What Is External Force
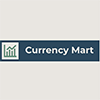
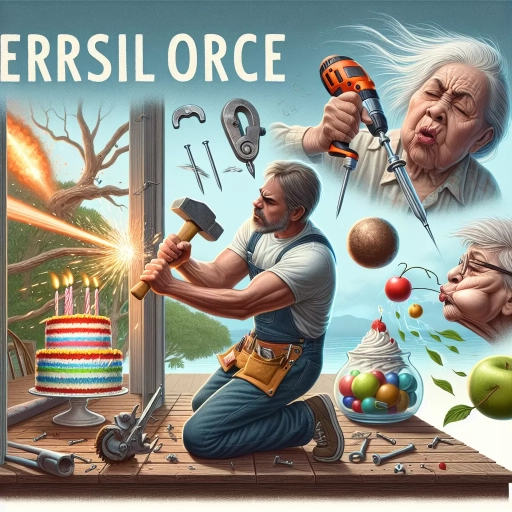
Definition and Concept of External Force
Understanding the concept of external force is crucial in the realm of physics, as it underpins our comprehension of how objects interact with their environment. An external force is a push or pull that originates from outside an object, influencing its motion or shape. This article delves into the multifaceted nature of external forces, providing a comprehensive overview through three key perspectives. First, we will explore the basic definition and examples of external forces, illustrating how they manifest in everyday life and scientific contexts. Next, we will distinguish between internal and external forces, clarifying the often-blurred lines between these two types of forces. Finally, we will examine the historical development of the concept, tracing how our understanding of external forces has evolved over time. By examining these aspects, readers will gain a robust understanding of what constitutes an external force and its significance in physical phenomena. Let us begin by defining and exemplifying external forces, laying the groundwork for a deeper exploration of this fundamental concept.
1. Basic Definition and Examples
**Basic Definition and Examples** An external force, a fundamental concept in physics, is any force that acts on an object from outside its boundaries. This distinction is crucial because it contrasts with internal forces, which arise from interactions within the system itself. To grasp the concept of external forces, it's essential to understand their role in altering the motion or state of an object. At its core, an external force is a push or pull that originates from outside the system being studied. For instance, when you push a box across the floor, the force exerted by your hand is an external force acting on the box. Similarly, gravity acting on an object is an external force because it originates from the Earth's mass rather than from within the object itself. Another example is friction; when a car brakes, the frictional force between the tires and the road is an external force that slows down the vehicle. In everyday life, external forces are ubiquitous. When you lift a book off a shelf, the upward force you apply is external to the book. The wind blowing against a tree or a building also constitutes an external force. Even the force exerted by a spring when it is compressed or stretched is considered external if it acts on an object outside of itself. Understanding external forces is vital in various fields such as engineering, where designers must account for external loads like wind resistance or gravitational pull when constructing buildings or bridges. In sports, athletes often manipulate external forces to achieve desired outcomes; for example, a golfer uses an external force (the swing of the club) to propel the ball into flight. The impact of external forces can be seen in Newton's laws of motion. According to Newton's first law (the law of inertia), an object will remain in its state unless acted upon by an external force. This principle underscores the importance of recognizing and quantifying these forces to predict and analyze motion accurately. In summary, external forces are pivotal in understanding how objects interact with their environment and how their motion can be altered. By identifying and analyzing these forces, we can better comprehend natural phenomena and design systems that operate efficiently and safely in response to various external influences. This foundational knowledge is essential for advancing our understanding of the physical world and for making practical applications in science and engineering.
