What Is Phosphorylation
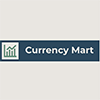
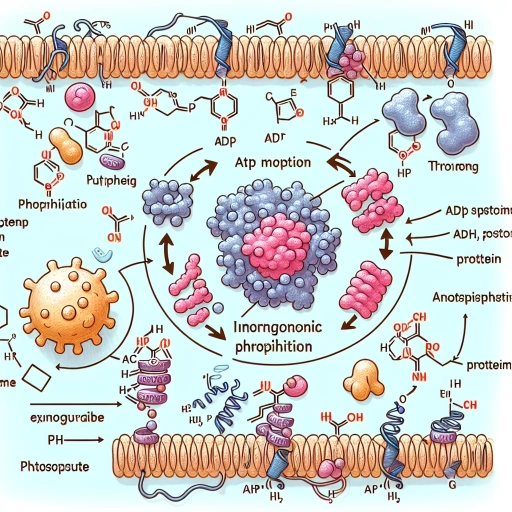
Understanding the Basics of Phosphorylation
Phosphorylation is a fundamental concept in the world of biochemistry with profound implications. To fully grasp its magnitude, we delve into three core areas. Initially, we delve into "The Science Behind Phosphorylation", exploring the elementary principles that underpin this process. This exploration employs easily digestible language and factual evidence to explain the intricate details of the phenomenon. Second, we illuminate "The Role of Phosphorylation in Biological Processes". Here, we contextualize the importance of phosphorylation within living organisms, demonstrating how it regulates vital functions such as metabolism and protein activation. Lastly, we demystify "The Types of Phosphorylation". Comprehending the complexity of phosphorylation is easier when one is familiar with its various forms - each with unique characteristics and functions. Having established a broad understanding of phosphorylation in the introduction, let us now delve deeply into the first point, "The Science Behind Phosphorylation", to provide a firm grounding in the science underpinning our subject. Each section's insights will collectively paint a comprehensive picture of the said topic.
The Science Behind Phosphorylation
Phosphorylation, an indispensable molecular mechanism in the realm of biology, is a process where a phosphate group is added to a protein molecule, thus playing a seminal part in managing cellular activity. The science behind this complex yet essential process lies in its ability to alter protein function swiftly and reversibly, hence modulating several cellular processes, from signal transduction to metabolism, and even orchestrating the dynamics of cellular life and death. Accordingly, the phenomenon implies a constant molecular 'tug of war' warranted by specific enzymes for phosphorylation (kinases) and dephosphorylation (phosphatases), depending on the cellular requirement at any given point in time. Elaborately, the core of this process is a reaction between the gamma phosphate group in ATP (adenosine triphosphate) and an -OH (hydroxyl group) on a protein. This union introduces a covalent bond where the energy-rich ATP’s phosphate is transferred to react with the recipient protein. This acts as a trigger for conformational changes in the protein molecule, thereby altering its activity. In a more tangible context, suppose the protein is an inactive lock. In that case, the phosphate, acting as a key inserted in the right spot, can spring the lock into activity, enabling or inhibiting its function. This precise action holds paramount importance because it serves a multitude of functions in our bodies, ranging from cellular communication to regulating hormone activity to controlling many metabolic reactions. However, the induction of a phosphate group does not persist indefinitely. Just as rapidly as the phosphate group is attached, the cellular machinery also expedites the removal of the group by using phosphatase enzymes, to switch off the protein activity when it is no longer required, securing a controlled and reversible switch. Thus, the scientific process of phosphorylation is a cornerstone in controlling most of the biological functions in our body, underlining its status as an inextricable part of cellular function and existence. It is a precise, controlled, and incredibly versatile process that warrants further exploration in future scientific studies.
The Role of Phosphorylation in Biological Processes
In the grand scheme of biological processes, phosphorylation plays an indispensable role. Acting as an on-off switch, phosphorylation modulates several biomolecular interactions and functions, facilitating a dynamic flow of life processes. The process entails the addition of a phosphate group to a protein molecule, a reaction that is directed by enzymes named kinases. This attachment of a phosphate group induces conformational changes in the protein, altering its activity, localization, and interaction with other molecules. Phosphorylation's reversible nature is what empowers it with significant control over biological functions. The phosphate group's add-on can be reversed by the action of another set of enzymes known as phosphatases. The equilibrium between kinase-driven phosphorylation and phosphatase-mediated dephosphorylation is integral for maintaining the homeostatic conditions in cells. Indeed, it is pivotal for signal transduction, the process crucial for communication between and within cells. Imbalances in phosphorylation have been linked to the onset of myriad human diseases, demonstrating its crucial role within the body. For instance, certain cancers and neurodegenerative disorders like Alzheimer's Disease are characterized by abnormal phosphorylation signaling routes. Likewise, phosphorylation identifies the cause and cures of diseases, as kinase inhibitors have demonstrated efficacy in cancer therapies. Moreover, phosphorylation permits cellular adaptation to environmental stimuli, signifying its role in evolutionary biology. It forms the core of metabolic regulation, bridging the energy cycle via ATP, the principal molecule for storing and transferring energy in cells. The transfer of the high-energy phosphate group from ATP to specific substrates in reactions fuels the biological machinery, highlighting how indispensable phosphorylation is to life. In all, the process of phosphorylation is a master regulator, influencing every aspect of cellular life. Its control over biological functions is critical for the proper functioning of cellular processes, as well as for adaptation and survival. Its understanding forms our comprehension of how biological processes are regulated—and misregulated—in physiology and disease, thereby making its role central to molecular and cellular biology.
