What Is 225 Celsius In Fahrenheit
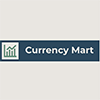
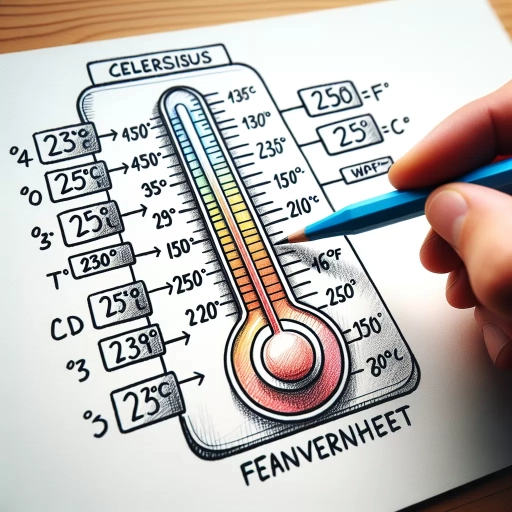
Understanding temperature conversions is crucial in various fields, from science and engineering to everyday life. One such conversion that often arises is transforming 225 degrees Celsius to Fahrenheit. This article delves into the intricacies of this conversion, starting with a comprehensive overview of the Celsius and Fahrenheit scales. We will explore how these two temperature scales differ and why understanding their relationship is essential. Next, we will provide a step-by-step guide on how to convert 225 degrees Celsius to Fahrenheit, ensuring accuracy and clarity. Finally, we will discuss the practical uses and implications of high temperatures, highlighting their significance in industrial, scientific, and everyday contexts. By grasping these concepts, readers will gain a deeper understanding of temperature measurements and their applications. Let's begin by understanding the Celsius and Fahrenheit scales.
Understanding the Celsius and Fahrenheit Scales
Understanding the Celsius and Fahrenheit scales is crucial for navigating various aspects of science, technology, and everyday life. These temperature scales, though different in their origins and applications, are fundamental tools in measuring thermal energy. To delve into the intricacies of these scales, it is essential to explore their historical development, key differences, and common applications. Historically, the development of these scales involved significant contributions from scientists such as Anders Celsius and Gabriel Fahrenheit, each with their own methodologies and reference points. The key differences between the two scales lie in their zero points, unit sizes, and practical uses. For instance, Celsius is widely used in scientific and international contexts due to its intuitive and linear scale, while Fahrenheit remains prevalent in certain regions like the United States for everyday weather forecasts. Understanding these distinctions is vital for accurate communication and application across different fields. By examining the historical development of these scales, we can better appreciate how they evolved to meet specific needs and how they continue to influence our understanding of temperature today. This journey begins with a look at the historical development of temperature scales.
Historical Development of Temperature Scales
The historical development of temperature scales is a fascinating narrative that spans centuries, reflecting the evolving understanding of thermal phenomena and the need for precise measurement. The earliest recorded attempts to measure temperature date back to ancient civilizations, such as the Greeks and Romans, who used primitive methods like observing the expansion and contraction of materials. However, it wasn't until the 16th century that the first thermoscopes were developed, marking the beginning of systematic temperature measurement. In the early 17th century, Italian scientist Santorio Santorio created a thermoscope that used air expansion to indicate temperature changes. This was followed by the work of Galileo Galilei, who improved upon this design. The next significant milestone came with the invention of the first mercury thermometer by Italian physicist Ferdinando II de' Medici in 1654. This innovation allowed for more accurate readings but still lacked a standardized scale. The development of standardized temperature scales began in earnest during the 18th century. In 1701, German physicist Ole Rømer proposed one of the first temperature scales, which divided the range between the freezing and boiling points of water into 60 degrees. However, it was not widely adopted. The modern era of temperature measurement began with the work of Swedish astronomer Anders Celsius in 1742. Celsius proposed a scale where 100 degrees separated the freezing and boiling points of water, with 0 degrees being the freezing point and 100 degrees being the boiling point. This scale, known as the Celsius scale, was initially inverted but was later reversed to its current form. Around the same time, German physicist Gabriel Fahrenheit developed his own scale in 1724. Fahrenheit's scale set the freezing point of water at 32 degrees and the boiling point at 212 degrees. Although it was widely used in the United States until recent decades, it has largely been replaced by the Celsius scale globally due to its simplicity and logical structure. The Fahrenheit scale's popularity in the United States can be attributed to its early adoption in scientific and everyday applications. However, as international scientific collaboration increased and metrication efforts gained momentum, the Celsius scale became the standard worldwide. Today, both scales are still in use, though the Celsius scale is more prevalent in scientific and international contexts. Understanding these historical developments provides a deeper appreciation for how our current temperature measurement systems evolved and why they remain essential tools in various fields from meteorology to chemistry. In summary, the historical development of temperature scales reflects a gradual progression from primitive observations to sophisticated measurement tools, culminating in the widely used Celsius and Fahrenheit scales that continue to play crucial roles in modern science and everyday life. This historical context underscores the importance of standardization and precision in temperature measurement, which is critical for accurate conversions like determining what 225 degrees Celsius is in Fahrenheit.
