What Is Peglyte
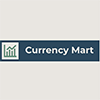
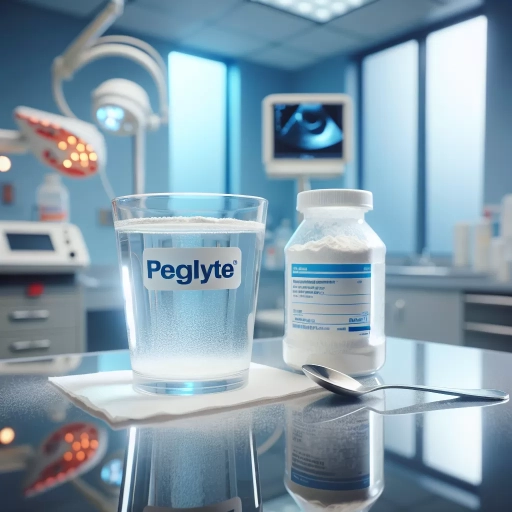
Pegylation, a process involving the covalent attachment of polyethylene glycol (PEG) to molecules such as proteins, peptides, and small molecules, has revolutionized various fields including pharmaceuticals, biotechnology, and medicine. This innovative technique enhances the solubility, stability, and bioavailability of therapeutic agents, making them more effective and safer for use. In this article, we will delve into the intricacies of pegylation, exploring its fundamental principles, diverse applications, and the benefits and challenges associated with it. First, we will **Understand Pegylated Compounds**, examining how PEG molecules interact with other substances to alter their pharmacokinetic properties. Next, we will discuss the **Applications and Uses of Pegylated Compounds**, highlighting their role in drug delivery systems, diagnostic tools, and other biomedical applications. Finally, we will analyze the **Benefits and Challenges of Pegylation**, considering both the advantages that have made pegylation a cornerstone in modern drug development and the hurdles that researchers face in optimizing this technology. By understanding these aspects, we can appreciate the full potential of pegylation and its impact on advancing healthcare. Let's begin by **Understanding Pegylated Compounds**.
Understanding Pegylated Compounds
Understanding pegylated compounds is a multifaceted topic that encompasses several key aspects, each crucial for a comprehensive grasp of these molecules. At the heart of this understanding lies the **Definition and Chemical Structure**, which provides the foundational knowledge necessary to appreciate the intricacies of pegylation. However, to fully contextualize pegylated compounds, it is also essential to delve into their **History and Development**, tracing the evolution from early research to current applications. This historical perspective highlights the scientific breakthroughs and challenges that have shaped the field. Additionally, exploring the **Types of Pegylation** reveals the diverse methods and strategies employed to modify molecules, each with its own set of advantages and limitations. By examining these three dimensions—definition, history, and types—we can gain a nuanced insight into how pegylation enhances the properties of therapeutic agents, making them more effective and safer for use. Let us begin by examining the **Definition and Chemical Structure** of pegylated compounds, which forms the basis of our understanding.
