What Is A Binary Compound
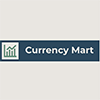
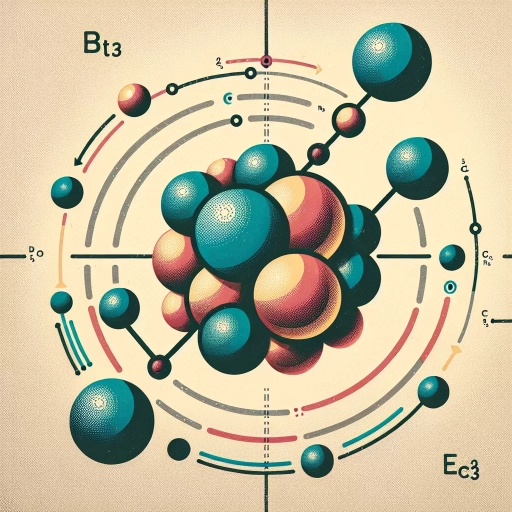
Binary compounds are fundamental in chemistry, consisting of two different elements chemically bonded together. These compounds play a crucial role in various fields, from industrial manufacturing to biological processes. To understand their significance, it is essential to delve into their definition and characteristics, which will be explored in the first section of this article. Additionally, we will examine how these compounds are formed and synthesized, highlighting the chemical reactions and processes involved. Finally, we will look at examples and applications of binary compounds, illustrating their importance in everyday life and advanced technologies. By grasping these aspects, readers will gain a comprehensive understanding of binary compounds. Let us begin by defining and exploring the characteristics of these essential chemical entities.
Definition and Characteristics of Binary Compounds
Binary compounds, composed of two distinct elements, are fundamental in chemistry due to their unique properties and widespread presence. Understanding these compounds involves delving into their chemical composition, molecular structure, and physical properties. The chemical composition of binary compounds is crucial as it defines the ratio and type of elements present, influencing the compound's overall characteristics. The molecular structure, which describes how these elements are arranged, plays a significant role in determining the compound's reactivity and stability. Additionally, the physical properties, such as melting point, solubility, and conductivity, provide valuable insights into the compound's behavior under various conditions. By examining these aspects, we can gain a comprehensive understanding of binary compounds. Let's begin by exploring the chemical composition of these compounds, which forms the foundation of their definition and characteristics.
Chemical Composition
Chemical composition is a fundamental concept in understanding binary compounds, which are chemical substances formed from two different elements. The composition of a binary compound is defined by the ratio of the atoms of each element present in the compound. This ratio is typically expressed using chemical formulas, where each element is represented by its symbol and the number of atoms of each element is indicated by a subscript number. For instance, in the binary compound sodium chloride (NaCl), the chemical formula indicates that one sodium atom is combined with one chlorine atom. The chemical composition of binary compounds can be determined through various analytical techniques such as mass spectrometry, atomic absorption spectroscopy, and X-ray fluorescence. These methods allow chemists to quantify the proportion of each element in the compound, ensuring that the composition adheres to the stoichiometric ratios defined by the chemical formula. Understanding the chemical composition is crucial for predicting the properties and behavior of binary compounds. For example, the composition influences the compound's melting and boiling points, solubility, and reactivity. In binary ionic compounds like NaCl, the electrostatic attraction between oppositely charged ions (sodium cations and chloride anions) dictates their physical properties and stability. Moreover, the chemical composition affects the compound's structure at both the molecular and crystal levels. In covalent binary compounds such as carbon dioxide (CO₂), the sharing of electron pairs between carbon and oxygen atoms results in a specific molecular geometry that influences its reactivity and environmental impact. In addition to these aspects, knowing the chemical composition helps in synthesizing binary compounds. Chemists must ensure that the reactants are mixed in the correct stoichiometric ratios to form the desired product efficiently. This precision is vital in industrial processes where binary compounds are produced on a large scale for various applications, including pharmaceuticals, electronics, and construction materials. Overall, understanding the chemical composition of binary compounds is essential for their identification, synthesis, and application across diverse fields. It provides a foundational framework for comprehending their properties and behaviors, thereby facilitating their use in science and technology.
