What Is A Positive Control
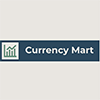
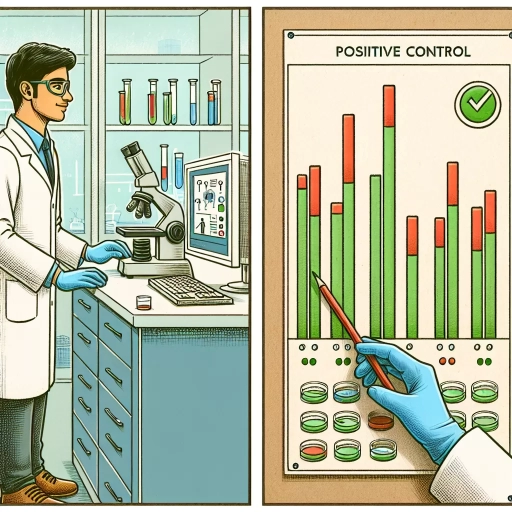
Understanding the Concept of a Positive Control
Understanding the concept of a positive control is crucial in scientific research, as it serves as a cornerstone for validating experimental results and ensuring the reliability of scientific findings. This concept is multifaceted, encompassing several key aspects that are essential for its effective application. First, it is important to grasp the definition and purpose of a positive control, which involves identifying a known variable that consistently produces a predictable outcome. This foundational understanding sets the stage for its practical implementation. Second, delving into the historical context and development of positive controls provides insight into how this concept has evolved over time, influenced by various scientific discoveries and methodologies. Finally, recognizing the importance of positive controls in scientific research highlights their critical role in maintaining the integrity and reproducibility of experiments. By exploring these dimensions, researchers can better appreciate the significance of positive controls and their indispensable contribution to the scientific process. Let us begin by examining the definition and purpose of a positive control, which forms the bedrock of this essential scientific tool.
Definition and Purpose
In the context of scientific experimentation, particularly in fields such as biology, chemistry, and medicine, the concept of a **positive control** is crucial for validating the reliability and accuracy of experimental results. A **positive control** is a sample or setup within an experiment that is known to produce a specific, expected outcome. This control serves as a benchmark against which the results of other samples or treatments can be compared. The primary purpose of a positive control is to ensure that the experimental conditions and methods are functioning correctly. For instance, in a biochemical assay designed to detect the presence of an enzyme, a positive control might include a known sample containing the enzyme. If this control sample yields the expected result—such as a color change or fluorescence—it confirms that the assay is working properly. This validation is essential because it allows researchers to trust that any negative results obtained from other samples are due to the absence of the enzyme rather than a failure of the assay itself. The definition and purpose of a positive control are intertwined with the principles of experimental design. By incorporating positive controls into their experiments, scientists can mitigate potential sources of error and ensure that their findings are robust and reproducible. For example, in clinical trials evaluating new drugs, positive controls might involve established treatments known to be effective for the condition being studied. If the new drug performs similarly to or better than the positive control, it suggests that the drug is likely effective. Moreover, positive controls help in troubleshooting experimental protocols. If unexpected results are obtained and the positive control does not yield the anticipated outcome, it indicates a problem with the experimental setup or reagents. This allows researchers to identify and correct issues before proceeding with further experimentation. In summary, the definition of a positive control as a sample or setup known to produce a specific outcome underscores its critical role in scientific research. The purpose of a positive control is multifaceted: it validates experimental methods, ensures reliability of results, aids in troubleshooting, and enhances the overall credibility of scientific findings. By understanding and effectively utilizing positive controls, researchers can ensure that their experiments are rigorous, reliable, and meaningful. This foundational concept is essential for advancing knowledge across various scientific disciplines and for making informed decisions based on empirical evidence.