Definition and Chemical Structure
**Definition and Chemical Structure** Pegylated compounds, a cornerstone in modern pharmaceuticals, are defined as molecules that have been chemically modified by the covalent attachment of polyethylene glycol (PEG) chains. This process, known as pegylation, significantly alters the physical and chemical properties of the parent molecule, enhancing its therapeutic efficacy and safety profile. The chemical structure of pegylated compounds involves the conjugation of PEG, a hydrophilic polymer composed of repeating ethylene oxide units, to a target molecule such as a protein, peptide, or small molecule drug. The PEG polymer itself is typically linear or branched and can vary in molecular weight, ranging from a few hundred to several thousand Daltons. The conjugation process usually involves a linker molecule that facilitates the attachment between the PEG chain and the therapeutic agent. Common linkers include succinimidyl esters, maleimides, and aldehydes, which react with functional groups on the target molecule like amines, thiols, or carboxyls. This precise engineering ensures that the pegylated compound retains its biological activity while gaining improved solubility, reduced immunogenicity, and extended plasma half-life due to the shielding effect provided by the PEG chains. The specific chemical structure of a pegylated compound can be tailored to optimize its pharmacokinetic and pharmacodynamic properties. For instance, the molecular weight of the PEG chain can be adjusted to influence the rate of renal clearance or the degree of cellular uptake. Additionally, the site of PEG attachment on the target molecule can be strategically chosen to minimize interference with its active site or binding regions. This meticulous design allows pegylated compounds to achieve better therapeutic outcomes by prolonging their circulation time, reducing dosing frequency, and minimizing adverse effects. Understanding the definition and chemical structure of pegylated compounds is crucial for appreciating their role in drug development and clinical applications. By leveraging the unique properties imparted by PEGylation, researchers and clinicians can develop more effective treatments for a wide range of diseases, from cancer and autoimmune disorders to genetic deficiencies and infectious diseases. The versatility and efficacy of pegylated compounds underscore their importance in contemporary medicine, making them a focal point in ongoing research aimed at advancing therapeutic strategies.
History and Development
The history and development of pegylated compounds are deeply intertwined with advancements in pharmaceutical science and biotechnology. The concept of pegylation, which involves the covalent attachment of polyethylene glycol (PEG) to molecules such as proteins, peptides, and small molecules, has its roots in the early 1970s. Initially, researchers like Frank Davis and Abraham Abuchowski explored the potential of PEG to enhance the solubility and stability of therapeutic agents. Their pioneering work demonstrated that PEGylation could significantly prolong the circulation time of proteins in the bloodstream, reduce immunogenicity, and improve overall therapeutic efficacy. Over the decades, this technology has evolved through rigorous scientific inquiry and technological innovation. In the 1980s, the first pegylated proteins were developed for clinical use, marking a significant milestone in drug delivery systems. One of the earliest approved pegylated drugs was peginterferon alfa-2a, used in the treatment of hepatitis B and C. This breakthrough set the stage for further research into various applications of pegylation across different therapeutic areas. The 1990s saw a surge in the development of new pegylation techniques and reagents, enabling more precise and efficient modification of biomolecules. This period also witnessed the emergence of second-generation pegylation methods that allowed for site-specific attachment of PEG molecules, enhancing the control over drug properties such as pharmacokinetics and pharmacodynamics. In recent years, advancements in polymer chemistry and bioconjugation technologies have further refined pegylation processes. Modern approaches include the use of branched PEGs, which offer improved stability and reduced antigenicity compared to linear PEGs. Additionally, the development of novel conjugation chemistries has expanded the range of molecules that can be pegylated, including small molecules and nucleic acids. The impact of pegylation on drug development is multifaceted. By reducing renal clearance and minimizing immune responses, pegylated compounds often exhibit improved bioavailability and longer half-lives compared to their unmodified counterparts. This translates into fewer dosing requirements for patients, enhancing compliance and overall quality of life. Furthermore, pegylation has opened up new therapeutic avenues by enabling the delivery of previously challenging molecules. Today, pegylated compounds are integral to various treatment regimens across oncology, hematology, and infectious diseases among others. The ongoing research in this field continues to push boundaries, exploring new applications such as targeted drug delivery systems and combination therapies. As our understanding of pegylation deepens, it is clear that this technology will remain a cornerstone in the development of innovative therapeutics for years to come.
