What Is A Neutral Atom
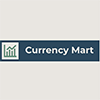
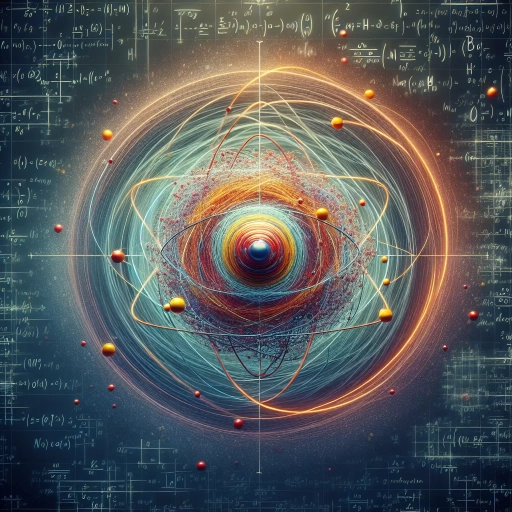
In the realm of atomic physics, understanding the fundamental nature of matter begins with the concept of a neutral atom. A neutral atom is a cornerstone in chemistry and physics, representing the basic building block of elements. This article delves into the intricacies of what constitutes a neutral atom, exploring its definition and basic principles, its components and properties, and how these atoms behave and interact with their environment. To grasp the essence of a neutral atom, it is crucial to first define what it is and the principles that govern its existence. This involves understanding the balance between protons and electrons that maintains its neutrality. Beyond this foundational knowledge, we must also examine the components that make up a neutral atom—protons, neutrons, and electrons—and their respective properties. Finally, understanding how these atoms interact with other particles and forces is essential for appreciating their role in chemical reactions and physical phenomena. In the following sections, we will delve into these aspects in detail. Starting with the **Definition and Basic Principles of a Neutral Atom**, we will lay the groundwork for a comprehensive understanding of this fundamental concept.
Definition and Basic Principles of a Neutral Atom
Understanding the definition and basic principles of a neutral atom is fundamental to grasping the intricacies of atomic physics. A neutral atom, by definition, is an atom that has an equal number of protons and electrons, resulting in no net charge. This balance is crucial for the stability and functionality of atoms within molecules and compounds. The article delves into three key aspects that underpin the concept of a neutral atom: **Atomic Structure Overview**, **Charge Balance in Atoms**, and **Stability of Neutral Atoms**. Firstly, an **Atomic Structure Overview** provides the foundational knowledge necessary to comprehend how protons, neutrons, and electrons interact within an atom. This section will explore the arrangement of these subatomic particles and their roles in forming a neutral atom. Secondly, **Charge Balance in Atoms** explains the critical equilibrium between positively charged protons and negatively charged electrons, which ensures that the atom remains neutral. Lastly, **Stability of Neutral Atoms** discusses how this balance contributes to the overall stability of the atom, preventing it from becoming ionized or reactive. By examining these principles, readers will gain a comprehensive understanding of what constitutes a neutral atom and why it is essential for chemical reactions and physical phenomena. Let us begin with an **Atomic Structure Overview** to lay the groundwork for this exploration.
Atomic Structure Overview
The atomic structure is the fundamental framework that defines the composition and behavior of a neutral atom, which is a cornerstone concept in chemistry and physics. At its core, an atom consists of three primary components: protons, neutrons, and electrons. **Protons** and **neutrons** reside in the nucleus, the central part of the atom, while **electrons** orbit around this nucleus. The number of protons in an atom determines its chemical element; for instance, hydrogen has one proton, helium has two, and so on. **Neutrons**, on the other hand, contribute to the overall mass of the atom but do not affect its chemical properties. The balance between protons and electrons is crucial for an atom to be neutral; each proton is matched by an electron, ensuring that the positive charge of the protons is offset by the negative charge of the electrons. The arrangement of electrons in an atom follows specific rules governed by quantum mechanics. Electrons are organized into energy levels or electron shells, each with a limited capacity for electron occupancy. The closest shell to the nucleus can hold up to two electrons, while subsequent shells can accommodate more electrons according to their energy level. This hierarchical structure is essential for understanding chemical bonding and reactivity, as it dictates how atoms interact with each other to form molecules. In addition to their arrangement, electrons also exhibit unique properties such as spin and orbital angular momentum. These properties influence how electrons fill available energy levels and how they interact with other electrons within the same atom or with those from neighboring atoms. The Pauli Exclusion Principle states that no two electrons in an atom can have the same set of quantum numbers, ensuring that each electron occupies a unique position within the atom's energy structure. Understanding atomic structure is pivotal for grasping various chemical phenomena. For example, the periodic table of elements is organized based on the number of protons (atomic number) and electron configuration. Elements in the same group (vertical column) share similar chemical properties due to having the same number of electrons in their outermost shell. This periodicity allows chemists to predict how different elements will react under various conditions. Moreover, knowledge of atomic structure underpins many technological advancements. In materials science, understanding how atoms arrange themselves in solids helps in designing new materials with specific properties such as conductivity or strength. In nuclear physics, the study of atomic nuclei has led to significant discoveries about nuclear reactions and energy production. In summary, the atomic structure provides a detailed blueprint for understanding how neutral atoms are composed and how they behave. By recognizing the roles of protons, neutrons, and electrons within an atom and their interactions governed by quantum mechanics principles, scientists can explain a wide range of chemical and physical phenomena. This foundational knowledge is essential for advancing fields such as chemistry, physics, materials science, and engineering.