Historical Context and Development
The concept of a positive control has its roots deeply embedded in the historical context of scientific experimentation, particularly within the fields of biology, chemistry, and medicine. The development of this crucial experimental tool can be traced back to the early days of scientific inquiry when researchers sought to validate their methods and ensure the reliability of their findings. In the 17th and 18th centuries, scientists like Robert Boyle and Antoine Lavoisier laid the groundwork for modern experimental design by emphasizing the importance of controlled conditions. However, it was not until the late 19th and early 20th centuries that the concept of a positive control began to take shape. During this period, pioneers such as Louis Pasteur and Robert Koch introduced rigorous standards for scientific experimentation. Pasteur's work on vaccination and Koch's postulates for proving microbial causation of diseases highlighted the necessity of having a known outcome to compare against. This was the genesis of the positive control—a sample or condition known to produce a specific result, thereby serving as a benchmark for the experiment's validity. The positive control ensured that any observed effects were due to the experimental treatment rather than other variables. In the 20th century, the rise of molecular biology further solidified the role of positive controls. With the advent of techniques like PCR (Polymerase Chain Reaction) and DNA sequencing, researchers needed reliable standards to verify that their methods were working correctly. Positive controls became essential in these contexts, allowing scientists to confirm that their reagents and protocols were functioning as expected. The development of positive controls has also been influenced by advances in statistical analysis and experimental design. The work of statisticians such as Ronald Fisher and Karl Pearson introduced principles of randomization and replication, which underscored the importance of controls in minimizing bias and ensuring reproducibility. Today, positive controls are an integral part of virtually all scientific experiments, from basic research to clinical trials. In contemporary science, the use of positive controls extends beyond traditional laboratory settings. In fields like environmental science and public health, positive controls help in validating monitoring protocols and ensuring that data collected are accurate and meaningful. For instance, in water quality testing, a positive control might involve spiking a sample with a known concentration of a contaminant to verify that the detection method is effective. Understanding the historical context and development of positive controls is crucial for appreciating their significance in modern scientific practice. By recognizing how these tools evolved over time, researchers can better design experiments that yield reliable results. The inclusion of a positive control in any experimental setup not only enhances the credibility of the findings but also ensures that the scientific community can trust and build upon those results. This foundational element of experimental design continues to play a vital role in advancing our understanding of the world around us.
Importance in Scientific Research
Scientific research is the cornerstone of advancements in various fields, and its importance cannot be overstated. At the heart of rigorous scientific inquiry lies the concept of controls, particularly the positive control, which plays a crucial role in validating experimental results. The significance of scientific research stems from its ability to systematically investigate phenomena, test hypotheses, and provide evidence-based conclusions. This process is essential for expanding our understanding of the world, driving innovation, and addressing complex problems. In the context of understanding a positive control, it is vital to recognize that scientific research relies heavily on the meticulous design of experiments. A positive control serves as a benchmark against which the outcomes of an experiment are measured. It ensures that the experimental conditions are conducive to producing the expected results, thereby validating the methodology and reagents used. For instance, in a biochemical assay, a positive control might involve using a known substance that consistently yields a specific response, confirming that the assay is functioning correctly. The importance of scientific research extends beyond the laboratory. It underpins technological advancements, informs policy decisions, and improves public health. For example, research on vaccines has saved countless lives by providing a scientific basis for their development and efficacy. Similarly, environmental research helps policymakers develop strategies to mitigate climate change and protect biodiversity. Moreover, scientific research fosters critical thinking and problem-solving skills. It encourages curiosity, creativity, and collaboration among researchers from diverse disciplines. The iterative process of formulating hypotheses, conducting experiments, analyzing data, and drawing conclusions enhances our analytical abilities and promotes a culture of continuous learning. Furthermore, scientific research is a global endeavor that transcends national boundaries. International collaborations in fields such as astronomy, genetics, and medicine have led to groundbreaking discoveries that benefit humanity as a whole. The sharing of knowledge and resources accelerates progress and ensures that findings are robust and reliable. In conclusion, the importance of scientific research is multifaceted and far-reaching. It not only advances our understanding of the world but also drives innovation, improves quality of life, and fosters global cooperation. The concept of a positive control is integral to this process, ensuring the integrity and reliability of experimental results. By understanding and appreciating the role of positive controls within the broader framework of scientific research, we can better grasp the significance of rigorous experimentation in advancing human knowledge.