Types of Pegylation
Pegylation, a process of attaching polyethylene glycol (PEG) molecules to other molecules or surfaces, has evolved into various types to cater to diverse biomedical applications. This modification enhances the solubility, stability, and biocompatibility of therapeutic agents, while also reducing immunogenicity and improving pharmacokinetics. **Linear Pegylation**: This is the most common form where a single PEG chain is attached to the target molecule. Linear PEGs are versatile and can be easily conjugated to proteins, peptides, and small molecules. They are widely used in drug delivery systems due to their straightforward synthesis and well-characterized properties. **Branched Pegylation**: In this type, multiple PEG chains are attached to a central core, creating a branched structure. Branched PEGs offer higher molecular weight and greater hydrodynamic volume compared to linear PEGs of the same molecular weight, which can further prolong the circulation time of therapeutic agents in the bloodstream. **Dendritic Pegylation**: Dendrimers are highly branched, spherical molecules that can be pegylated to create dendritic PEGs. These structures have a high surface area-to-volume ratio, allowing for multiple attachments and enhanced drug loading capacity. Dendritic PEGs are particularly useful for targeted drug delivery due to their ability to encapsulate drugs within their core. **Comb-Shaped Pegylation**: This type involves attaching multiple linear PEG chains to a polymer backbone, resembling a comb-like structure. Comb-shaped PEGs combine the advantages of both linear and branched PEGs, offering improved solubility and stability while maintaining a relatively low molecular weight. **Y-Shaped Pegylation**: Y-shaped PEGs involve two PEG chains attached to a central core, forming a Y configuration. This design allows for balanced hydrophilicity and hydrophobicity, making it suitable for applications requiring specific interactions with biological surfaces. **Multi-Arm Pegylation**: Multi-arm PEGs feature several PEG chains radiating from a central point. These structures are highly effective in increasing the molecular weight of therapeutic proteins without significantly altering their native conformation, thereby extending their half-life in the body. Each type of pegylation offers unique advantages tailored to specific therapeutic needs. For instance, linear and branched PEGs are commonly used for protein modification to reduce immunogenicity and enhance circulation time. Dendritic and comb-shaped PEGs are more suited for drug encapsulation and targeted delivery due to their high surface area and drug-loading capacity. Understanding these different types of pegylation is crucial for developing effective pegylated compounds that can meet the demands of modern biomedicine. By selecting the appropriate type of pegylation, researchers can optimize the performance of therapeutic agents, leading to better patient outcomes and improved treatment efficacy.
Applications and Uses of Pegylated Compounds
Pegylated compounds, which involve the covalent attachment of polyethylene glycol (PEG) to molecules, have revolutionized various fields due to their enhanced solubility, stability, and reduced immunogenicity. These modifications have far-reaching implications across multiple disciplines. In the realm of **Pharmaceutical Applications**, pegylation significantly improves the pharmacokinetics and bioavailability of drugs, making them more effective and safer for patients. Beyond pharmaceuticals, **Biotechnology and Research** benefit from pegylation as it facilitates the development of novel diagnostic tools and therapeutic agents by enhancing their biocompatibility and longevity. Additionally, **Medical Therapies and Treatments** are advanced through pegylated compounds, which enable targeted drug delivery and prolonged therapeutic effects, thereby improving patient outcomes. This article delves into these critical applications, starting with a detailed exploration of how pegylation transforms the landscape of pharmaceuticals, enhancing drug efficacy and patient safety.