Molecular Structure
Molecular structure is a fundamental concept in chemistry that describes the arrangement of atoms within a molecule. It is crucial for understanding the properties and behavior of binary compounds, which are chemical compounds composed of two different elements. The molecular structure of a binary compound can be visualized using various models such as the ball-and-stick model, space-filling model, and Lewis structure. In a binary compound, the molecular structure is determined by the type of chemical bond formed between the two elements. For instance, ionic bonds typically form between a metal and a nonmetal, resulting in compounds like sodium chloride (NaCl) where the sodium ion is electrostatically attracted to the chloride ion. Covalent bonds, on the other hand, form between two nonmetals and involve the sharing of electron pairs, as seen in molecules like hydrogen chloride (HCl) where hydrogen and chlorine share electrons to achieve a stable electronic configuration. The geometry of the molecular structure is also significant. It can be predicted using VSEPR (Valence Shell Electron Pair Repulsion) theory, which states that electron pairs around a central atom will arrange themselves to minimize repulsion. For example, in carbon dioxide (CO₂), the central carbon atom forms double bonds with two oxygen atoms, leading to a linear molecular geometry due to the symmetrical arrangement of electron pairs. Understanding the molecular structure of binary compounds is essential for predicting their physical and chemical properties. For example, the polarity of a molecule, which arises from the unequal sharing of electrons in covalent bonds, influences its solubility and boiling point. Polar molecules like water (H₂O) are more soluble in water than nonpolar molecules like carbon tetrachloride (CCl₄). Moreover, the molecular structure affects the reactivity of binary compounds. The presence of specific functional groups or bonds can make a compound more reactive under certain conditions. For instance, hydrogen fluoride (HF) is highly reactive due to its polar covalent bond, which makes it a strong acid. In conclusion, the molecular structure of binary compounds provides critical insights into their composition, properties, and reactivity. By analyzing these structures through various models and theories, chemists can better understand how these compounds interact with other substances and predict their behavior under different conditions. This knowledge is indispensable in fields such as materials science, pharmaceuticals, and environmental chemistry where binary compounds play pivotal roles.
Physical Properties
Physical properties of binary compounds are intrinsic characteristics that can be observed or measured without altering the chemical identity of the substance. These properties include melting and boiling points, density, solubility, and electrical conductivity. For instance, the melting and boiling points of binary compounds are typically higher than those of their constituent elements due to the stronger intermolecular forces between the atoms. Density is another crucial physical property, often determined by the atomic masses and the packing efficiency of the atoms in the crystal lattice. Solubility varies widely among binary compounds; some are highly soluble in water or other solvents, while others are insoluble. Electrical conductivity is also a significant property, with some binary compounds exhibiting high conductivity due to the presence of free electrons, such as in metal-metal binary compounds, while others may be insulators or semiconductors. Understanding these physical properties is essential for predicting the behavior and potential applications of binary compounds in various fields, including chemistry, materials science, and engineering. For example, silicon carbide (SiC), a binary compound of silicon and carbon, has a high melting point and excellent hardness, making it suitable for use in high-temperature applications and abrasive materials. Similarly, gallium arsenide (GaAs), a semiconductor binary compound, is widely used in electronic devices due to its unique electrical properties. These examples illustrate how the physical properties of binary compounds can dictate their utility and performance in diverse technological contexts.
Formation and Synthesis of Binary Compounds
The formation and synthesis of binary compounds are fundamental processes in chemistry, involving the combination of two elements to form a new compound. Understanding these processes is crucial for various fields, including materials science, pharmaceuticals, and industrial chemistry. This article delves into the intricacies of binary compound formation, focusing on three key aspects: the chemical reactions involved, the methods of synthesis, and the conditions necessary for their formation. By examining the chemical reactions involved, we can grasp the underlying mechanisms that drive the formation of these compounds. This understanding is essential as it lays the groundwork for exploring different methods of synthesis, which can vary significantly depending on the elements and desired outcomes. Additionally, recognizing the specific conditions required for the formation of binary compounds is vital for optimizing their production. In this article, we will first explore the chemical reactions involved in the formation of binary compounds, setting the stage for a deeper analysis of synthesis methods and formation conditions.
