What Is The Particle Theory
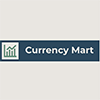
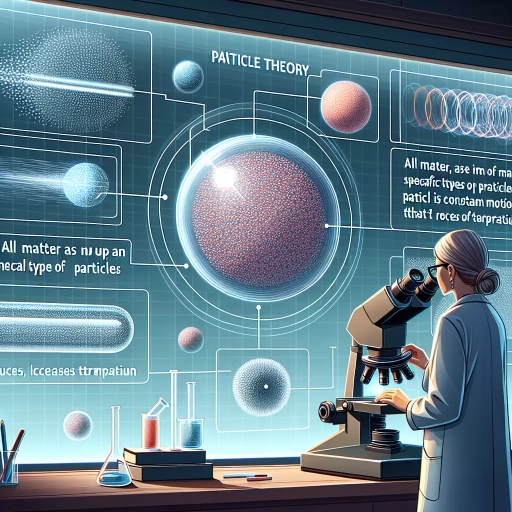
The particle theory, a fundamental concept in physics and chemistry, provides a comprehensive framework for understanding the behavior and properties of matter at its most basic level. This theory posits that all matter is composed of tiny, indivisible particles known as atoms or molecules, which interact with each other in specific ways. In this article, we will delve into the intricacies of the particle theory, starting with an **Introduction to the Particle Theory**, where we will explore its historical development and key principles. We will then examine the **Core Concepts of the Particle Theory**, including the characteristics of particles, their interactions, and the laws governing their behavior. Finally, we will discuss the **Applications and Implications of the Particle Theory**, highlighting how this theory has revolutionized various fields such as chemistry, physics, and engineering. By understanding these aspects, readers will gain a deeper appreciation for the significance and far-reaching impact of the particle theory. Let us begin by introducing the foundational elements of this pivotal scientific concept.
Introduction to the Particle Theory
The Particle Theory, a cornerstone of modern physics, has revolutionized our understanding of the fundamental nature of matter and energy. This theory, which posits that everything is composed of tiny, indivisible particles, has a rich historical background that spans centuries. From ancient Greek philosophers like Democritus to the groundbreaking experiments of modern scientists, the evolution of the Particle Theory has been a journey marked by curiosity and innovation. At its core, the theory is built on key principles and assumptions that explain how these particles interact and behave. Understanding these principles is crucial for grasping the theory's significance. The importance of the Particle Theory in modern science cannot be overstated; it underpins many technological advancements and scientific discoveries, influencing fields from chemistry to quantum mechanics. In this article, we will delve into the historical background of the Particle Theory, explore its key principles and assumptions, and discuss its importance in modern science, providing a comprehensive introduction to this foundational concept. **Introduction to the Particle Theory**
Historical Background of the Particle Theory
The historical background of the particle theory is a rich and evolving narrative that spans centuries, reflecting the gradual shift from ancient philosophical speculations to modern scientific discoveries. The concept of particles as fundamental building blocks of matter dates back to ancient Greece, where philosophers such as Democritus (c. 460–370 BCE) proposed the idea of atoms as indivisible, eternal units. This early atomic theory was largely speculative but laid the groundwork for later scientific inquiry. In the 17th and 18th centuries, scientists like Pierre Gassendi and Antoine Lavoisier revived interest in atomic theories, with Lavoisier's work on chemical elements providing a more empirical basis for understanding matter. The 19th century saw significant advancements with the development of the kinetic theory of gases by scientists such as Ludwig Boltzmann and James Clerk Maxwell. This theory posited that gases consist of tiny particles in constant motion, which helped explain various physical phenomena like pressure and temperature. The discovery of the electron by J.J. Thomson in 1897 marked a pivotal moment, as it introduced the concept of subatomic particles and challenged the traditional view of atoms as indivisible. Early in the 20th century, Ernest Rutherford's famous gold foil experiment (1909) revealed the nucleus of an atom, leading to the development of the Rutherford model. This was later refined by Niels Bohr's model (1913), which introduced energy levels and electron shells. The discovery of the neutron by James Chadwick in 1932 completed the basic structure of the atom as we understand it today. The mid-20th century witnessed the dawn of particle physics with the discovery of subatomic particles beyond electrons, protons, and neutrons. The invention of particle accelerators enabled scientists to study these particles in detail, leading to the discovery of mesons, baryons, and other hadrons. The quark model proposed by Murray Gell-Mann and George Zweig in the 1960s further elucidated the structure of hadrons, suggesting that they are composed of even smaller particles called quarks. The Standard Model of particle physics, developed in the 1970s and 1980s, integrated these findings into a comprehensive framework that describes the behavior of fundamental particles and forces. This model includes quarks, leptons (such as electrons), and gauge bosons (like photons), along with the Higgs boson discovered in 2012 at CERN's Large Hadron Collider. Today, particle theory continues to evolve with ongoing research into dark matter, dark energy, and beyond the Standard Model physics, pushing our understanding of the universe to new frontiers. This historical journey underscores how the particle theory has transformed from a philosophical concept to a robust scientific framework that underpins our modern understanding of the physical world.