Key Differences Between Celsius and Fahrenheit
The Celsius and Fahrenheit scales are two distinct temperature measurement systems, each with its own set of characteristics and applications. The primary difference between the two lies in their zero points and the size of their units. The Celsius scale, also known as the centigrade scale, sets its zero point at the freezing point of water (0°C) and its boiling point at 100°C. In contrast, the Fahrenheit scale sets its zero point at a much lower temperature, where water freezes at 32°F and boils at 212°F. This means that for any given temperature, the Celsius value will be significantly lower than the Fahrenheit value. Another key difference is the size of the units. The Celsius scale has 100 units between the freezing and boiling points of water, while the Fahrenheit scale has 180 units between these same points. This results in a more granular measurement system for Celsius, making it more precise for scientific and everyday use. For example, a temperature change of 1°C is equivalent to a change of 1.8°F, highlighting the finer resolution of the Celsius scale. In terms of usage, Celsius is the standard temperature scale in most countries and is widely used in scientific research, medicine, and everyday applications due to its simplicity and logical structure. Fahrenheit, on the other hand, is predominantly used in the United States for weather forecasting and everyday temperature measurements. When converting between the two scales, specific formulas are used. To convert Celsius to Fahrenheit, you use the formula \(F = C \times \frac{9}{5} + 32\), where \(F\) is the temperature in Fahrenheit and \(C\) is the temperature in Celsius. Conversely, to convert Fahrenheit to Celsius, you use \(C = (F - 32) \times \frac{5}{9}\). These formulas are essential for accurately translating temperatures between the two scales. Understanding these differences is crucial for effective communication and accurate measurement across various fields. For instance, knowing that 225°C is equivalent to 437°F can be vital in industrial processes or cooking where precise temperature control is necessary. In summary, while both scales have their historical significance and practical uses, the Celsius scale's logical structure and global acceptance make it the preferred choice for most applications.
Common Applications of Each Scale
Understanding the Celsius and Fahrenheit scales is crucial for various applications across different fields. The Celsius scale, also known as the centigrade scale, is widely used in scientific and everyday contexts. It is the standard temperature scale in most countries and is preferred in scientific research due to its simplicity and logical structure. For instance, in chemistry and physics, the Celsius scale is used to measure the melting and boiling points of substances, with water freezing at 0°C and boiling at 100°C. In meteorology, weather forecasts often report temperatures in Celsius, making it easier for the general public to understand temperature variations. Additionally, in medicine, body temperature is commonly measured in Celsius, with normal body temperature ranging from 36.5°C to 37.5°C. On the other hand, the Fahrenheit scale is predominantly used in the United States for everyday applications. It is commonly seen in weather forecasts and household thermometers in the U.S. For example, in aviation, pilots often use Fahrenheit to report air temperatures, which can be critical for flight safety. In cooking, many American recipes specify temperatures in Fahrenheit, such as baking a cake at 350°F or roasting meat at 400°F. Furthermore, in HVAC (heating, ventilation, and air conditioning) systems, thermostats often display temperatures in Fahrenheit, making it easier for homeowners to adjust their heating and cooling settings. Both scales have historical significance and practical uses. The Fahrenheit scale was developed by Gabriel Fahrenheit in the early 18th century and was widely used until the Celsius scale gained international acceptance. Today, while the Celsius scale is more universally adopted, the Fahrenheit scale remains integral to certain industries and cultural practices. Understanding both scales is essential for effective communication and accurate measurement across diverse fields and regions. In summary, the Celsius scale is preferred in scientific research, international communication, and most everyday applications outside the U.S., while the Fahrenheit scale is still prevalent in the U.S. for various practical uses. Knowing how to convert between these two scales, such as converting 225°C to Fahrenheit (which is approximately 437°F), enhances one's ability to navigate different contexts efficiently. This dual understanding fosters better collaboration and accuracy in both professional and personal settings.