Chemical Reactions Involved
Chemical reactions play a pivotal role in the formation and synthesis of binary compounds, which are composed of two different elements. These reactions involve the transformation of reactants into products through various mechanisms, often driven by changes in chemical bonds. One common type of reaction is the synthesis reaction, where two elements combine to form a new compound. For example, when hydrogen gas (H₂) reacts with oxygen gas (O₂), they form water (H₂O) through a highly exothermic reaction: 2H₂ + O₂ → 2H₂O. This process illustrates how binary compounds can be synthesized from their constituent elements. Another significant type of reaction is the single displacement reaction, where one element displaces another from a compound. For instance, when zinc metal (Zn) reacts with hydrochloric acid (HCl), zinc chloride (ZnCl₂) and hydrogen gas are produced: Zn + 2HCl → ZnCl₂ + H₂. This reaction demonstrates how binary compounds like zinc chloride can be formed through the displacement of hydrogen by zinc. Combustion reactions are also crucial in forming binary compounds, particularly oxides. When magnesium metal (Mg) burns in oxygen, it forms magnesium oxide (MgO): 2Mg + O₂ → 2MgO. This reaction highlights the synthesis of a binary oxide through combustion. In addition to these types, acid-base reactions can lead to the formation of binary salts. For example, when sodium hydroxide (NaOH) reacts with hydrochloric acid (HCl), sodium chloride (NaCl) and water are formed: NaOH + HCl → NaCl + H₂O. This reaction shows how binary salts like sodium chloride can be synthesized from an acid-base neutralization. The formation of binary compounds often involves redox reactions, where one element is oxidized while another is reduced. The reaction between aluminum metal (Al) and chlorine gas (Cl₂) to form aluminum chloride (AlCl₃) is a classic example: 2Al + 3Cl₂ → 2AlCl₃. Here, aluminum is oxidized, and chlorine is reduced, resulting in the formation of a binary halide. Understanding these chemical reactions is essential for comprehending the principles behind the formation and synthesis of binary compounds. Each type of reaction provides insights into how different elements interact and combine to form new substances with unique properties. By mastering these concepts, chemists can design and execute various synthetic routes to produce a wide range of binary compounds with specific applications in fields such as materials science, pharmaceuticals, and energy storage.
Methods of Synthesis
The synthesis of binary compounds, which are composed of two different elements, can be achieved through several methods, each tailored to the specific properties and reactivity of the elements involved. One common method is the **direct combination** of elements, where the reactants are heated or subjected to high pressure to facilitate their reaction. For example, the synthesis of sodium chloride (NaCl) from sodium (Na) and chlorine (Cl₂) involves heating sodium metal in a stream of chlorine gas, resulting in the formation of NaCl. Another method is **single displacement reactions**, where one element displaces another from a compound. For instance, when zinc (Zn) reacts with copper(II) sulfate (CuSO₄), zinc displaces copper to form zinc sulfate (ZnSO₄) and copper metal. **Hydrothermal synthesis** is another technique used for synthesizing binary compounds, particularly those that are difficult to obtain through traditional methods. This involves heating the reactants in a sealed vessel filled with water or another solvent under high pressure and temperature, allowing for the formation of crystalline materials. This method is often used for synthesizing metal oxides and sulfides. **Sol-gel processing** is a versatile method that involves the hydrolysis and condensation of metal alkoxides to form a gel-like substance, which is then dried and calcined to produce the desired binary compound. This technique is particularly useful for synthesizing metal oxides with controlled particle size and morphology. **Electrochemical synthesis** can also be employed, where an electric current is used to drive the reaction between two elements. For example, the electrolysis of molten sodium chloride produces sodium metal at the cathode and chlorine gas at the anode. This method is highly effective for producing pure metals and their compounds. In addition, **chemical vapor deposition (CVD)** is a technique where volatile precursors are decomposed or reacted to deposit the desired binary compound on a substrate. This method is widely used in semiconductor manufacturing for producing thin films of materials like silicon carbide (SiC) and titanium nitride (TiN). Lastly, **mechanochemical synthesis** involves the use of mechanical energy, such as grinding or milling, to induce chemical reactions between solid reactants. This method is particularly useful for synthesizing compounds that are difficult to obtain through other means due to their high reactivity or stability issues. Each of these methods offers unique advantages and is selected based on the specific requirements of the synthesis, including the properties of the elements involved, the desired purity of the product, and the scalability of the process. Understanding these various synthesis techniques is crucial for the formation and study of binary compounds, enabling researchers to tailor their approaches to achieve high-quality materials with precise properties.