Key Principles and Assumptions
The particle theory, a foundational concept in physics and chemistry, is built upon several key principles and assumptions that underpin our understanding of the behavior and properties of matter. At its core, the particle theory posits that all matter is composed of tiny, indivisible particles known as atoms or molecules. A crucial assumption here is the **atomic hypothesis**, which states that these particles are the fundamental building blocks of all substances. This idea was first proposed by ancient Greek philosophers such as Democritus and later refined by scientists like John Dalton in the 19th century. One of the primary principles of the particle theory is the **Law of Conservation of Mass**, which asserts that matter cannot be created or destroyed in a chemical reaction. This principle underscores the idea that the total mass of reactants equals the total mass of products, reinforcing the notion that atoms are conserved during chemical transformations. Another key principle is the **Law of Definite Proportions**, which states that a chemical compound always contains its component elements in fixed ratios by mass, regardless of its source or method of preparation. This law supports the atomic theory by indicating that compounds are formed from atoms in specific, whole-number ratios. The particle theory also relies on the assumption of **random motion**. According to this, particles in a substance are in constant motion due to thermal energy. This concept is central to understanding phenomena such as diffusion, Brownian motion, and the behavior of gases as described by the kinetic molecular theory. Additionally, the theory assumes that particles have **intermolecular forces**, which are responsible for the physical properties of substances like melting and boiling points, viscosity, and surface tension. Furthermore, the particle theory incorporates the concept of **discrete energy levels**. This principle, particularly relevant in quantum mechanics, suggests that particles can only occupy specific energy states rather than any arbitrary energy level. This idea is fundamental to understanding atomic spectra and chemical bonding. In summary, the particle theory is grounded in a set of well-defined principles and assumptions that collectively explain the nature and behavior of matter at its most fundamental level. These principles not only provide a framework for understanding chemical reactions and physical properties but also serve as a basis for more advanced theories in physics and chemistry, making them indispensable tools for scientific inquiry and discovery. By grasping these foundational concepts, we can better appreciate the intricate mechanisms governing our physical world and continue to advance our knowledge of the universe.