Converting 225 Celsius to Fahrenheit
Converting temperatures between Celsius and Fahrenheit is a fundamental skill that has numerous practical applications in various fields, including science, cooking, and everyday life. Understanding how to perform this conversion accurately is crucial for ensuring precision and safety. This article will guide you through the process of converting 225 degrees Celsius to Fahrenheit, leveraging three key components: **The Conversion Formula**, **Step-by-Step Calculation Process**, and **Real-World Examples of Temperature Conversions**. By mastering the conversion formula, you will be able to transform any Celsius value into its Fahrenheit equivalent with ease. The step-by-step calculation process will walk you through the specific steps required to convert 225 degrees Celsius to Fahrenheit, making the process clear and accessible. Finally, real-world examples will illustrate the practical significance of temperature conversions, helping you appreciate the relevance of this skill in everyday scenarios. Let's begin by delving into **The Conversion Formula**, which serves as the foundation for all temperature conversions between Celsius and Fahrenheit.
The Conversion Formula
The Conversion Formula is a straightforward mathematical equation used to convert temperatures from Celsius to Fahrenheit. This formula is essential for understanding and performing temperature conversions accurately. The formula is: \( \text{Fahrenheit} = (\text{Celsius} \times \frac{9}{5}) + 32 \). To illustrate its application, let's use the specific example of converting 225 degrees Celsius to Fahrenheit. By substituting 225 for Celsius in the formula, we get: \( \text{Fahrenheit} = (225 \times \frac{9}{5}) + 32 \). First, multiply 225 by 9, which equals 2025. Then divide this result by 5, yielding 405. Finally, add 32 to this result, giving us a final temperature of 437 degrees Fahrenheit. This step-by-step process demonstrates how the Conversion Formula simplifies and standardizes the conversion between these two temperature scales, making it an indispensable tool in various scientific, culinary, and everyday applications. Understanding and applying this formula ensures precision and consistency in temperature conversions, which is crucial in fields such as chemistry, cooking, and meteorology. Therefore, mastering the Conversion Formula is key to accurately converting temperatures between Celsius and Fahrenheit.
Step-by-Step Calculation Process
To convert 225 degrees Celsius to Fahrenheit, you need to follow a step-by-step calculation process. Here’s how you can do it: 1. **Start with the Celsius temperature**: You have 225 degrees Celsius. 2. **Apply the conversion formula**: The formula to convert Celsius to Fahrenheit is \( F = \frac{9}{5}C + 32 \), where \( C \) is the temperature in Celsius and \( F \) is the temperature in Fahrenheit. 3. **Plug in the value**: Substitute 225 for \( C \) in the formula: \( F = \frac{9}{5}(225) + 32 \). 4. **Perform the multiplication**: Calculate \( \frac{9}{5} \times 225 \): This equals \( 9 \times 45 = 405 \). 5. **Add 32**: Add 32 to the result from step 4: \( 405 + 32 = 437 \). 6. **Final result**: The temperature 225 degrees Celsius is equal to 437 degrees Fahrenheit. This process ensures accuracy and clarity in converting between these two common temperature scales, making it straightforward to understand and apply in various contexts. By following these steps, you can confidently convert any Celsius temperature to its Fahrenheit equivalent.