2. Distinguishing Internal vs. External Forces
When delving into the realm of physics, understanding the distinction between internal and external forces is crucial for accurately analyzing and predicting the behavior of physical systems. Internal forces are those that arise from interactions within a system itself, such as the gravitational attraction between two planets in a solar system or the tension in a rope connecting two objects. These forces do not alter the overall momentum of the system because they act in pairs, as described by Newton's third law of motion. For instance, in a closed system like a car with passengers, the forces exerted by the passengers on each other and on the car are internal; they do not change the total momentum of the car-passenger system. On the other hand, external forces are those that act on a system from outside its boundaries. These forces can significantly impact the system's motion and momentum. Examples include frictional forces from the ground, gravitational forces from Earth, and thrust from an engine. Unlike internal forces, external forces can cause changes in the system's overall momentum because they are not balanced by equal and opposite forces within the system. For example, when a car accelerates down a road, the thrust provided by its engine is an external force that increases its momentum, while friction from the road surface is another external force that opposes this motion. Understanding this distinction is vital in various fields such as engineering, where designing structures and machines requires careful consideration of both internal stresses and external loads. In biomechanics, knowing whether forces acting on the human body are internal (like muscle contractions) or external (like ground reaction forces during walking) helps in analyzing movement patterns and preventing injuries. Furthermore, in cosmology, distinguishing between internal gravitational forces within galaxies and external gravitational influences from neighboring galaxies aids in understanding galaxy dynamics. In practical terms, recognizing whether a force is internal or external helps in applying conservation laws effectively. For instance, the law of conservation of momentum applies to closed systems where only internal forces are present; however, if external forces are involved, this law must be adjusted accordingly to account for changes in momentum. This nuanced understanding enhances our ability to model real-world phenomena accurately and make precise predictions about how systems will behave under different conditions. In conclusion, the differentiation between internal and external forces is a fundamental concept in physics that underpins our comprehension of how physical systems interact with their environment. By identifying whether forces are originating from within or outside a system, we can better analyze dynamics, apply conservation laws, and predict outcomes with greater precision. This clarity is essential not only for theoretical physics but also for practical applications across various disciplines.
3. Historical Development of the Concept
The concept of external force has a rich and evolving history that spans centuries, reflecting the gradual refinement of our understanding of the physical world. The earliest recorded discussions on forces date back to ancient Greece, where philosophers such as Aristotle and Archimedes laid foundational ideas. Aristotle, in his work "Physica," introduced the concept of "natural motion" and "violent motion," which can be seen as precursors to the modern distinction between internal and external forces. Archimedes, known for his contributions to mechanics, particularly in the field of statics, provided insights into the balance of forces that would later influence the development of modern physics. The Renaissance period saw significant advancements with the work of Galileo Galilei and Johannes Kepler. Galileo's experiments with falling bodies and his formulation of the law of inertia marked a crucial shift towards understanding how external forces act on objects. Kepler's laws of planetary motion further elucidated how gravitational forces, an archetypal example of external forces, govern celestial mechanics. However, it was Sir Isaac Newton who synthesized these insights into his groundbreaking "Philosophiæ Naturalis Principia Mathematica" in 1687. Newton's three laws of motion and the law of universal gravitation provided a comprehensive framework for understanding external forces as agents that cause changes in the motion of objects. In the 19th century, the work of scientists like Michael Faraday and James Clerk Maxwell expanded our understanding of external forces beyond mechanical interactions. Faraday's discovery of electromagnetic induction and Maxwell's formulation of the electromagnetic field equations introduced new types of external forces—electromagnetic forces—that act on charged particles. This period also saw the development of thermodynamics, where external forces were considered in the context of heat transfer and energy exchange. The 20th century brought about revolutionary changes with the advent of quantum mechanics and relativity. Albert Einstein's theory of general relativity redefined our understanding of gravity as a curvature of spacetime caused by mass and energy, rather than a force acting across space. Quantum mechanics, developed by figures such as Niels Bohr and Erwin Schrödinger, introduced wave-particle duality and the concept of quantum fields, which further refined our understanding of how external forces interact at the microscopic level. Today, the concept of external force is integral to various fields including physics, engineering, and materials science. Modern research continues to explore new dimensions of external forces, such as those involved in nanotechnology and advanced materials science. The historical development of this concept underscores the cumulative nature of scientific knowledge, where each era builds upon the discoveries of the past to provide a more nuanced and accurate understanding of how external forces shape our physical world. This evolution not only reflects our growing sophistication in describing natural phenomena but also highlights the enduring importance of understanding external forces in advancing technological innovation and scientific inquiry.