Importance in Modern Science
In the realm of modern science, the particle theory stands as a cornerstone of our understanding of the physical world. This fundamental concept, which posits that matter is composed of discrete particles rather than being continuous, has revolutionized various fields including physics, chemistry, and materials science. The importance of the particle theory lies in its ability to explain a wide range of phenomena at both microscopic and macroscopic levels. For instance, in physics, the particle theory underpins quantum mechanics and the Standard Model of particle physics, which describe the behavior of subatomic particles like electrons, protons, and neutrons. These particles are the building blocks of atoms, and their interactions govern chemical reactions and the properties of materials. In chemistry, the particle theory is crucial for understanding chemical bonding and reactions. The concept of atoms as particles allows chemists to predict how different elements will react with each other based on their atomic structure. This knowledge is essential for developing new materials with specific properties, such as conductive polymers or nanomaterials. Moreover, the particle theory is pivotal in fields like materials science and engineering, where understanding the behavior of particles at the atomic and subatomic level enables the design of advanced materials with tailored properties. The particle theory also has significant implications for technological advancements. For example, in electronics, the understanding of electron behavior as particles is crucial for the development of transistors and other semiconductor devices that form the backbone of modern electronics. Similarly, in medical science, particle physics informs our understanding of radiation therapy and imaging techniques such as positron emission tomography (PET scans), which rely on the interaction of subatomic particles. Furthermore, the particle theory has led to groundbreaking discoveries that continue to shape our understanding of the universe. The discovery of particles like quarks and gluons has provided insights into the strong nuclear force, while the detection of the Higgs boson has confirmed key aspects of the Standard Model. These findings not only deepen our knowledge but also inspire new areas of research and innovation. In summary, the particle theory is a foundational principle in modern science that bridges multiple disciplines by providing a unified framework for understanding matter at its most fundamental level. Its importance extends from explaining basic chemical reactions to driving technological innovations and advancing our comprehension of the universe itself. As we delve into the intricacies of particle theory, we uncover a rich tapestry of scientific knowledge that continues to evolve and expand our understanding of reality.
Core Concepts of the Particle Theory
The Particle Theory, a cornerstone of modern physics, offers a profound understanding of the fundamental nature of matter and energy. At its core, this theory posits that all substances are composed of tiny, indivisible particles that interact and behave in specific ways. This introduction will delve into the essential concepts that underpin the Particle Theory, setting the stage for a deeper exploration of its key aspects. We will begin by examining the **Definition and Types of Particles**, where we will discuss the various kinds of particles that constitute matter, from atoms and molecules to subatomic particles like electrons, protons, and neutrons. Next, we will explore **Behavior and Interactions of Particles**, highlighting how these particles move, collide, and combine to form different substances. Finally, we will venture into **Quantum Mechanics and Particle Theory**, where we will uncover how quantum principles govern the behavior of particles at the atomic and subatomic level. By understanding these core concepts, we can gain a comprehensive insight into the Particle Theory and its far-reaching implications. Transitioning seamlessly from these foundational ideas, we will introduce the **Introduction to the Particle Theory**, providing a cohesive framework for grasping this pivotal scientific concept.
Definition and Types of Particles
The particle theory, a foundational concept in physics and chemistry, posits that matter is composed of tiny, indivisible particles. At the heart of this theory lies the definition and classification of these particles, which are crucial for understanding the behavior and properties of matter. **Particles** are defined as the smallest units of a substance that retain the chemical properties of that substance. These particles can be broadly categorized into several types, each with distinct characteristics. 1. **Atoms**: The most fundamental particles are atoms, which are the building blocks of elements. An atom consists of a nucleus containing protons and neutrons, surrounded by electrons in orbitals. Atoms are the smallest units of an element that still retain the properties of that element. 2. **Molecules**: When atoms bond together, they form molecules. Molecules are groups of two or more atoms that share electrons to achieve a stable electronic configuration. The type and arrangement of atoms within a molecule determine its chemical and physical properties. 3. **Ions**: Ions are atoms or groups of atoms that have gained or lost electrons, resulting in a net positive or negative charge. Cations are positively charged ions formed by losing electrons, while anions are negatively charged ions formed by gaining electrons. 4. **Free Radicals**: Free radicals are highly reactive particles that contain unpaired electrons. These unpaired electrons make free radicals highly reactive as they seek to form bonds with other molecules to achieve stability. 5. **Subatomic Particles**: Within atoms, there are even smaller particles known as subatomic particles. These include protons, neutrons, and electrons. Protons and neutrons reside in the nucleus, while electrons orbit around it. Protons carry a positive charge, neutrons have no charge, and electrons carry a negative charge. Understanding these types of particles is essential for grasping core concepts such as chemical bonding, stoichiometry, and the behavior of gases, liquids, and solids. The particle theory explains how these particles interact and combine to form various substances and how their interactions influence the macroscopic properties of matter. By recognizing the different types of particles and their roles, scientists can predict and explain a wide range of phenomena in chemistry and physics, from the simplest chemical reactions to complex biological processes. This foundational knowledge underpins much of modern science and technology, making it a critical component of scientific literacy.