Real-World Examples of Temperature Conversions
Converting temperatures between Celsius and Fahrenheit is a common necessity in various real-world scenarios. For instance, in the automotive industry, understanding the operating temperatures of engines is crucial. A car engine might operate optimally at around 90°C (194°F), but if it exceeds 100°C (212°F), it could lead to overheating and potential damage. Similarly, in cooking, precise temperature control is essential; a perfectly roasted turkey requires an internal temperature of about 74°C (165°F), while baking a cake often involves temperatures around 180°C (356°F). In medical settings, body temperature is typically measured in Celsius, but healthcare professionals may need to convert it to Fahrenheit for patients who are more familiar with that scale; a normal body temperature is approximately 37°C (98.6°F). In meteorology, weather forecasts often report temperatures in both scales to cater to different audiences; for example, a summer day might reach highs of 32°C (90°F) or lows of -20°C (-4°F) in winter. These examples highlight the practical importance of temperature conversions in everyday life, making it essential to know how to convert between Celsius and Fahrenheit accurately, such as converting 225°C to Fahrenheit. This particular conversion is relevant in industrial processes where high temperatures are involved, such as in the production of steel or ceramics, where 225°C (437°F) might be a critical temperature point for material properties. Understanding these conversions ensures that professionals across various fields can communicate effectively and make informed decisions based on accurate temperature readings.
Practical Uses and Implications of High Temperatures
High temperatures play a pivotal role in various aspects of modern society, from industrial processes to scientific research and safety protocols. The practical uses and implications of high temperatures are multifaceted and far-reaching. In industrial settings, high temperatures are crucial for manufacturing processes such as metalworking, chemical synthesis, and energy production. These applications not only drive economic growth but also underpin the development of advanced technologies. However, the handling of high temperatures necessitates stringent safety considerations to prevent accidents and ensure worker safety. Additionally, scientific research involving high temperatures continues to expand our understanding of materials science, thermodynamics, and other fields, leading to breakthroughs that can transform industries. This article will delve into these three critical areas: the industrial applications of high temperatures, the safety considerations for handling them, and the scientific research that leverages these extreme conditions. We begin by examining the industrial applications of high temperatures, which form the backbone of many modern industries.
Industrial Applications of High Temperatures
High temperatures play a crucial role in various industrial applications, driving efficiency, quality, and innovation across multiple sectors. In the **metallurgy industry**, high temperatures are essential for processes such as smelting, refining, and alloying metals. For instance, steel production involves heating iron ore to temperatures above 1000°C to remove impurities and achieve the desired chemical composition. Similarly, in **ceramics manufacturing**, high temperatures are used to fire and sinter ceramic materials, enhancing their mechanical strength and durability. In the **chemical industry**, high-temperature reactions are fundamental for synthesizing a wide range of products. For example, the Haber-Bosch process for ammonia production requires temperatures around 450°C to facilitate the reaction between nitrogen and hydrogen. Additionally, **petroleum refining** relies on high-temperature processes like cracking and reforming to convert crude oil into various petroleum products such as gasoline, diesel, and jet fuel. The **energy sector** also heavily relies on high temperatures. In power plants, steam turbines operate at temperatures up to 600°C to generate electricity efficiently. Moreover, **nuclear reactors** use high temperatures to produce steam that drives turbines, highlighting the critical role of thermal energy in electricity generation. In **aerospace engineering**, materials are often tested and processed at extreme temperatures to ensure their performance under harsh conditions. This includes the development of heat-resistant materials for aircraft and spacecraft components. Furthermore, **advanced materials research** involves high-temperature treatments to create materials with unique properties, such as superconductors and nanomaterials. High temperatures are also integral to **waste management** through incineration processes that burn waste at temperatures exceeding 1000°C, reducing volume and eliminating pathogens. This method is particularly effective for hazardous waste disposal. In **food processing**, high temperatures are used for sterilization and pasteurization to ensure food safety. Techniques like retort processing involve heating food to high temperatures to kill bacteria and extend shelf life. Lastly, **research and development** in fields such as materials science and chemistry frequently require high-temperature experiments to study material properties and chemical reactions under extreme conditions. These diverse applications underscore the significance of high temperatures in driving industrial progress and innovation, enabling the production of a wide range of essential products and services.