Conditions for Formation
The formation of binary compounds, which are chemical compounds composed of two different elements, is governed by specific conditions that ensure the stability and feasibility of the resulting compound. One primary condition is the difference in electronegativity between the two elements involved. Typically, binary compounds form when one element is a metal and the other is a nonmetal, with the metal having a lower electronegativity value compared to the nonmetal. This difference allows for the transfer of electrons from the metal to the nonmetal, resulting in the formation of ions with opposite charges that attract each other to form a stable ionic bond. Another crucial condition is the ability of the elements to achieve a stable electronic configuration. According to the octet rule, atoms tend to gain, lose, or share electrons to achieve a full outer energy level, similar to that of the noble gases. For instance, when sodium (Na) reacts with chlorine (Cl), sodium loses one electron to form Na+ and chlorine gains one electron to form Cl-, both achieving a stable octet configuration. This process leads to the formation of sodium chloride (NaCl), a common binary compound. The reactivity of the elements also plays a significant role. Highly reactive metals like sodium and potassium readily form binary compounds with highly reactive nonmetals such as fluorine and chlorine. Conversely, less reactive metals may require specific conditions or catalysts to form binary compounds with less reactive nonmetals. Additionally, the formation of binary compounds can be influenced by thermodynamic factors such as temperature and pressure. Some binary compounds may only form under specific thermal or pressure conditions. For example, the synthesis of certain metal oxides requires high temperatures to overcome activation energy barriers and facilitate the reaction. Finally, the presence of catalysts or other reactants can also affect the formation of binary compounds. Catalysts can lower the activation energy required for a reaction, making it more feasible under standard conditions. For instance, in the Haber-Bosch process for ammonia synthesis, iron acts as a catalyst to facilitate the reaction between nitrogen and hydrogen gases. In summary, the formation of binary compounds is contingent upon several key conditions including electronegativity differences, the ability to achieve stable electronic configurations, reactivity of the elements, thermodynamic conditions, and sometimes the presence of catalysts. These factors collectively determine whether and how two elements will combine to form a stable binary compound. Understanding these conditions is essential for predicting and synthesizing binary compounds in various chemical reactions.
Examples and Applications of Binary Compounds
Binary compounds, composed of two distinct elements, play a pivotal role in various aspects of our lives. From the mundane to the industrial and biological, these compounds are ubiquitous and essential. In everyday life, common binary compounds such as water (H₂O), carbon dioxide (CO₂), and ammonia (NH₃) are integral to our daily routines and environmental balance. Moving beyond the domestic sphere, industrial uses of binary compounds are vast and critical, with applications in manufacturing, energy production, and advanced materials. For instance, silicon carbide (SiC) is used in high-performance ceramics and semiconductors. Additionally, the biological importance of binary compounds cannot be overstated; they form the backbone of many biological processes and molecules, such as oxygen (O₂) and hydrogen peroxide (H₂O₂), which are crucial for cellular respiration and defense mechanisms. This article will delve into these three key areas, starting with an exploration of common binary compounds in everyday life, highlighting their omnipresence and significance in our daily lives.
Common Binary Compounds in Everyday Life
Binary compounds, which consist of two different elements, are ubiquitous in everyday life, playing crucial roles in various applications. One of the most common binary compounds is water (H₂O), essential for human survival and a cornerstone of biological processes. Another example is carbon dioxide (CO₂), a vital component of the Earth's atmosphere and a key player in photosynthesis and respiration. In the realm of technology, silicon dioxide (SiO₂), or silica, is a fundamental material in the production of glass and semiconductors, which are integral to modern electronics. Sodium chloride (NaCl), or table salt, is not only a seasoning but also crucial in preserving food and maintaining bodily functions. Hydrogen peroxide (H₂O₂) is widely used as a disinfectant and bleach, while ammonia (NH₃) serves as a precursor to fertilizers and cleaning agents. Additionally, sulfuric acid (H₂SO₄) is a pivotal chemical in the manufacturing of batteries, detergents, and pharmaceuticals. These binary compounds illustrate how simple combinations of elements can yield substances with diverse and essential applications, underscoring their importance in both natural and industrial contexts. Their versatility and widespread use highlight the significance of binary compounds in enhancing our daily lives and driving technological advancements.