Types and Applications of Positive Controls
In the realm of scientific research and experimentation, positive controls play a crucial role in ensuring the validity and reliability of results. These controls serve as benchmarks to verify that experimental procedures are functioning correctly, thereby enhancing the credibility of the findings. The types and applications of positive controls are diverse and multifaceted, encompassing various domains. This article delves into three primary categories: Biological Positive Controls, Chemical Positive Controls, and Technological Positive Controls. Biological Positive Controls involve the use of living organisms or biological samples to validate experimental outcomes. For instance, in genetic studies, a known gene sequence can be used as a positive control to confirm the efficacy of PCR (Polymerase Chain Reaction) techniques. Chemical Positive Controls, on the other hand, utilize specific chemicals to ensure that chemical reactions or assays are performing as expected. These controls are essential in fields such as pharmacology and toxicology where precise chemical interactions are critical. Technological Positive Controls leverage advanced technologies like software algorithms and hardware systems to verify the accuracy of data collection and analysis. This is particularly important in fields like bioinformatics and computational biology where data integrity is paramount. Understanding these different types of positive controls is essential for maintaining rigorous scientific standards. By exploring each category in depth, researchers can better design experiments that yield reliable results. Let us begin by examining the role of Biological Positive Controls in scientific research.
Biological Positive Controls
**Biological Positive Controls** In the realm of scientific research and experimentation, biological positive controls play a crucial role in ensuring the validity and reliability of experimental outcomes. These controls are essential components that help researchers verify the efficacy of their methods, reagents, and equipment. Essentially, a biological positive control is a sample or condition known to produce a specific, expected result when subjected to a particular experimental procedure. This known outcome serves as a benchmark against which the results of other samples can be compared. One of the primary types of biological positive controls involves using well-characterized biological samples that are known to respond in a predictable manner to the experimental conditions. For instance, in molecular biology experiments, a positive control might include a DNA sample known to contain a specific gene or sequence that the researcher is attempting to detect. When this sample is processed alongside experimental samples, it helps confirm that the PCR (Polymerase Chain Reaction) primers, enzymes, and other reagents are functioning correctly. Similarly, in cell biology studies, a positive control could be a cell line known to express a particular protein or exhibit a specific behavior under certain conditions. The applications of biological positive controls are diverse and widespread across various scientific disciplines. In clinical diagnostics, positive controls are used to validate the performance of diagnostic tests. For example, in HIV testing, a positive control sample containing the virus is used to ensure that the test kit can accurately detect the presence of HIV. This not only helps in confirming the sensitivity of the test but also in maintaining quality control standards. In drug discovery and development, biological positive controls are indispensable for assessing the efficacy of potential therapeutic agents. Researchers use cell lines or animal models known to respond to certain drugs as positive controls to evaluate the effectiveness of new compounds. This approach allows for the comparison of new drug candidates against established treatments, providing valuable insights into their potential therapeutic benefits. Moreover, biological positive controls are critical in environmental monitoring and toxicology studies. Here, they help in assessing the impact of pollutants on biological systems. For example, a positive control might involve exposing a known sensitive species to a toxic substance to gauge its effects, thereby validating the experimental setup and ensuring that any observed effects in other samples are due to the pollutant rather than experimental errors. In addition to their practical applications, biological positive controls also contribute significantly to the advancement of scientific knowledge. By providing a reliable reference point, these controls enable researchers to refine their methods, troubleshoot experimental issues, and interpret data with greater confidence. This, in turn, enhances the reproducibility and reliability of scientific findings, which are fundamental to the scientific method. In summary, biological positive controls are vital tools in scientific research, offering a robust means of validating experimental procedures and ensuring the accuracy of results. Their widespread use across various fields underscores their importance in maintaining high standards of scientific rigor and integrity. By incorporating these controls into experimental designs, researchers can enhance the credibility and impact of their work, ultimately contributing to a deeper understanding of biological systems and the development of innovative solutions in medicine, diagnostics, and beyond.