Safety Considerations for Handling High Temperatures
When handling high temperatures, safety considerations are paramount to prevent injuries and ensure operational integrity. At temperatures such as 225 degrees Celsius (437 degrees Fahrenheit), the risk of burns, fires, and equipment damage is significantly elevated. **Personal Protective Equipment (PPE)** is essential; this includes heat-resistant gloves, face shields, and protective clothing that can withstand extreme heat. **Thermal Insulation** of equipment and tools is crucial to prevent accidental contact with hot surfaces. **Ventilation Systems** must be in place to remove heat and any hazardous fumes that may be generated. **Training and Procedures** are vital; personnel should be well-trained in handling high-temperature equipment and materials, and clear protocols should be established for emergency situations. **Regular Maintenance** of equipment is necessary to ensure that all components are functioning correctly and safely. **Temperature Monitoring** systems should be used to continuously track temperatures, providing real-time data to prevent overheating. **Fire Safety Measures** must be implemented, including the presence of fire extinguishers rated for high-temperature fires and a fire evacuation plan. **Chemical Compatibility** should be considered when working with materials at high temperatures, as some substances may react dangerously when heated. **Electrical Safety** is also critical; electrical components must be rated for high-temperature environments to prevent electrical fires. In addition, **Work Area Design** plays a significant role; workspaces should be designed to minimize the risk of accidents, with clear pathways and no obstructions. **Emergency Response Plans** should be developed and regularly practiced to ensure prompt and effective action in case of an incident. By adhering to these safety considerations, the risks associated with handling high temperatures can be significantly mitigated, ensuring a safe and efficient working environment.
Scientific Research Involving High Temperatures
Scientific research involving high temperatures is a multifaceted field that spans various disciplines, including materials science, physics, chemistry, and engineering. At the core of this research is the understanding and manipulation of materials under extreme thermal conditions. For instance, in materials science, high-temperature studies are crucial for developing advanced ceramics and composites used in aerospace and automotive industries. These materials must withstand temperatures exceeding 225°C (437°F) to ensure structural integrity and performance in harsh environments. In physics, researchers explore the behavior of superconducting materials at high temperatures, which has significant implications for energy transmission and storage. The discovery of high-temperature superconductors has opened new avenues for efficient energy systems, potentially revolutionizing power grids and transportation systems. Chemical reactions also undergo profound changes at elevated temperatures, making high-temperature chemistry a vital area of study. This field helps in understanding catalytic processes, combustion dynamics, and the synthesis of novel compounds with unique properties. Engineering applications of high-temperature research are equally compelling. In the field of nuclear engineering, understanding the behavior of materials at extreme temperatures is essential for designing safe and efficient reactors. Similarly, in the context of space exploration, materials must be able to withstand the intense heat generated during re-entry into Earth's atmosphere or when exposed to the harsh conditions of other planets. The practical uses and implications of high-temperature research are far-reaching. For example, in industrial processes such as steel production and chemical synthesis, high temperatures are critical for achieving desired outcomes efficiently. In biomedical research, high-temperature sterilization techniques are used to ensure the safety of medical instruments and equipment. Additionally, the study of high-temperature phenomena has led to advancements in thermal energy storage systems, which can help mitigate the intermittency of renewable energy sources by storing thermal energy for later use. Furthermore, environmental science benefits from high-temperature research through the study of volcanic activity and the Earth's mantle. Understanding these processes helps scientists predict natural hazards and gain insights into Earth's internal dynamics. The technological spin-offs from such research also contribute to the development of advanced sensors and monitoring systems capable of operating in extreme environments. In summary, scientific research involving high temperatures is a rich and diverse field with profound practical implications. From advancing materials science and engineering to enhancing our understanding of fundamental physical and chemical processes, this research drives innovation across multiple sectors, ultimately contributing to technological advancements and societal benefits.