Behavior and Interactions of Particles
The behavior and interactions of particles are fundamental aspects of the particle theory, which underpins our understanding of the physical world. At its core, the particle theory posits that matter is composed of tiny, indivisible particles such as atoms and molecules. These particles are in constant motion, even at absolute zero, due to thermal energy. The interactions among these particles dictate the properties and behaviors of substances. In gases, particles are widely spaced and move freely in any direction, colliding with each other and the walls of their container. This random motion explains the pressure exerted by gases and their ability to expand and fill containers. In liquids, particles are closer together but still have enough kinetic energy to slide past one another, allowing for fluidity. Solids, on the other hand, have particles that are tightly packed and vibrate in place, giving them rigidity. The interactions between particles also influence phase changes. For instance, when a solid is heated, the increased kinetic energy of its particles allows them to break free from their fixed positions and move more freely, transitioning into a liquid state. Similarly, cooling a gas reduces the kinetic energy of its particles, causing them to come together and form droplets or crystals. Chemical reactions are another manifestation of particle interactions. Atoms or molecules collide with sufficient energy and proper orientation to form new bonds or break existing ones, resulting in the creation of new substances. The principles of quantum mechanics further refine our understanding by introducing wave-particle duality and the probabilistic nature of particle behavior, explaining phenomena such as electron spin and orbital configurations. Moreover, the behavior of particles at different scales—from atomic to subatomic—reveals intricate patterns governed by specific laws. Electrons orbiting nuclei follow the rules of quantum mechanics, while protons and neutrons within nuclei adhere to nuclear forces. Understanding these interactions is crucial for explaining phenomena like radioactivity, where unstable nuclei emit particles to achieve stability. In summary, the behavior and interactions of particles form the backbone of the particle theory. By examining how these tiny entities move, collide, and bond with each other, we gain insights into the properties of matter across various states and scales. This foundational knowledge not only explains everyday phenomena but also underpins advanced scientific disciplines such as chemistry and physics.
Quantum Mechanics and Particle Theory
Quantum Mechanics and Particle Theory are intricately linked, forming the cornerstone of modern physics. At its core, Quantum Mechanics is a theoretical framework that describes the behavior of matter and energy at the smallest scales, where classical physics no longer applies. This realm is governed by principles such as wave-particle duality, superposition, and entanglement. In this context, particles like electrons and photons exhibit both wave-like and particle-like properties, challenging our intuitive understanding of reality. Particle Theory, particularly within the Standard Model of particle physics, builds upon these quantum principles to explain the fundamental nature of matter and forces. The Standard Model posits that all matter is composed of elementary particles such as quarks and leptons, which are the building blocks of protons, neutrons, and electrons. These particles interact via fundamental forces mediated by gauge bosons like photons (for electromagnetism), gluons (for the strong nuclear force), and W and Z bosons (for the weak nuclear force). The Higgs boson, discovered in 2012, plays a crucial role in explaining how particles acquire mass. A key aspect of Particle Theory is the concept of fields, which permeate space and time. Each type of particle corresponds to a specific field; for instance, the electromagnetic field is associated with photons. These fields are quantized, meaning they come in discrete packets (quanta) rather than being continuous. This quantization is a direct result of quantum mechanics and underpins our understanding of particle interactions. The predictive power of Quantum Mechanics and Particle Theory has been extensively validated through numerous experiments. For example, the existence of antiparticles (predicted by Dirac's equation) and the precise measurements of particle properties in high-energy collisions have consistently supported theoretical predictions. Furthermore, phenomena such as quantum tunneling and quantum entanglement have been experimentally confirmed, reinforcing our confidence in these theories. In summary, Quantum Mechanics provides the foundational principles for understanding particle behavior, while Particle Theory, through the Standard Model, offers a comprehensive framework for describing the fundamental constituents of matter and their interactions. Together, these theories have revolutionized our understanding of the universe at its most basic level, enabling groundbreaking technological advancements and continuing to inspire new areas of research in physics.