Chemical Positive Controls
Chemical positive controls are a crucial component in various scientific experiments, particularly in fields such as biochemistry, molecular biology, and pharmacology. These controls involve the use of specific chemicals or substances that are known to produce a predictable and consistent response under controlled conditions. The primary purpose of chemical positive controls is to validate the experimental setup, ensuring that the methods and reagents used are functioning correctly. For instance, in enzyme assays, a chemical positive control might be a known substrate that the enzyme acts upon, confirming that the enzyme is active and the assay conditions are optimal. Similarly, in drug discovery, chemical positive controls can be reference compounds with established pharmacological activity, helping to verify the efficacy of new compounds being tested. The types of chemical positive controls vary widely depending on the nature of the experiment. In analytical chemistry, standards such as certified reference materials (CRMs) serve as positive controls to calibrate instruments and ensure accurate quantification of analytes. In molecular biology, plasmids or DNA fragments with known sequences can be used as positive controls for PCR (Polymerase Chain Reaction) or sequencing reactions to confirm the integrity of the DNA and the efficiency of the reaction. In cell biology, positive controls might include specific agonists or antagonists that elicit a well-characterized cellular response, such as cell proliferation or apoptosis. The applications of chemical positive controls are diverse and critical across different research areas. In clinical diagnostics, positive controls are essential for validating the performance of diagnostic kits and ensuring the accuracy of test results. For example, in COVID-19 testing, positive controls containing viral RNA or antigens are used to verify that the test can detect the virus reliably. In environmental monitoring, chemical positive controls help in the validation of methods for detecting pollutants, ensuring that the analytical techniques are sensitive and specific enough to detect contaminants at relevant concentrations. Moreover, chemical positive controls play a pivotal role in quality control and quality assurance processes in industries such as pharmaceuticals and biotechnology. They help in maintaining consistency and reliability in manufacturing processes by ensuring that raw materials, intermediates, and final products meet specified standards. This is particularly important for regulatory compliance, as regulatory agencies require robust validation of analytical methods and manufacturing processes. In addition to their practical applications, chemical positive controls also contribute significantly to the advancement of scientific knowledge. By providing a baseline for comparison, these controls enable researchers to distinguish between true experimental effects and artifacts or errors in the experimental setup. This helps in building confidence in the results obtained and facilitates the interpretation of data in a more accurate and meaningful way. In summary, chemical positive controls are indispensable tools in scientific research and industrial applications. They ensure the reliability and validity of experimental results, facilitate the development of new methods and products, and are crucial for maintaining high standards in quality control and regulatory compliance. Their diverse applications underscore their importance as a fundamental aspect of rigorous scientific inquiry and industrial practice.
Technological Positive Controls
Technological positive controls are integral components in various scientific and industrial processes, ensuring the reliability and accuracy of experiments, tests, and operations. These controls leverage advanced technologies to provide a benchmark against which the performance of a system, process, or experiment can be measured. In the context of laboratory research, technological positive controls often involve standardized samples or conditions that are known to produce a specific outcome. For instance, in molecular biology, a positive control might be a DNA sample known to contain a particular gene sequence, which is used to validate the efficacy of PCR (Polymerase Chain Reaction) primers. This ensures that any failure to detect the gene in experimental samples is not due to the primers themselves but rather to the absence of the gene in those samples. In clinical diagnostics, technological positive controls are crucial for validating the sensitivity and specificity of diagnostic tests. For example, in COVID-19 testing, a positive control might be a sample containing a known quantity of SARS-CoV-2 RNA. This control helps in confirming that the test can accurately detect the virus when it is present, thereby enhancing confidence in test results. Similarly, in pharmaceutical manufacturing, technological positive controls are used to ensure the quality and potency of drugs. Here, a positive control could be a reference standard of a drug that is known to have a specific effect, which is used to compare against the efficacy of the manufactured drug. Technological positive controls also play a significant role in environmental monitoring. In water quality testing, for instance, a positive control might involve adding a known concentration of a pollutant to a water sample to verify that the testing method can accurately detect and quantify the pollutant. This ensures that any negative results are not due to methodological flaws but rather to the absence of the pollutant. Moreover, technological positive controls are essential in software development and cybersecurity. In software testing, positive controls can be simulated scenarios designed to trigger specific responses from the system, ensuring that it behaves as expected under various conditions. For example, a positive control might involve simulating a user login attempt with valid credentials to verify that the system grants access correctly. In cybersecurity, positive controls can include simulated attacks or penetration tests to evaluate the robustness of security measures. The applications of technological positive controls extend beyond these examples, encompassing fields such as food safety testing, where controls are used to ensure that detection methods for pathogens like Salmonella or E. coli are functioning correctly. In each of these contexts, technological positive controls serve as critical checkpoints that validate the integrity of processes and ensure that results are reliable and meaningful. By leveraging advanced technologies to create these controls, scientists and practitioners can enhance the precision and trustworthiness of their work, ultimately contributing to better decision-making and outcomes across diverse domains.