Charge Balance in Atoms
Charge balance in atoms is a fundamental concept that underpins the stability and neutrality of atoms. In a neutral atom, the total positive charge of the protons in the nucleus is exactly balanced by the total negative charge of the electrons orbiting around it. This delicate balance is crucial because it ensures that the atom does not exhibit a net electric charge, which would otherwise make it an ion. To understand this balance, it's essential to consider the basic structure of an atom. At the heart of every atom lies the nucleus, which contains protons and neutrons. Protons carry a positive charge, while neutrons are neutral. The number of protons in an atom's nucleus defines its atomic number and determines the element to which the atom belongs. Surrounding the nucleus are electrons, which are negatively charged particles that occupy specific energy levels or electron shells. The principle of charge balance dictates that in a neutral atom, the number of protons (positive charges) must equal the number of electrons (negative charges). For instance, a hydrogen atom has one proton in its nucleus and one electron in its outermost shell, making it neutral. Similarly, an oxygen atom has eight protons and eight electrons, maintaining its neutrality. This balance is not just a matter of numerical equality but also involves the arrangement of electrons in their respective shells. According to the Aufbau principle and the Pauli exclusion principle, electrons fill up energy levels in a specific order, ensuring that each shell is filled before electrons start populating higher energy levels. This orderly arrangement helps maintain the stability of the atom by minimizing electron-electron repulsion and optimizing electron-nucleus attraction. The importance of charge balance extends beyond individual atoms; it also plays a critical role in chemical bonding and reactions. When atoms interact with each other to form molecules or compounds, they often do so by gaining or losing electrons to achieve a more stable electronic configuration. However, in these processes, the overall charge balance must still be maintained for the resulting molecule or compound to be stable. In summary, charge balance in atoms is a fundamental principle that ensures the neutrality and stability of atoms by equating the number of protons with the number of electrons. This balance is crucial for understanding atomic structure, chemical bonding, and the overall behavior of elements and their compounds. It underscores the intricate harmony between positive and negative charges within an atom, making it a cornerstone concept in chemistry and physics.
Stability of Neutral Atoms
The stability of neutral atoms is a fundamental concept in understanding the structure and behavior of matter at the atomic level. A neutral atom, by definition, has an equal number of protons and electrons, resulting in no net charge. This balance is crucial for the atom's stability because it ensures that the electrostatic forces within the atom are in equilibrium. The protons, positively charged particles, reside in the nucleus, while the electrons, negatively charged particles, orbit around it in electron shells or energy levels. The stability of a neutral atom can be attributed to several key principles. First, the electrostatic attraction between the positively charged protons and the negatively charged electrons holds the atom together. This attraction is balanced by the repulsive forces between electrons and between protons, which prevent the atom from collapsing or expanding indefinitely. The electrons occupy specific energy levels or orbitals, each with a limited capacity, as described by the Pauli Exclusion Principle. This principle states that no two electrons in an atom can have the same set of quantum numbers, ensuring that each electron occupies a unique position and energy state. Another critical factor contributing to stability is the arrangement of electrons in their respective shells. According to Hund's Rule and the Aufbau Principle, electrons fill the lowest available energy levels first, and each orbital is filled with electrons in a way that maximizes spin multiplicity. This orderly arrangement minimizes electron-electron repulsion and maximizes electron-nucleus attraction, thereby enhancing the overall stability of the atom. Furthermore, the stability of neutral atoms is also influenced by the concept of electron configuration. The electron configuration describes how electrons are distributed among the various energy levels or shells. For example, noble gases like helium and neon have full outer energy levels, which makes them particularly stable and unreactive. In contrast, atoms with partially filled outer shells tend to be more reactive as they seek to achieve a full outer shell configuration through chemical bonding. In addition to these principles, the stability of neutral atoms is reinforced by the concept of ionization energy and electron affinity. Ionization energy is the energy required to remove an electron from a neutral atom, while electron affinity is the energy released when an electron is added to a neutral atom. These energies are indicative of how tightly bound the electrons are to the nucleus and how stable the atom is in its neutral state. In summary, the stability of neutral atoms is a result of the delicate balance between electrostatic attractions and repulsions, the orderly arrangement of electrons in energy levels, and the specific electron configurations that minimize energy and maximize stability. Understanding these principles provides a solid foundation for comprehending the behavior and properties of neutral atoms, which are the building blocks of all matter.