Types and Examples of External Forces
External forces are fundamental in understanding the dynamics of physical systems, influencing the motion and behavior of objects in various environments. These forces can be categorized into several types, each with distinct characteristics and effects. This article delves into three primary types of external forces: gravitational forces, frictional forces, and applied forces along with normal forces. Gravitational forces, the universal attraction between masses, shape the large-scale structure of the universe and govern the motion of celestial bodies. Frictional forces, arising from surface interactions, play a crucial role in everyday life by enabling traction and preventing slipping. Applied forces and normal forces, which include any force exerted by an external agent and the force exerted by a surface on an object, respectively, are essential in understanding mechanical interactions and structural integrity. By exploring these categories, we gain a comprehensive understanding of how external forces interact with objects to produce motion, deformation, or equilibrium. Let us begin by examining the first of these: gravitational forces.
1. Gravitational Forces
Gravitational forces are one of the most fundamental and pervasive types of external forces in the universe. These forces arise from the interaction between masses, where every point mass attracts every other point mass by a force acting along the line intersecting both points. This concept, first described by Sir Isaac Newton in his groundbreaking work "Philosophiæ Naturalis Principia Mathematica," is encapsulated in Newton's Law of Universal Gravitation. According to this law, the gravitational force between two objects is directly proportional to the product of their masses and inversely proportional to the square of the distance between their centers. Gravitational forces play a crucial role in shaping our everyday experiences and the structure of the cosmos. On Earth, gravity pulls objects towards its center, giving them weight and keeping us firmly on the ground. It is also responsible for the tides, as the gravitational pull of the Moon and Sun on Earth's oceans causes water levels to rise and fall. In celestial mechanics, gravity governs the orbits of planets around stars, moons around planets, and even galaxies within galaxy clusters. The gravitational attraction between stars and gas can lead to the formation of new stars and planetary systems. One of the most striking examples of gravitational forces is seen in black holes—regions of space where the gravitational pull is so strong that nothing, including light, can escape once it falls within a certain boundary known as the event horizon. Another example is gravitational waves, ripples in spacetime predicted by Albert Einstein's theory of General Relativity and later detected directly by the Laser Interferometer Gravitational-Wave Observatory (LIGO). These waves are produced by violent cosmic events such as the collision of two black holes or neutron stars. In addition to these phenomena, gravitational forces have practical applications in various fields. For instance, understanding gravity is essential for space exploration; it helps scientists and engineers design trajectories for spacecraft and predict their orbits around celestial bodies. In geophysics, studying gravitational fields helps in mapping subsurface structures and locating mineral deposits. Despite its ubiquity and importance, gravity remains one of the least understood forces compared to other fundamental forces like electromagnetism and the strong and weak nuclear forces. Researchers continue to explore its mysteries through ongoing studies in cosmology, particle physics, and theoretical physics. For example, theories such as Modified Newtonian Dynamics (MOND) and TeVeS attempt to explain observed phenomena without invoking dark matter—a hypothetical form of matter thought to influence galaxy rotation curves through gravitational effects. In conclusion, gravitational forces are a cornerstone of our understanding of the physical world. They influence everything from falling objects on Earth to the vast expanses of interstellar space. As scientists continue to unravel the intricacies of gravity through both theoretical frameworks and empirical observations, we gain deeper insights into how this fundamental force shapes our universe at all scales.