Best Practices for Implementing Positive Controls
Implementing positive controls is a crucial step in ensuring the reliability and accuracy of experimental results across various scientific disciplines. Best practices in this area are multifaceted, involving several key components that collectively enhance the validity of research findings. First, **Selecting Appropriate Positive Controls** is essential to establish a baseline for comparison, ensuring that the experimental conditions are correctly calibrated. This involves choosing controls that are relevant to the specific research question and can accurately reflect the expected outcomes. Second, **Standardization and Validation** are critical to maintain consistency and verify the efficacy of the controls, thereby ensuring reproducibility of results. This process involves rigorous testing and calibration to confirm that the controls perform as expected under different conditions. Finally, **Troubleshooting Common Issues** is vital to address any deviations or anomalies that may arise during the experiment, allowing researchers to identify and rectify problems promptly. By focusing on these three areas, researchers can significantly improve the quality and reliability of their experiments. To begin, it is imperative to delve into the process of selecting appropriate positive controls, as this foundational step sets the stage for all subsequent actions.
Selecting Appropriate Positive Controls
When implementing positive controls in scientific experiments, selecting the appropriate controls is crucial for ensuring the validity and reliability of the results. A positive control is a sample or condition that is known to produce a specific outcome, serving as a benchmark to verify that the experimental setup is functioning correctly. The key to effective positive controls lies in their ability to confirm that the experimental conditions are sufficient to elicit the expected response. To select appropriate positive controls, researchers must first understand the nature of their experiment and the specific variables being tested. For instance, in molecular biology experiments involving PCR (Polymerase Chain Reaction), a positive control might include a known DNA template that consistently amplifies under standard conditions. This ensures that any failure to amplify the target DNA in experimental samples is not due to issues with the PCR reagents or equipment but rather with the sample itself. In drug efficacy studies, a positive control could be a well-established drug known to produce a significant effect on the target condition. This helps in validating the experimental model and confirming that the observed effects are indeed due to the drug being tested rather than experimental artifacts. Similarly, in cell culture experiments, using cells that are known to respond to a particular stimulus can serve as a positive control, ensuring that the cells are healthy and responsive. Another critical aspect is ensuring that the positive control is relevant to the specific experimental context. For example, if studying a disease model in mice, using a strain that is genetically predisposed to develop the disease can serve as an effective positive control. This approach helps in validating the disease model and ensuring that any observed effects are consistent with what is expected based on prior knowledge. Moreover, it is essential to use multiple positive controls whenever possible to account for potential variability. This could involve using different concentrations of a known substance or multiple strains of cells that are expected to respond similarly. By doing so, researchers can increase confidence in their results and mitigate the risk of false negatives or false positives. Finally, documenting and standardizing the use of positive controls is vital for reproducibility. Detailed protocols should be maintained, including information on the source and preparation of the positive control samples. This not only facilitates replication of the experiment by other researchers but also ensures consistency across different batches of experiments conducted within the same laboratory. In summary, selecting appropriate positive controls is a cornerstone of rigorous scientific experimentation. By choosing controls that are relevant, reliable, and well-documented, researchers can ensure that their experiments yield accurate and meaningful results. This practice not only enhances the credibility of the findings but also contributes to the broader scientific community by promoting transparency and reproducibility.