Pharmaceutical Applications
Pharmaceutical applications of pegylated compounds have revolutionized the field of drug delivery and therapeutic efficacy. Pegylation, the process of attaching polyethylene glycol (PEG) to a molecule, significantly enhances the pharmacokinetic and pharmacodynamic profiles of drugs. One of the primary advantages of pegylation is its ability to prolong the circulation time of therapeutic agents in the bloodstream, thereby reducing the frequency of dosing and improving patient compliance. For instance, pegylated interferons used in the treatment of hepatitis B and C exhibit extended half-lives compared to their non-pegylated counterparts, allowing for once-weekly dosing instead of daily injections. Moreover, pegylation can enhance the solubility of hydrophobic drugs, making them more suitable for intravenous administration. This is particularly beneficial for drugs like paclitaxel, which is used in cancer treatment but has poor aqueous solubility. Pegylation also reduces immunogenicity and antigenicity, minimizing the risk of adverse immune reactions. For example, pegylated liposomal doxorubicin (Doxil) is less cardiotoxic compared to conventional doxorubicin due to its reduced interaction with cardiac tissue. In addition to these benefits, pegylated compounds often exhibit improved stability and reduced clearance rates. This is crucial for biologics such as proteins and peptides that are prone to rapid degradation in the body. Pegvisomant, a pegylated growth hormone receptor antagonist used in the treatment of acromegaly, demonstrates enhanced stability and bioavailability compared to its unmodified form. The versatility of pegylation extends beyond small molecules and biologics; it also applies to nanoparticles and liposomes. Pegylated nanoparticles can evade the reticuloendothelial system (RES), allowing them to accumulate preferentially in tumor tissues due to the enhanced permeability and retention (EPR) effect. This targeted delivery enhances therapeutic efficacy while minimizing systemic toxicity. Furthermore, pegylation can be tailored to achieve specific release profiles through the use of different PEG molecular weights and conjugation strategies. This flexibility enables the development of personalized therapies that cater to individual patient needs. For example, pegylated enzymes such as pegvaliase (Palynziq) for phenylketonuria treatment can be engineered to provide sustained enzyme activity over extended periods. In conclusion, the pharmaceutical applications of pegylated compounds are diverse and transformative. By improving drug solubility, stability, and circulation time while reducing immunogenicity and toxicity, pegylation has become a cornerstone in modern drug development. As research continues to advance, it is likely that pegylated compounds will play an increasingly important role in addressing complex therapeutic challenges across various disease states.
Biotechnology and Research
Biotechnology and research play pivotal roles in the development and application of pegylated compounds, which are molecules that have been chemically modified by the covalent attachment of polyethylene glycol (PEG) polymer chains. This modification enhances the solubility, stability, and pharmacokinetics of therapeutic proteins, peptides, and small molecules, thereby improving their efficacy and reducing immunogenicity. The integration of biotechnology in this field involves advanced techniques such as genetic engineering, recombinant DNA technology, and protein engineering to design and produce pegylated compounds with specific properties. Research in biotechnology has led to the development of various pegylation methods, including random and site-specific pegylation, each with its own advantages and challenges. Random pegylation involves the attachment of PEG chains to multiple sites on a protein or molecule, which can sometimes lead to heterogeneous products. In contrast, site-specific pegylation targets specific amino acid residues, ensuring a more uniform product with predictable pharmacological properties. This precision is crucial for therapeutic applications where consistency is key. The applications of pegylated compounds are diverse and expanding rapidly. In the pharmaceutical industry, pegylation is used to extend the half-life of drugs, allowing for less frequent dosing and improved patient compliance. For instance, peginterferon alfa-2a, a pegylated form of interferon alpha, is used in the treatment of hepatitis B and C, offering a longer duration of action compared to its non-pegylated counterpart. Similarly, pegaspargase, a pegylated form of the enzyme L-asparaginase, is employed in the treatment of acute lymphoblastic leukemia, reducing the risk of allergic reactions. Beyond pharmaceuticals, pegylated compounds are also finding uses in diagnostics and drug delivery systems. In diagnostics, pegylated nanoparticles are being explored for their ability to enhance contrast in imaging techniques such as MRI and CT scans. These nanoparticles can be designed to target specific tissues or cells, providing more accurate and detailed images. In drug delivery, pegylated liposomes and micelles are being developed to improve the solubility and bioavailability of poorly soluble drugs, ensuring that therapeutic agents reach their target sites more effectively. Furthermore, ongoing research in biotechnology is pushing the boundaries of what is possible with pegylation. Advances in synthetic biology are enabling the design of novel PEG polymers with tailored properties, such as different molecular weights and branching patterns. These advancements are expected to further enhance the therapeutic potential of pegylated compounds by allowing for more precise control over their pharmacokinetic profiles. In conclusion, the synergy between biotechnology and research has been instrumental in the development and optimization of pegylated compounds. Continued innovation in this field promises to unlock new therapeutic possibilities, improve existing treatments, and expand the range of applications for these versatile molecules. As biotechnological tools continue to evolve, we can anticipate even more sophisticated and effective pegylated compounds that will transform healthcare and diagnostics in the years to come.