2. Frictional Forces
Frictional forces are a fundamental type of external force that play a crucial role in our daily lives, influencing how objects interact and move relative to each other. These forces arise from the interaction between two surfaces that are in contact, and they act in the opposite direction of the intended or actual motion. There are several types of frictional forces, each with distinct characteristics and applications. **Static Friction** is the force that prevents an object from moving when a force is applied to it. It is the maximum force that must be overcome for an object to start moving. For instance, when you try to push a heavy box across the floor, static friction must be overcome before the box begins to move. The coefficient of static friction depends on the nature of the surfaces in contact; for example, rubber on concrete has a higher coefficient than ice on ice. **Kinetic Friction**, also known as sliding friction, occurs when an object is already moving and acts to slow it down. This force is generally weaker than static friction but still significant. When you slide a book across a table, kinetic friction is at work, gradually reducing the book's speed until it comes to a stop. **Rolling Friction** is another type of frictional force that occurs when an object rolls over a surface. This type of friction is typically weaker than both static and kinetic friction because rolling involves less surface contact and thus less resistance. For example, a wheel rolling on pavement experiences rolling friction, which is why it can travel further with less effort compared to sliding. **Fluid Friction** or **Viscous Drag** occurs in fluids such as air or water and opposes the motion of objects through these mediums. The resistance depends on the viscosity of the fluid and the shape and speed of the moving object. For instance, an airplane experiences fluid friction as it moves through the air, which affects its speed and fuel efficiency. Understanding frictional forces is essential for various engineering applications. In automotive design, engineers optimize tire tread patterns to maximize static friction for better grip on roads while minimizing rolling friction for improved fuel efficiency. In aerospace engineering, reducing fluid friction is critical for achieving higher speeds and better aerodynamics. In addition to their practical applications, frictional forces also have significant implications in everyday activities. For example, without sufficient static friction between your feet and the ground, walking would be impossible; similarly, kinetic friction helps in stopping vehicles safely by converting kinetic energy into heat energy. In conclusion, frictional forces are vital components of external forces that influence how objects move and interact with their surroundings. By understanding these different types—static, kinetic, rolling, and fluid friction—we can better design systems that either harness or mitigate these forces to achieve desired outcomes in various fields of science and engineering.
3. Applied Forces and Normal Forces
When discussing external forces, it is crucial to delve into the specifics of applied forces and normal forces, as these are fundamental components that shape our understanding of how objects interact with their environment. **Applied forces** are those that are intentionally exerted on an object by an external agent. For instance, when you push a door open or pull a rope to lift a load, you are applying a force. These forces can be either static or dynamic; static forces do not cause motion but can change the shape of an object (like compressing a spring), while dynamic forces result in acceleration and movement (such as pushing a car forward). The direction and magnitude of applied forces are critical in determining the resulting motion or deformation of the object. On the other hand, **normal forces** are a type of contact force that arises from the interaction between two surfaces in contact. This force acts perpendicular to the surface of contact and is often denoted as \( F_N \). A classic example is the force exerted by the ground on your feet when you stand; this normal force counteracts your weight, preventing you from sinking into the ground. Normal forces play a pivotal role in understanding frictional forces since frictional forces act parallel to the surface and are directly influenced by the magnitude of the normal force. For example, if you increase the normal force by pressing harder on a surface, the frictional force also increases, making it more difficult to slide an object across that surface. The interplay between applied and normal forces is essential in various real-world scenarios. In engineering, understanding these forces helps in designing structures that can withstand external loads without collapsing. For instance, architects must consider both the applied forces (like wind and weight) and normal forces (such as those exerted by foundations) to ensure that buildings remain stable. In physics experiments, accurately measuring applied and normal forces is crucial for validating theoretical models and predicting outcomes. Moreover, these concepts extend beyond static situations to dynamic ones. In sports, athletes leverage applied forces to achieve high performance; for example, a sprinter applies significant force to the ground to generate speed, while a gymnast relies on precise control over normal forces during landings to avoid injury. The balance between these forces is what distinguishes a successful performance from an unsuccessful one. In conclusion, applied and normal forces are integral to comprehending how external forces influence objects in various contexts. By grasping these fundamental concepts, we can better analyze and predict the behavior of objects under different conditions, whether in everyday life or in complex scientific and engineering applications. This understanding not only enhances our ability to solve problems but also fosters innovation and safety across multiple disciplines.