Components and Properties of a Neutral Atom
Understanding the components and properties of a neutral atom is fundamental to grasping the building blocks of matter. A neutral atom, by definition, has an equal number of protons and electrons, ensuring it carries no net charge. This balance is crucial for the stability and identity of an element. To delve into the intricacies of a neutral atom, it is essential to explore three key aspects: the roles of protons, neutrons, and electrons; the significance of atomic mass and atomic number; and the structure of electron shells and orbitals. Protons, neutrons, and electrons are the primary constituents of an atom, each playing a distinct role in its composition. Protons reside in the nucleus and determine the element's identity, while neutrons contribute to the atom's mass without affecting its charge. Electrons orbit the nucleus, influencing chemical properties and interactions. The atomic mass and atomic number provide critical information about an atom's size and identity. The atomic number, equal to the number of protons, defines the element, while the atomic mass, which includes both protons and neutrons, gives an indication of the atom's overall mass. Lastly, electron shells and orbitals describe how electrons are arranged around the nucleus. This arrangement is vital for understanding chemical bonding and reactivity. By examining these components and properties, we gain a comprehensive understanding of the neutral atom's structure and behavior. Transitioning to the first supporting idea, let's begin with **Protons, Neutrons, and Electrons**, where we will explore in detail the roles and interactions of these fundamental particles within the atom.
Protons, Neutrons, and Electrons
In the intricate structure of a neutral atom, three fundamental components play crucial roles: protons, neutrons, and electrons. **Protons** are positively charged particles that reside in the nucleus, the central core of the atom. The number of protons in an atom determines its atomic number, which uniquely identifies each element. For instance, hydrogen has one proton, helium has two, and so on. Protons contribute to the overall positive charge of the nucleus and are essential for defining the chemical properties of an element. **Neutrons**, on the other hand, are neutral particles that also inhabit the nucleus alongside protons. Unlike protons, neutrons have no charge and thus do not affect the atom's overall charge. However, they do contribute to the atom's mass number when combined with protons. The mass number is the sum of protons and neutrons in an atom's nucleus. Neutrons play a vital role in stabilizing the nucleus; without them, many atoms would be unstable due to the repulsive forces between positively charged protons. **Electrons** are negatively charged particles that orbit around the nucleus in regions known as electron shells or energy levels. The number of electrons in a neutral atom is equal to the number of protons, ensuring that the positive and negative charges balance out, resulting in a net charge of zero. Electrons are arranged in specific patterns according to their energy levels and are responsible for chemical bonding and reactivity. The arrangement of electrons in an atom is governed by the principles of quantum mechanics and the Pauli exclusion principle, which dictate how many electrons can occupy each energy level. The interplay between these components is crucial for understanding atomic structure and behavior. For example, when an atom gains or loses electrons, it becomes an ion with a positive or negative charge, respectively. This process can significantly alter its chemical properties and reactivity. Additionally, variations in neutron numbers can lead to different isotopes of the same element, which have identical chemical properties but differ in mass. In summary, protons define an element's identity through their positive charge and presence in the nucleus; neutrons stabilize the nucleus and contribute to the atom's mass; while electrons determine chemical reactivity through their arrangement around the nucleus. Together, these components form a delicate balance that underpins the structure and properties of a neutral atom, making each element unique yet predictable in its behavior according to established physical laws. Understanding these components is essential for grasping fundamental concepts in chemistry and physics and for appreciating the intricate beauty of atomic structure.