Medical Therapies and Treatments
Medical therapies and treatments have evolved significantly with the advent of pegylated compounds, which are molecules modified by the attachment of polyethylene glycol (PEG) chains. This modification enhances the pharmacokinetic and pharmacodynamic properties of therapeutic agents, making them more effective and safer for patients. Pegylation increases the hydrodynamic size of the molecule, reducing renal clearance and prolonging its circulation time in the bloodstream. This extended half-life allows for less frequent dosing, improving patient compliance and reducing side effects associated with peak drug concentrations. One of the most notable applications of pegylated compounds is in the treatment of chronic diseases such as hepatitis B and C. Pegylated interferons, for instance, have become a cornerstone in antiviral therapy due to their enhanced stability and bioavailability compared to their non-pegylated counterparts. These modified interferons exhibit improved antiviral activity while minimizing adverse effects like flu-like symptoms and hematologic abnormalities. In oncology, pegylated liposomal formulations of chemotherapeutic agents like doxorubicin have revolutionized cancer treatment by reducing cardiotoxicity and other systemic side effects. The liposomal encapsulation combined with pegylation ensures targeted delivery to tumor sites, thereby enhancing therapeutic efficacy while sparing healthy tissues. Moreover, pegylated proteins and enzymes are used to treat genetic disorders such as phenylketonuria (PKU) and severe combined immunodeficiency (SCID). For example, pegvaliase is a pegylated enzyme that helps manage PKU by breaking down phenylalanine, an amino acid that can accumulate to toxic levels in patients with this condition. Additionally, pegylated nanoparticles are being explored for targeted drug delivery in various medical conditions. These nanoparticles can be engineered to release therapeutic agents in response to specific stimuli at the disease site, ensuring localized treatment with minimal systemic exposure. The versatility of pegylation extends beyond pharmaceuticals; it also plays a crucial role in medical imaging and diagnostics. Pegylated contrast agents used in MRI scans improve image resolution by prolonging the circulation time of the contrast material, allowing for better visualization of tissues and organs. In summary, pegylated compounds have transformed medical therapies by enhancing drug stability, reducing toxicity, and improving therapeutic outcomes across a wide range of diseases. Their applications span from antiviral treatments to cancer therapy, genetic disorders, and advanced diagnostic tools, underscoring their significance in modern medicine. As research continues to advance, the potential uses of pegylated compounds are likely to expand further, offering new hope for patients suffering from diverse medical conditions.
Benefits and Challenges of Pegylation
Pegylation, the process of attaching polyethylene glycol (PEG) to molecules such as proteins, peptides, and small molecules, has revolutionized the field of pharmaceuticals by offering a multitude of benefits while also presenting several challenges. This technique enhances drug delivery and stability, allowing therapeutic agents to remain in the bloodstream longer and reach their targets more effectively. However, it also raises concerns about immunogenicity and toxicity, as the body may recognize PEGylated molecules as foreign and mount an immune response. Despite these challenges, ongoing research is paving the way for future directions and innovations in pegylation technology. By understanding these aspects, we can better harness the potential of pegylation to improve patient outcomes. In this article, we will delve into the enhanced drug delivery and stability provided by pegylation, explore the immunogenicity and toxicity concerns associated with it, and discuss the future directions and innovations that are shaping this field. First, let's examine how pegylation significantly enhances drug delivery and stability.