Impact and Applications of External Forces
The impact and applications of external forces are fundamental concepts in physics, influencing various aspects of our daily lives and technological advancements. External forces, whether they be frictional, gravitational, or applied, play a crucial role in determining the motion and acceleration of objects. This article delves into three key areas to illustrate the significance of external forces: motion and acceleration, energy transfer and work done, and real-world applications in engineering and physics. By understanding how external forces affect motion and acceleration, we can predict and control the behavior of objects under different conditions. Additionally, recognizing how these forces facilitate energy transfer and the work done on an object is essential for optimizing mechanical systems. Finally, exploring real-world applications in engineering and physics highlights the practical implications of these principles, from designing safer vehicles to constructing more efficient machinery. This comprehensive approach will provide a thorough insight into the multifaceted role of external forces, beginning with the foundational concept of motion and acceleration.
1. Motion and Acceleration
Motion and acceleration are fundamental concepts in understanding the impact and applications of external forces. Motion, in its simplest form, refers to the change in position of an object with respect to time. However, when an external force is applied, it can alter this motion by causing acceleration. Acceleration is the rate of change of velocity and is a direct result of the force applied, as described by Newton's second law of motion: \(F = ma\), where \(F\) is the net force acting on an object, \(m\) is its mass, and \(a\) is the resulting acceleration. The relationship between motion and acceleration highlights the critical role of external forces. For instance, in a car accelerating from a standstill, the force exerted by the engine propels the vehicle forward, increasing its velocity over time. This acceleration is not just a theoretical concept but has practical applications in various fields. In engineering, understanding motion and acceleration is crucial for designing safe and efficient transportation systems, such as high-speed trains and aircraft. The precise calculation of forces and resulting accelerations ensures that these vehicles can operate within safe limits while achieving desired performance metrics. Moreover, the study of motion and acceleration underlies many technological advancements. In robotics, for example, external forces are carefully controlled to achieve precise movements and tasks. Industrial robots use actuators that apply specific forces to move limbs or manipulate objects with high accuracy, leveraging the principles of motion and acceleration to perform complex tasks efficiently. In sports science, understanding how external forces affect motion is vital for optimizing athletic performance. Coaches and trainers analyze the forces applied during different movements to enhance speed, agility, and strength. For instance, in sprinting, the force exerted by the ground on the athlete's feet determines their acceleration off the starting line. By optimizing this force through proper technique and training, athletes can achieve faster times. Additionally, in medical fields like physical therapy and rehabilitation, understanding motion and acceleration helps in designing treatment plans. Therapists use external forces to help patients regain mobility after injuries or surgeries. By carefully applying forces through exercises and therapies, they can help patients achieve controlled movements that aid in recovery. The impact of external forces on motion and acceleration also extends to safety measures. In automotive safety, airbags deploy with a specific force to decelerate occupants during crashes, reducing the risk of injury by managing the acceleration of the body. Similarly, in construction, safety harnesses are designed to absorb forces during falls, limiting the acceleration experienced by workers to prevent severe injuries. In conclusion, the interplay between motion and acceleration due to external forces is a cornerstone of various scientific and technological applications. From engineering and robotics to sports science and medical rehabilitation, understanding how forces influence motion enables us to design more efficient systems, enhance performance, and ensure safety across diverse domains. This fundamental principle underscores the significance of external forces in shaping our world and improving our lives.