Atomic Mass and Atomic Number
In the realm of atomic physics, two fundamental concepts are crucial for understanding the structure and properties of a neutral atom: atomic mass and atomic number. The **atomic number**, denoted by the symbol Z, is a unique identifier for each element and represents the number of protons present in the nucleus of an atom. This number determines the chemical properties of an element, as it dictates the number of electrons in a neutral atom, which in turn influences how atoms interact with each other. For instance, hydrogen has an atomic number of 1, indicating it has one proton in its nucleus, while helium has an atomic number of 2, signifying two protons. On the other hand, **atomic mass**, often symbolized as A, is a measure of the total mass of protons and neutrons in the nucleus of an atom. Unlike atomic number, atomic mass is not a whole number because it accounts for the varying masses of neutrons and the slight differences in mass between protons and neutrons. Atomic mass is typically expressed in atomic mass units (amu), where one amu is approximately equal to the mass of one proton or neutron. For example, carbon-12 has an atomic mass of exactly 12 amu by definition, while carbon-13 has an atomic mass of approximately 13 amu due to the additional neutron. The interplay between atomic number and atomic mass is pivotal in defining isotopes—atoms of the same element that have the same number of protons but different numbers of neutrons. Isotopes share identical chemical properties due to their equal atomic numbers but differ in physical properties such as mass and stability. For instance, carbon-12 and carbon-14 are isotopes of carbon; they both have six protons (Z=6) but differ in their neutron count, leading to distinct atomic masses. Understanding these concepts is essential for grasping various phenomena in chemistry and physics. The atomic number helps predict an element's position in the periodic table and its reactivity, while the atomic mass provides insights into an atom's stability and potential for radioactive decay. In scientific research and applications, precise knowledge of these values is critical for tasks ranging from nuclear reactions to forensic analysis. Moreover, the distinction between atomic number and atomic mass underscores the complexity and precision required in scientific inquiry. While the atomic number remains constant for all atoms of a given element, variations in atomic mass reflect the natural diversity within elements due to isotopic differences. This nuanced understanding not only enriches our comprehension of atomic structure but also highlights the intricate balance that governs the behavior of matter at its most fundamental level. In summary, the atomic number and atomic mass are foundational elements in the study of neutral atoms. The former defines an element's chemical identity and position in the periodic table, while the latter reflects the total mass of its nuclear constituents. Together, these concepts provide a comprehensive framework for understanding the intricate world of atomic physics and chemistry, enabling scientists to explore and explain a wide range of phenomena with precision and accuracy.
Electron Shells and Orbitals
In the context of understanding the components and properties of a neutral atom, it is crucial to delve into the concept of electron shells and orbitals. Electron shells, also known as energy levels or electron layers, are the regions around an atom's nucleus where electrons are found. These shells are quantized, meaning they can only occupy specific energy levels. The number of electron shells in an atom corresponds to the number of energy levels that electrons can occupy, with each shell having a unique capacity for electron accommodation. Within these electron shells, electrons reside in orbitals, which are three-dimensional regions that describe the probability of finding an electron within a particular energy level. There are four types of orbitals: s, p, d, and f. S-orbitals are spherical in shape and can hold up to two electrons. P-orbitals have a dumbbell shape and can accommodate up to six electrons. D-orbitals have more complex shapes and can hold up to ten electrons, while f-orbitals, with their intricate lobes, can contain up to fourteen electrons. The arrangement of electrons in these orbitals follows specific rules. The Aufbau principle states that electrons fill the lowest available energy levels first. The Pauli exclusion principle dictates that no two electrons in an atom can have the same set of quantum numbers, meaning each orbital can hold a maximum of two electrons with opposite spins. Additionally, Hund's rule specifies that when filling orbitals of equal energy, electrons occupy them singly and with parallel spins before pairing up. Understanding electron shells and orbitals is essential for explaining various atomic properties such as chemical reactivity, ionization energy, and electron affinity. For instance, atoms with full outer shells (noble gases) are chemically inert because they have a stable electron configuration. Conversely, atoms with partially filled outer shells are more reactive as they seek to achieve a full shell through chemical bonding. Moreover, the concept of electron shells and orbitals underpins the periodic table's structure. Elements in the same period (horizontal row) have the same number of electron shells but differ in the number of electrons in their outermost shell. Elements in the same group (vertical column) have similar chemical properties due to having the same number of electrons in their outermost shell, which determines their reactivity. In summary, electron shells and orbitals are fundamental to understanding the structure and behavior of neutral atoms. They provide a framework for explaining how electrons are arranged around the nucleus and how this arrangement influences an atom's chemical properties and reactivity. This knowledge is pivotal for understanding broader concepts in chemistry and physics, making it a cornerstone of atomic theory.