Enhanced Drug Delivery and Stability
Enhanced drug delivery and stability are pivotal aspects of modern pharmaceutical research, and one of the most effective strategies to achieve these goals is through pegylation. Pegylation involves the covalent attachment of polyethylene glycol (PEG) to a drug or therapeutic protein, which significantly alters its pharmacokinetic and pharmacodynamic properties. This modification enhances the drug's solubility, reduces immunogenicity, and prolongs its circulation time in the bloodstream. As a result, pegylated drugs exhibit improved bioavailability and reduced dosing frequency, making them more patient-friendly and potentially increasing treatment adherence. The enhanced stability of pegylated drugs is largely due to the hydrophilic nature of PEG, which creates a protective shield around the drug molecule. This shield prevents rapid clearance by the immune system and reduces enzymatic degradation, thereby extending the drug's half-life. For instance, pegylated interferons used in the treatment of hepatitis B and C have shown significantly longer half-lives compared to their non-pegylated counterparts, allowing for less frequent dosing and improved therapeutic outcomes. Moreover, pegylation can mask the antigenic sites on proteins, reducing the likelihood of an immune response. This is particularly beneficial for biologic drugs, which are often recognized as foreign by the immune system, leading to neutralizing antibodies that can diminish their efficacy. By masking these sites, pegylation helps in maintaining the therapeutic activity of the drug over a longer period. However, despite these benefits, pegylation also presents several challenges. One of the primary concerns is the potential for reduced activity due to steric hindrance. The large PEG molecules can interfere with the binding of the drug to its target, thereby reducing its therapeutic efficacy. Additionally, the process of pegylation itself can be complex and may require careful optimization to ensure that the desired pharmacological properties are retained. Another challenge is the heterogeneity of pegylated products. The attachment of PEG molecules can occur at multiple sites on a protein, leading to a mixture of different pegylated species. This heterogeneity can complicate the manufacturing process and make it difficult to achieve consistent product quality. Furthermore, regulatory approval for pegylated drugs can be more stringent due to the need for thorough characterization and validation of these complex molecules. In conclusion, while pegylation offers significant advantages in terms of drug delivery and stability, it also poses several challenges that must be carefully addressed. By understanding these benefits and challenges, researchers and manufacturers can better design and develop pegylated drugs that maximize therapeutic efficacy while minimizing potential drawbacks. This balanced approach is crucial for harnessing the full potential of pegylation in improving patient outcomes across a wide range of diseases.
Immunogenicity and Toxicity Concerns
Immunogenicity and toxicity concerns are critical aspects to consider when evaluating the benefits and challenges of pegylation, a process where polyethylene glycol (PEG) is covalently attached to molecules such as proteins, peptides, or small molecules. Pegylation enhances the pharmacokinetic properties of these molecules by increasing their solubility, reducing immunogenicity, and prolonging their circulation time in the body. However, despite these advantages, there are significant immunogenicity and toxicity concerns that must be addressed. One of the primary immunogenicity concerns is the potential for anti-PEG antibodies to form. These antibodies can neutralize the therapeutic effect of pegylated drugs and may lead to anaphylactic reactions in some cases. Studies have shown that pre-existing anti-PEG antibodies are present in a significant portion of the population, which could impact the efficacy and safety of pegylated therapies. Furthermore, repeated exposure to PEGylated products can induce an immune response, leading to the formation of new anti-PEG antibodies. This immunogenic response can be particularly problematic for patients requiring long-term treatment with pegylated drugs. Toxicity concerns also arise from the metabolic breakdown products of PEG. While PEG itself is generally considered biocompatible, its degradation products can accumulate in tissues over time, especially in patients with renal impairment. This accumulation may lead to adverse effects such as nephrotoxicity or other organ-specific toxicities. Additionally, the size and molecular weight of the PEG moiety can influence its biodistribution and clearance, with larger PEG molecules potentially causing more severe toxicity issues due to their slower clearance rates. Moreover, the conjugation process itself can introduce new toxicities. For instance, residual solvents or by-products from the pegylation reaction might remain in the final product, posing risks to patient safety. Ensuring rigorous purification and quality control measures during manufacturing is essential to mitigate these risks. Despite these challenges, researchers are actively exploring strategies to minimize immunogenicity and toxicity associated with pegylation. One approach involves using alternative polymers that may be less immunogenic than traditional PEG. Another strategy focuses on optimizing the design of pegylated conjugates to reduce the likelihood of anti-PEG antibody formation while maintaining therapeutic efficacy. In conclusion, while pegylation offers numerous benefits in terms of improving drug delivery and pharmacokinetics, it is crucial to carefully evaluate and manage the associated immunogenicity and toxicity concerns. By understanding these challenges and developing innovative solutions, scientists can continue to harness the potential of pegylation to create safer and more effective therapeutic agents. This balanced approach ensures that patients receive the maximum benefit from pegylated treatments while minimizing potential risks.
