advanced ceramics often involves the synthesis and characterization of pure substances with unique properties. These materials have potential applications in fields such as energy storage, aerospace engineering, and biomedical devices. In conclusion, scientific research and analysis are fundamental to understanding and harnessing the potential of pure substances. By ensuring the purity and characterization of these substances through rigorous analytical methods, scientists can unlock their full potential across various disciplines, from basic research to industrial applications. This underscores the critical importance of maintaining high standards of purity in scientific inquiry to drive innovation and progress.
Industrial and Pharmaceutical Uses
Pure substances play a pivotal role in both industrial and pharmaceutical sectors, where their precise chemical composition is crucial for ensuring consistency, efficacy, and safety. In industrial applications, pure substances are essential for the production of high-quality materials and products. For instance, in the semiconductor industry, ultra-pure silicon is required to manufacture microchips and other electronic components. The purity of silicon ensures that these components operate reliably and efficiently, which is vital for modern technology. Similarly, in the chemical industry, pure substances are used as catalysts or reactants to produce a wide range of products such as plastics, dyes, and fertilizers. The use of pure substances in these processes helps in achieving predictable outcomes and maintaining product quality. In the pharmaceutical sector, the importance of pure substances cannot be overstated. Drugs and medications must be formulated from pure active pharmaceutical ingredients (APIs) to guarantee their potency and safety for human consumption. Impurities can significantly alter the therapeutic effect of a drug or introduce harmful side effects. Therefore, pharmaceutical companies adhere to stringent purity standards during the synthesis and purification of APIs. Additionally, excipients—substances that are added to a drug to enhance its stability or bioavailability—must also be of high purity to avoid any adverse reactions. The rigorous testing and quality control measures in place for pharmaceuticals rely heavily on the availability of pure substances, ensuring that medications are both effective and safe for patients. Moreover, pure substances are critical in research and development across various fields. In scientific research, pure substances serve as standards for calibration and validation of analytical methods. This ensures that experimental results are accurate and reproducible. In biotechnology, pure enzymes and other biomolecules are used to develop diagnostic kits and therapeutic agents. The purity of these biomolecules is essential for their specific functions and interactions within biological systems. Overall, the importance of pure substances in industrial and pharmaceutical applications underscores their role in maintaining product quality, ensuring safety, and driving innovation. The stringent requirements for purity in these sectors highlight the necessity of advanced purification techniques and rigorous quality control measures to produce substances that meet the highest standards. By leveraging pure substances, industries can achieve greater precision, reliability, and efficacy in their products and processes, ultimately contributing to advancements in technology, healthcare, and other critical areas.
Environmental and Health Implications
The importance and applications of pure substances are underscored by their significant environmental and health implications. Pure substances, by definition, consist of only one type of molecule or atom, making them crucial in various scientific, industrial, and medical contexts. However, their purity also means that any adverse effects they may have on the environment or human health are more pronounced and predictable. For instance, in environmental science, pure substances like chemicals and metals can be particularly hazardous if released into ecosystems. Chlorine gas, a pure substance used in water treatment, is highly toxic to aquatic life if not properly managed. Similarly, heavy metals such as mercury and lead, when present in their pure form, can accumulate in the food chain and cause severe health issues including neurological damage and developmental problems. In the realm of public health, the purity of substances is critical for ensuring safety in medical treatments and consumer products. Pharmaceuticals, for example, must be synthesized and purified to exacting standards to ensure efficacy and minimize side effects. Impurities in drugs can lead to adverse reactions or reduced effectiveness, highlighting the importance of stringent quality control measures. Additionally, pure substances are essential in diagnostic tools and medical research; for instance, pure isotopes are used in imaging techniques like PET scans to provide precise diagnostic information without the interference of impurities. Moreover, the environmental impact of pure substances extends beyond direct toxicity to include broader ecological consequences. The production processes for many pure substances often involve energy-intensive methods that contribute to greenhouse gas emissions and climate change. For example, the extraction and refining of pure silicon for solar panels require significant amounts of energy, which can offset some of the environmental benefits associated with renewable energy technologies. In conclusion, while pure substances offer numerous benefits across various fields due to their predictable properties and high purity levels, it is imperative to consider their potential environmental and health implications. By understanding these risks and implementing stringent regulations and sustainable practices, we can harness the advantages of pure substances while mitigating their negative impacts on both human health and the environment. This balanced approach ensures that the applications of pure substances align with broader societal goals of sustainability and public well-being.