Applications and Implications of the Particle Theory
The Particle Theory, a cornerstone of modern physics and chemistry, has far-reaching implications that span multiple disciplines and technological advancements. This theory, which posits that matter is composed of discrete particles such as atoms and subatomic particles, underpins our understanding of the physical world. In this article, we will delve into the multifaceted applications and implications of the Particle Theory, exploring its pivotal role in both chemistry and physics. We will examine how this fundamental concept drives technological innovations, from semiconductor manufacturing to medical imaging. Additionally, we will discuss future research directions and potential discoveries that could emerge from continued exploration of particle theory. By understanding the intricacies and applications of the Particle Theory, we can better appreciate its transformative impact on science and technology. This introduction sets the stage for a deeper exploration into the Role in Chemistry and Physics, Technological Innovations Based on Particle Theory, and Future Research Directions and Potential Discoveries, ultimately leading to a comprehensive Introduction to the Particle Theory.
Role in Chemistry and Physics
The particle theory, a fundamental concept in both chemistry and physics, plays a pivotal role in understanding the behavior and properties of matter at its most basic level. In chemistry, the particle theory is essential for explaining the structure and interactions of atoms and molecules. It posits that matter is composed of tiny particles called atoms, which are further divided into protons, neutrons, and electrons. This theory underpins the periodic table, allowing chemists to predict chemical properties and reactions based on the arrangement of electrons around the nucleus. For instance, the valence shell electron pair repulsion (VSEPR) theory relies on particle theory to explain the shapes of molecules, which is crucial for understanding chemical bonding and reactivity. Additionally, the kinetic molecular theory, a subset of particle theory, describes the behavior of gases by assuming that gas molecules are in constant random motion, colliding with each other and the walls of their container. This theory is vital for understanding gas laws and thermodynamic processes. In physics, the particle theory is equally significant, particularly in the realm of quantum mechanics and particle physics. Here, particles such as electrons, photons, and quarks are studied in detail to understand their properties and interactions. Quantum mechanics, which is built on the principles of wave-particle duality and uncertainty, explains how particles can exhibit both wave-like and particle-like behavior. This theory has led to numerous technological advancements, including the development of transistors, lasers, and computer chips. Furthermore, particle physics delves into the nature of subatomic particles and forces, such as electromagnetism and the strong and weak nuclear forces. The Standard Model of particle physics, which describes all known subatomic particles and their interactions, is a direct application of the particle theory. This model has been instrumental in predicting the existence of new particles, such as the Higgs boson, which was discovered at CERN's Large Hadron Collider in 2012. The applications and implications of the particle theory are far-reaching and diverse. In materials science, understanding the behavior of particles at the atomic and subatomic level allows for the design of new materials with specific properties, such as superconductors and nanomaterials. In medicine, particle theory underpins various diagnostic and therapeutic techniques, including positron emission tomography (PET) scans and radiation therapy. Additionally, the principles of particle theory are crucial for energy production, particularly in nuclear power plants where nuclear reactions involve the interaction of subatomic particles. Overall, the particle theory serves as a foundational framework that bridges chemistry and physics, enabling scientists to explore and explain the intricate world of matter at its most fundamental level. Its applications continue to drive innovation across various fields, highlighting its enduring importance in modern science.