Industrial Uses of Binary Compounds
Binary compounds, consisting of two elements, play a crucial role in various industrial applications due to their unique properties and versatility. One of the most significant industrial uses is in the production of semiconductors, where binary compounds like silicon carbide (SiC) and gallium arsenide (GaAs) are essential for manufacturing electronic devices. Silicon carbide, for instance, is used in high-power electronics and solar cells due to its high thermal conductivity and resistance to radiation. Gallium arsenide, on the other hand, is preferred for high-frequency applications such as microwave devices and solar panels because of its superior electron mobility compared to silicon. In the chemical industry, binary compounds are vital as catalysts and reagents. For example, aluminum chloride (AlCl₃) is a key catalyst in the Friedel-Crafts alkylation and acylation reactions, which are crucial for producing various organic compounds used in pharmaceuticals, dyes, and plastics. Another important binary compound is titanium dioxide (TiO₂), widely used as a pigment in paints, coatings, and plastics due to its bright white color and high opacity. The energy sector also relies heavily on binary compounds. Lithium cobalt oxide (LiCoO₂), a binary compound of lithium and cobalt, is a primary component in lithium-ion battery cathodes, enabling efficient energy storage for electric vehicles and portable electronics. Similarly, zinc sulfide (ZnS) is used in phosphor coatings for fluorescent lamps and LEDs, enhancing their luminosity and efficiency. In materials science, binary compounds are used to create advanced materials with specific properties. Boron carbide (B₄C), for example, is one of the hardest substances known and is used in body armor and ballistic shields due to its exceptional hardness and light weight. Tungsten carbide (WC), another binary compound, is used in cutting tools and wear-resistant parts because of its high hardness and resistance to abrasion. Furthermore, binary compounds are integral in environmental technologies. For instance, titanium dioxide is also used in photocatalytic applications for water purification and air cleaning due to its ability to decompose organic pollutants when exposed to light. Additionally, zinc oxide (ZnO) is used in sunscreen formulations and as an antimicrobial agent in various consumer products. In conclusion, the industrial uses of binary compounds are diverse and critical across multiple sectors. Their unique chemical and physical properties make them indispensable for a wide range of applications, from electronics and energy storage to materials science and environmental technologies. Understanding these compounds is essential for advancing technological innovation and meeting the demands of modern industries.
Biological Importance of Binary Compounds
Binary compounds, consisting of two different elements, play a crucial role in various biological processes and systems. One of the most significant biological importance of binary compounds is their presence in essential biomolecules. For instance, water (H₂O), a binary compound of hydrogen and oxygen, is the medium in which all cellular reactions occur and is vital for maintaining cellular structure and function. Another example is carbon dioxide (CO₂), a binary compound of carbon and oxygen, which is crucial for photosynthesis in plants and respiration in animals. In the human body, binary compounds such as sodium chloride (NaCl) or common salt are essential for maintaining fluid balance and nerve function. Potassium chloride (KCl) is another binary compound that helps regulate heart function and muscle contractions. The binary compound hydrogen peroxide (H₂O₂) acts as an antimicrobial agent within the body, helping to protect against infections. Furthermore, binary compounds are integral to the structure and function of biomolecules like nucleic acids and proteins. Phosphorus pentoxide (P₂O₅), when combined with other elements, forms phosphate groups that are fundamental components of DNA and RNA, enabling genetic information storage and transmission. Similarly, sulfur-containing binary compounds like hydrogen sulfide (H₂S) are involved in various metabolic pathways and have been implicated in signaling processes within cells. In addition to their roles within organisms, binary compounds also influence ecosystems. For example, nitrogen oxides (NOx), such as nitric oxide (NO) and nitrogen dioxide (NO₂), are involved in atmospheric chemistry and can affect air quality and plant growth. The binary compound silicon dioxide (SiO₂), or silica, is a major component of soil and plays a critical role in plant nutrition. The applications of binary compounds extend beyond biological systems to medical treatments and agricultural practices. For instance, oxygen (O₂) therapy is used to treat respiratory conditions by increasing oxygen levels in the blood. In agriculture, ammonium nitrate (NH₄NO₃) serves as a fertilizer to enhance crop yields by providing essential nitrogen for plant growth. In conclusion, the biological importance of binary compounds is multifaceted and pervasive. From their roles in fundamental cellular processes to their applications in medicine and agriculture, these compounds are indispensable for life as we know it. Their unique properties make them essential for maintaining health, supporting ecosystems, and driving various biological functions that underpin life on Earth.