Standardization and Validation
Standardization and validation are crucial components in the implementation of positive controls, ensuring that experimental results are reliable, reproducible, and accurate. Standardization involves establishing consistent protocols and procedures across different experiments to minimize variability. This includes using standardized reagents, instruments, and environmental conditions to ensure that all experiments are conducted under the same parameters. For instance, in molecular biology, standardizing the concentration of primers and enzymes in PCR reactions helps in achieving consistent amplification results. Similarly, in clinical trials, standardizing patient selection criteria and treatment protocols ensures that the study population is homogeneous and the outcomes are comparable. Validation, on the other hand, confirms that the methods and materials used in an experiment are effective and yield accurate results. This process involves verifying that the positive control behaves as expected under various conditions. For example, in immunohistochemistry, validating a new antibody involves testing it against known positive and negative controls to ensure it specifically binds to the target antigen without cross-reacting with other proteins. In drug development, validating a positive control compound means confirming its efficacy and safety through rigorous testing to serve as a benchmark for new compounds. The integration of standardization and validation enhances the reliability of positive controls by reducing errors and increasing precision. When these practices are adhered to, researchers can confidently interpret their data, knowing that any observed effects are due to the experimental variables rather than procedural inconsistencies. Moreover, standardized and validated positive controls facilitate the comparison of results across different studies and laboratories, promoting collaboration and advancing scientific knowledge more efficiently. In practice, implementing these best practices involves meticulous documentation of all procedures and results. This includes detailed records of reagent preparation, instrument calibration, and environmental conditions. Regular quality control checks also help in maintaining consistency over time. Additionally, participating in inter-laboratory comparisons and adhering to international standards (such as ISO guidelines) further ensures that the methods used are globally recognized and accepted. Ultimately, the rigorous application of standardization and validation in positive controls not only strengthens the scientific integrity of research but also accelerates the translation of findings into practical applications. By ensuring that experimental results are trustworthy and reproducible, scientists can build upon existing knowledge with confidence, driving innovation and progress in various fields such as medicine, biotechnology, and environmental science. Thus, these practices are essential for maintaining high standards in scientific research and ensuring that positive controls serve their intended purpose effectively.
Troubleshooting Common Issues
When implementing positive controls, it is crucial to be prepared for and adept at troubleshooting common issues that may arise. Positive controls, by definition, are procedures or measures designed to ensure that a system or process operates as intended, often serving as a benchmark against which other results can be compared. However, even with meticulous planning, challenges can emerge. One of the most frequent issues is **inconsistent results**, which can stem from various factors such as variability in reagents, equipment malfunctions, or human error. To address this, it is essential to maintain strict quality control protocols, including regular calibration of equipment and rigorous training for personnel involved in the process. Another common problem is **false positives or negatives**, which can significantly skew the accuracy of your data. This often occurs due to contamination, improper sample handling, or incorrect settings on analytical instruments. To mitigate these risks, ensure that all samples are handled in a sterile environment and that instruments are properly validated before use. Additionally, implementing multiple layers of quality checks and using control samples can help identify and rectify such issues promptly. **Systematic errors** are another area of concern. These errors can arise from flawed methodologies or inherent biases in the system design. For instance, if a positive control is not accurately representative of the conditions being tested, it may lead to misleading conclusions. To avoid this, it is vital to validate the positive control under various conditions to ensure its reliability across different scenarios. **Technical glitches** can also hinder the effectiveness of positive controls. These might include software bugs, hardware failures, or connectivity issues with automated systems. Regular maintenance and updates of equipment, along with having backup systems in place, can help minimize downtime and ensure continuous operation. Moreover, **compliance and regulatory issues** must be considered. Positive controls must adhere to relevant standards and guidelines to ensure their validity and acceptance. Staying updated with the latest regulations and best practices is essential to avoid any legal or ethical complications. Finally, **communication breakdowns** within teams can lead to misunderstandings about the role and implementation of positive controls. Clear documentation and open communication channels are critical to ensure that all team members understand the importance and proper use of these controls. By being proactive in identifying and addressing these common issues through rigorous quality control, validation processes, regular maintenance, compliance adherence, and clear communication, you can significantly enhance the reliability and effectiveness of your positive controls. This not only ensures that your systems operate as intended but also builds trust and confidence in the outcomes derived from these controls. In the context of best practices for implementing positive controls, troubleshooting these common issues is an integral part of maintaining high standards and achieving accurate, reliable results.