2. Energy Transfer and Work Done
Energy transfer and work done are fundamental concepts that underpin the understanding of external forces and their impact on various systems. When an external force is applied to an object, it can cause the object to move or change its state of motion, which is a manifestation of energy transfer. This process is quantified through the concept of work done, defined as the product of the force applied and the displacement of the object in the direction of the force. Mathematically, work done (W) is expressed as \( W = F \cdot d \cdot \cos(\theta) \), where \( F \) is the magnitude of the force, \( d \) is the displacement, and \( \theta \) is the angle between the force and displacement vectors. The significance of energy transfer and work done extends across multiple domains. In mechanical systems, understanding these concepts is crucial for designing efficient machines and mechanisms. For instance, in automotive engineering, optimizing the work done by the engine on the wheels directly impacts fuel efficiency and vehicle performance. Similarly, in construction, calculating the work done by forces such as gravity and tension helps engineers design structures that can withstand various loads without collapsing. In thermodynamics, energy transfer plays a pivotal role in understanding heat engines and refrigeration systems. Here, work done is often associated with the conversion of thermal energy into mechanical energy or vice versa. This principle is essential for developing more efficient power plants and HVAC systems that minimize energy losses while maximizing output. From a biological perspective, energy transfer is vital for understanding muscle mechanics and locomotion. Muscles generate force through chemical reactions that convert biochemical energy into mechanical work, enabling movement and maintaining posture. This intricate balance of forces and energy transfer is critical for human health and performance. Moreover, in electrical systems, work done by electric forces drives the flow of electric current. The concept of voltage (electric potential difference) can be seen as a measure of the work done per unit charge to move charges from one point to another. This understanding is fundamental for designing electrical circuits and ensuring safe and efficient transmission of electrical energy. In everyday life, the impact of energy transfer and work done is ubiquitous. For example, when you lift a heavy object, you are doing work against gravity; when you pedal a bicycle, you are converting chemical energy from your muscles into mechanical work that propels the bike forward. These interactions highlight how external forces shape our environment and influence our daily activities. In conclusion, the interplay between energy transfer and work done is a cornerstone of understanding how external forces influence various systems. By grasping these concepts, we can design more efficient technologies, optimize performance in mechanical and biological systems, and appreciate the intricate balance of forces that govern our world. This foundational knowledge not only enhances our technological capabilities but also deepens our understanding of the natural world and our place within it.
3. Real-World Applications in Engineering and Physics
External forces play a pivotal role in various real-world applications across engineering and physics, driving innovation and problem-solving in diverse fields. One significant application is in **Structural Engineering**, where understanding external forces such as gravity, wind, and seismic activity is crucial for designing buildings, bridges, and other infrastructure. Engineers must calculate the stresses and strains these forces impose on structures to ensure stability and safety. For instance, the design of skyscrapers involves meticulous analysis of wind resistance to prevent structural failure, while bridges are engineered to withstand both static loads from traffic and dynamic forces from natural disasters like earthquakes. Another critical area is **Aerospace Engineering**, where external forces like aerodynamic drag, lift, and thrust are fundamental to the design and operation of aircraft and spacecraft. The interaction between these forces determines the performance characteristics of vehicles, such as speed, maneuverability, and fuel efficiency. In rocket propulsion, for example, the balance between thrust generated by engines and the drag encountered during ascent is meticulously managed to achieve optimal trajectory and payload capacity. In **Materials Science**, external forces are used to enhance material properties through processes like mechanical alloying and high-pressure torsion. These techniques involve applying intense external forces to alter the microstructure of materials, leading to improved strength, ductility, and resistance to wear and corrosion. This is particularly relevant in the development of advanced materials for applications ranging from biomedical implants to high-performance sports equipment. Furthermore, in **Robotics and Mechatronics**, understanding external forces is essential for the design of robotic systems that interact with their environment. Robots must be able to apply precise forces to perform tasks such as assembly, manipulation, and locomotion. Advanced sensors and actuators are used to measure and control these forces, enabling robots to adapt to changing conditions and maintain stability. Lastly, in **Medical Physics**, external forces are harnessed in diagnostic and therapeutic technologies. For example, magnetic resonance imaging (MRI) relies on strong magnetic fields to align hydrogen nuclei within the body, while ultrasound technology uses sound waves to create images of internal structures. In physical therapy, controlled external forces are applied through devices like orthotics and prosthetics to aid in patient rehabilitation. In summary, the impact of external forces is multifaceted and far-reaching, influencing a wide range of engineering and physics applications. From ensuring structural integrity to enhancing material properties, and from propelling spacecraft to aiding medical diagnostics, understanding and manipulating external forces are key to advancing technology and solving complex problems in various fields. This underscores the importance of studying external forces as a fundamental aspect of both engineering and physics.