Behavior and Interactions of Neutral Atoms
Understanding the behavior and interactions of neutral atoms is a cornerstone of modern chemistry, offering insights into the fundamental principles that govern the physical world. This complex subject can be approached through several key perspectives. First, examining **Chemical Bonding and Reactions** reveals how neutral atoms form bonds with other atoms, leading to the creation of molecules and compounds. This process is crucial for understanding chemical transformations and the stability of substances. Second, delving into **Physical Properties and States** helps us comprehend how neutral atoms behave under different conditions, such as temperature and pressure, influencing their phase transitions and overall physical characteristics. Lastly, exploring **Interactions with Other Atoms and Molecules** sheds light on how neutral atoms engage with their surroundings, including intermolecular forces and the dynamics of atomic interactions. By grasping these concepts, we can better appreciate the intricate dance of atoms and their role in shaping the chemical universe. Let us begin by diving into the first of these critical areas: **Chemical Bonding and Reactions**.
Chemical Bonding and Reactions
Chemical bonding and reactions are fundamental processes that underpin the behavior and interactions of neutral atoms. At its core, chemical bonding involves the formation of chemical bonds between atoms, which are primarily driven by the desire to achieve a stable electronic configuration. Neutral atoms, having a balanced number of protons and electrons, seek to either gain, lose, or share electrons to mimic the noble gas configuration—a state of maximum stability. One primary type of chemical bond is the ionic bond, where one or more electrons are transferred from one atom to another. This transfer results in the formation of ions with opposite charges that attract each other, creating a strong electrostatic bond. For instance, when sodium (Na) reacts with chlorine (Cl), sodium loses an electron to become a positively charged ion (Na⁺), while chlorine gains an electron to become a negatively charged ion (Cl⁻). The electrostatic attraction between these ions forms sodium chloride (NaCl), commonly known as table salt. Another crucial type of bond is the covalent bond, where atoms share one or more pairs of electrons to achieve stability. This sharing can be equal, as in the case of hydrogen molecules (H₂) where each hydrogen atom shares its single electron with the other, or unequal, as seen in polar covalent bonds like those in water molecules (H₂O). Here, oxygen's greater electronegativity pulls the shared electrons closer to itself, creating a partial positive charge on the hydrogen atoms and a partial negative charge on the oxygen atom. Chemical reactions involve the breaking and forming of these chemical bonds. These reactions can be classified into various types such as synthesis, decomposition, single displacement, double displacement, and combustion reactions. For example, in a synthesis reaction like the formation of ammonia (NH₃), nitrogen gas (N₂) reacts with hydrogen gas (H₂) to form ammonia through the sharing of electrons: N₂ + 3H₂ → 2NH₃. This reaction illustrates how neutral atoms interact to form new compounds by establishing covalent bonds. The behavior and interactions of neutral atoms during these reactions are governed by several key principles. The law of conservation of mass states that matter cannot be created or destroyed in a chemical reaction; only transformed from one substance to another. Additionally, the law of definite proportions asserts that a chemical compound always contains its component elements in fixed ratio by mass. These principles underscore the predictable nature of chemical bonding and reactions. Understanding chemical bonding and reactions also involves recognizing the role of energy. Chemical reactions often involve either the absorption or release of energy in the form of heat, light, or other forms. Exothermic reactions release energy into the surroundings, while endothermic reactions absorb energy from the surroundings. For instance, combustion reactions like burning methane (CH₄) in oxygen (O₂) release heat and light: CH₄ + 2O₂ → CO₂ + 2H₂O. In conclusion, chemical bonding and reactions are integral to understanding how neutral atoms interact and behave. Through ionic and covalent bonding mechanisms, atoms achieve stability by forming compounds that adhere to fundamental chemical laws. These interactions not only explain the formation of molecules but also the transformations that occur during chemical reactions, highlighting the dynamic yet predictable nature of atomic behavior.