Technological Innovations Based on Particle Theory
Technological innovations based on particle theory have revolutionized various fields, transforming the way we live, work, and interact. At the heart of these advancements is the understanding that matter is composed of tiny, indivisible particles such as atoms and subatomic particles like electrons, protons, and neutrons. This fundamental concept has led to breakthroughs in materials science, electronics, medicine, and energy production. In materials science, particle theory underpins the development of nanotechnology. By manipulating atoms and molecules at the nanoscale, scientists can create materials with unique properties such as superconductors, nanotubes, and graphene. These materials are crucial for enhancing the performance of electronic devices, improving energy storage in batteries, and creating advanced composites for aerospace and automotive industries. The electronics sector has also seen significant advancements due to particle theory. The transistor, a cornerstone of modern electronics, relies on the behavior of electrons in semiconductors. This understanding has enabled the miniaturization of electronic components, leading to the development of smaller, faster, and more efficient devices such as smartphones and laptops. Furthermore, quantum computing—a field that leverages the principles of quantum mechanics to process information—promises to solve complex problems that are currently unsolvable by classical computers. In medicine, particle theory informs the development of diagnostic and therapeutic tools. Positron Emission Tomography (PET) scans, for instance, use positrons (the antiparticles of electrons) to create detailed images of the body's internal structures. This technology aids in diagnosing diseases like cancer and neurological disorders. Additionally, radiation therapy in cancer treatment relies on the controlled use of high-energy particles to destroy cancer cells while minimizing damage to healthy tissue. Energy production has also been transformed by particle theory. Nuclear power plants generate electricity by harnessing the energy released from the fission of atomic nuclei. This process involves splitting heavy atoms into lighter ones, releasing a vast amount of energy in the form of heat, which is then converted into electricity. Moreover, research into fusion energy—the process of combining light atomic nuclei to form heavier ones—holds promise for a nearly limitless and clean source of energy. In conclusion, technological innovations grounded in particle theory have far-reaching implications across multiple disciplines. From enhancing material properties to revolutionizing electronics and medicine, these advancements underscore the profound impact of understanding the smallest building blocks of matter. As research continues to delve deeper into the mysteries of particles and their interactions, we can expect even more transformative technologies to emerge, shaping our future in profound ways.
Future Research Directions and Potential Discoveries
As we delve into the future research directions and potential discoveries within the realm of particle theory, several exciting avenues emerge that promise to revolutionize our understanding of the universe. One of the most compelling areas is the exploration of dark matter and dark energy, which collectively account for approximately 95% of the universe's mass-energy budget yet remain shrouded in mystery. Future experiments, such as those involving highly sensitive detectors and advanced telescopes, are poised to uncover the properties and behaviors of these elusive components. For instance, the next generation of particle colliders, like the Future Circular Collider (FCC), could potentially create conditions that allow for the direct detection of dark matter particles. Another significant direction involves the study of neutrinos, which have already revealed surprising insights into the universe's fundamental laws. Ongoing and future experiments, such as DUNE (Deep Underground Neutrino Experiment) and Hyper-Kamiokande, aim to elucidate neutrino properties further, including their mass hierarchy and CP violation. These findings could not only deepen our understanding of particle physics but also shed light on cosmological phenomena like the matter-antimatter asymmetry in the universe. The intersection of particle physics and cosmology is another fertile ground for research. Theoretical frameworks such as inflationary models and string theory continue to be refined, offering potential explanations for the universe's early moments and the nature of space-time itself. Upcoming missions like the Simons Observatory and CMB-S4 will provide unprecedented data on the cosmic microwave background, allowing scientists to test these theories with greater precision. Furthermore, advancements in computational power and machine learning algorithms are expected to play a crucial role in analyzing the vast datasets generated by modern particle detectors. This synergy between experimental physics and data science could lead to novel methods for identifying patterns and anomalies that might otherwise go unnoticed, potentially uncovering new particles or interactions. Lastly, the quest for a unified theory that reconciles quantum mechanics with general relativity remains a driving force behind many research endeavors. Theoretical constructs such as loop quantum gravity and certain versions of string theory are being actively explored, with hopes that they might provide a consistent framework for understanding all fundamental forces and particles. While these pursuits are inherently challenging, they hold the promise of transforming our comprehension of reality at its most fundamental level. In summary, future research in particle theory is set to explore some of the most profound mysteries in physics, from dark matter to unified theories. These investigations not only have the potential to reveal groundbreaking discoveries but also to reshape our understanding of the cosmos and its underlying laws. As scientists continue to push the boundaries of knowledge, we can anticipate a future filled with exciting revelations that will further illuminate the intricate tapestry of the universe.