Future Directions and Innovations
As we delve into the future directions and innovations in pegylation, it becomes evident that this technology is poised for significant advancements. Pegylation, the process of attaching polyethylene glycol (PEG) molecules to proteins, drugs, or other therapeutic agents, has already shown considerable promise in enhancing the pharmacokinetics and reducing immunogenicity of various treatments. However, ongoing research is focused on overcoming current limitations and expanding its applications. One of the key future directions involves the development of more sophisticated PEGylation strategies. Researchers are exploring novel PEG architectures, such as branched or multi-arm PEGs, which can offer improved stability and longer circulation times compared to traditional linear PEGs. Additionally, site-specific PEGylation techniques are being refined to ensure that the attachment of PEG molecules does not interfere with the biological activity of the therapeutic agent. This precision engineering aims to maximize therapeutic efficacy while minimizing potential side effects. Another area of innovation is in the realm of targeted delivery systems. By conjugating PEG with targeting moieties such as antibodies, peptides, or small molecules, researchers aim to create therapeutics that can selectively bind to specific cells or tissues. This targeted approach could significantly enhance the therapeutic index by delivering drugs directly to the site of action, thereby reducing systemic exposure and associated adverse effects. The integration of pegylation with other emerging technologies, such as nanotechnology and gene therapy, is also a promising avenue. For instance, PEG-coated nanoparticles can be designed to encapsulate drugs or genetic material, providing a controlled release mechanism that prolongs therapeutic action and improves bioavailability. Similarly, pegylated gene vectors are being developed to enhance the stability and transfection efficiency of genetic material, potentially revolutionizing gene therapy. Furthermore, advancements in analytical techniques and computational modeling are crucial for optimizing pegylation processes. High-throughput screening methods and advanced spectroscopic tools allow for detailed characterization of pegylated products, ensuring consistency and quality. Computational models help predict the behavior of pegylated molecules in vivo, facilitating the design of optimal PEGylation strategies. In terms of clinical applications, future innovations will likely focus on expanding the range of diseases that can be treated using pegylated therapeutics. For example, pegylated enzymes are being explored for the treatment of genetic disorders, while pegylated cytokines and growth factors are under investigation for their potential in regenerative medicine. The use of pegylation in oncology is also gaining traction, with pegylated chemotherapeutics and immunotherapies showing promising results in preclinical studies. However, despite these exciting developments, there are challenges that need to be addressed. The cost of pegylation processes can be prohibitive, making it essential to develop more cost-effective methods. Additionally, regulatory frameworks need to evolve to accommodate the unique characteristics of pegylated products. Addressing these challenges will be crucial for translating these innovations into clinical practice. In conclusion, the future of pegylation is marked by significant potential for innovation and expansion. As researchers continue to push the boundaries of this technology, we can expect to see more efficient, targeted, and effective therapeutic agents that transform the landscape of healthcare. By addressing current challenges and leveraging emerging technologies, pegylation stands poised to play an increasingly important role in the development of novel treatments for a wide range of diseases.