Physical Properties and States
Physical properties and states are fundamental aspects of understanding the behavior and interactions of neutral atoms. A neutral atom, by definition, has an equal number of protons and electrons, resulting in no net charge. The physical properties of these atoms, such as atomic mass, atomic radius, electronegativity, and ionization energy, play crucial roles in determining their behavior and interactions. Atomic mass, for instance, is a measure of the total number of protons and neutrons in the nucleus of an atom. This property influences the atom's density and its position in the periodic table, which in turn affects how it interacts with other atoms. The atomic radius, which varies across elements due to differences in electron configuration and nuclear charge, impacts the atom's reactivity and ability to form bonds with other atoms. Electronegativity, a measure of an atom's tendency to attract electrons in a covalent bond, is another key physical property. It influences the polarity of bonds and the overall stability of molecules. Ionization energy, the energy required to remove an electron from a neutral atom, is indicative of an atom's reactivity and its tendency to lose or gain electrons to form ions. The states of matter—solid, liquid, and gas—are also critical in understanding how neutral atoms interact. In solids, atoms are closely packed and have fixed positions within a crystal lattice, leading to strong interatomic forces that result in rigidity and resistance to changes in shape. In liquids, atoms are closer together but are free to move past one another, allowing for fluidity. Gases consist of widely spaced atoms that are free to move in any direction, resulting in high compressibility and expansibility. The transitions between these states—melting (solid to liquid), boiling (liquid to gas), sublimation (solid to gas), and condensation (gas to liquid)—are driven by changes in temperature and pressure. These phase changes involve significant alterations in the arrangement and motion of atoms, highlighting the dynamic nature of their interactions. Understanding these physical properties and states is essential for predicting how neutral atoms will behave under various conditions. For example, knowing the boiling point of an element can help predict its volatility and reactivity at different temperatures. Similarly, understanding electronegativity can help chemists design molecules with specific properties, such as polarity or stability. In summary, the physical properties of neutral atoms—such as atomic mass, atomic radius, electronegativity, and ionization energy—along with their states of matter, provide a comprehensive framework for understanding their behavior and interactions. These principles underpin much of chemistry and physics, enabling scientists to predict and manipulate the behavior of atoms in various contexts, from simple chemical reactions to complex biological processes. By grasping these fundamental concepts, researchers can better understand the intricate world of atomic interactions and develop new materials, technologies, and treatments that leverage this knowledge.
Interactions with Other Atoms and Molecules
Interactions with other atoms and molecules are fundamental to understanding the behavior of neutral atoms. These interactions are governed by the principles of quantum mechanics and electrostatic forces, which dictate how atoms engage with their surroundings. Neutral atoms, having an equal number of protons and electrons, do not carry a net charge but still participate in various types of interactions due to their electronic and nuclear properties. One primary type of interaction is the van der Waals force, a weak intermolecular force that arises from temporary dipoles formed in atoms or molecules. These dipoles can induce and attract other dipoles, leading to a weak attraction between neutral atoms. This force is crucial for understanding the physical properties of substances, such as melting and boiling points, as well as the behavior of gases. Another significant interaction involves the formation of chemical bonds. Although neutral atoms do not have a net charge, they can still form bonds through the sharing or transfer of electrons. Covalent bonds, for instance, are formed when two or more atoms share one or more pairs of electrons to achieve a stable electronic configuration. Ionic bonds, on the other hand, result from the transfer of electrons between atoms, leading to the formation of ions that are electrostatically attracted to each other. In addition to these bonding interactions, neutral atoms can also engage in non-covalent interactions such as hydrogen bonding and π-π stacking. Hydrogen bonding occurs when a hydrogen atom bonded to a highly electronegative atom (like oxygen, nitrogen, or fluorine) is attracted to another electronegative atom in a different molecule or within the same molecule if it is sufficiently far apart. This type of interaction is particularly important in biological systems, such as DNA and proteins, where it plays a crucial role in maintaining structure and function. Furthermore, neutral atoms can interact through electromagnetic forces when they are excited or ionized. For example, when an atom absorbs energy from an external source (like light), its electrons can transition to higher energy levels. This excited state can lead to interactions with other atoms or molecules through processes such as fluorescence or phosphorescence, where the atom releases excess energy as light. In summary, the interactions of neutral atoms with other atoms and molecules are multifaceted and critical for understanding various physical and chemical phenomena. From van der Waals forces that influence macroscopic properties to covalent and ionic bonds that form the basis of molecular structures, these interactions underpin much of what we observe in nature and utilize in technology. By grasping these fundamental interactions, we gain deeper insights into the behavior of neutral atoms and their role in shaping our world.