The Types of Phosphorylation
Phosphorylation is an essential biological process that plays a fundamental role in various cell functions and signaling pathways. An integral part of understanding the basics of phosphorylation involves comprehending the different types of phosphorylation available. Broadly, phosphorylation can be classified into three prime categories: substrate-level phosphorylation, oxidative phosphorylation, and photophosphorylation. Each category is distinguished by its specific processes, mechanisms, and involvement in bioenergetic reactions. Firstly, substrate-level phosphorylation is a type of enzymatic reaction where a phosphate group is transferred directly from a high-energy level substrate to ADP (adenosine diphosphate), therefore yielding ATP (adenosine triphosphate). They are mostly seen in glycolysis and the citric acid cycle, and they do not need an external energy source. Secondly, the oxidative phosphorylation is responsible for the production of ATP in cellular respiration. It consists of the process where electrons are transferred from electron carriers to oxygen molecules resulting in ATP synthesis. This mechanism primarily occurs within mitochondria and involves a series of enzymatic reactions including the electron transport chain and chemiosmosis, which utilizes energy derived from the process of oxidation. Lastly, the photophosphorylation; a unique kind of phosphorylation exclusive to photosynthetic organisms. Light energy is used to promote the transfer of phosphates to ADP, generating ATP. The process takes place in chloroplasts, where it utilizes sunlight captured by chlorophyll. This mechanism is critical for the conversion of sunlight into chemical energy during photosynthesis. In conclusion, the variations of phosphorylation, namely substrate-level phosphorylation, oxidative phosphorylation, and photophosphorylation, are integral to energy production and exchange within biological systems. As phosphorylation remains a key player in numerous cellular functions and processes, a deeper understanding of its multifaceted types can lend significant insights into complex molecular dynamics and bioenergetic reactions.
The Mechanisms of Phosphorylation
Phosphorylation, a critical biochemical process underlying a multitude of cellular functions, has fascinated scientists for many years. The complexity of this process lies not only in the transformation of the molecules involved but also in the mechanisms propelling the alterations. The crux of understanding phosphorylation demands a comprehensive study of three predominant facets associated with this intricate procedure. These include the enzymes catalyzing the process, the biochemical alterations occurring during phosphorylation, and its pivotal role in signal transduction in proteins. An extensive exploration of these factors can illuminate the diverse functionality and pertinence of this ubiquitous cellular process. The initiation of this voyage into the profound mechanisms of Phosphorylation starts with the specialized biocatalysts, 'the enzymes.' These biological workhorses are critical to driving the process with extreme precision, modulating the rate of chemical change, and dictating the course of action within the cellular environment. Delving into the inner workings of enzymes involved in phosphorylation can unlock the secrets to the complex mechanisms that guide the dynamics of cellular life.
Enzymes Involved in Phosphorylation
Phosphorylation, as a metabolic process, hinges on the activity of enzymes, which function as the driving force behind its various mechanisms. To understand the depth and complexity of this process, one needs to delve into the role of these essential enzymes. The first key players in phosphorylation are Kinases, enzymes that catalyze the transfer of a phosphate group from high-energy donor molecules, such as ATP, to specific substrates, a process termed Phosphorylation. This action results in a functional change of the target protein by changing enzyme activity, cellular location, or association with other proteins. The human genome houses approximately 500 kinase enzymes, each of which plays a unique role in the phosphorylation process. The vast array of kinases is a testament to the varied and delicate roles of phosphorylation in cell biology. Phosphatases, on the other hand, are enzymes that remove a phosphate group from the substrate by hydrolysis, a reaction termed dephosphorylation. This mechanism brings balance into the phosphorylation process, highlighting the dynamic regulation between kinases and phosphatases in the cell. Latter regulates numerous cellular processes, ranging from cell division and growth to cell differentiation and apoptosis. Thus, the interplay of Kinases and Phosphatases in the phosphorylation-dephosphorylation process forms a key component of cellular homeostasis. Furthermore, there is the group of secondary enzymes that assist in modifying the phosphate group once it is attached to the target molecule. These enzymes, such as phosphoglycerate Mutase, function in tandem with kinases, playing instrumental roles in the propagation of the phosphorylation process and intricate metabolic pathways like Glycolysis and the Citric Acid Cycle. To encapsulate, enzymes involved in phosphorylation illustrate a harmonious yet complex metabolic ballet. This balanced interplay is fundamental to many critical cell functions and biochemical pathways. As researchers continue to delve deeper into the dynamics of phosphorylation, the remarkable roles of kinases, phosphatases, and secondary modifying enzymes become increasingly clear- unravelling the intricate lattice of life's biochemical processes.
The Biochemical Process of Phosphorylation
The biochemical process of phosphorylation is an intricately orchestrated scientific marvel that enables life's many physiological functions. This supporting paragraph serves to illuminate the vast mechanisms of phosphorylation, a subtitle under the overarching topic of what phosphorylation means. Phosphorylation is essentially a biochemical process where a phosphate group is added to a molecule, often a protein. This might sound simple, but the outcomes are profoundly impactful. The initiation of phosphorylation sets a series of actions into motion, essentially as a trigger mechanism. This triggering through phosphorylation is critical for maintaining the daily operation of cells and managing complex tasks such as metabolism, cell-division, and DNA repair. This process begins with an enzyme called a kinase. It identifies and attaches itself to the target protein within a cell. Then, driven by the power stored within ATP (adenosine triphosphate), the kinase enables the transfer of a phosphate group onto the protein. This transfer fundamentally alters the protein function, typically activating or deactivating it. What is remarkable about this process is its precision. Despite the myriad proteins found in a cell, kinase precisely identifies its target - like a lock and key. This level of specificity enables phosphorylation to act as a language of sorts. Through phosphorylation, cells communicate, regulate their actions, and maintain harmony within the complex cellular environment. But phosphorylation doesn’t act alone. In tandem with an equally important process, dephosphorylation, they form a potent pair, controlling and maintaining the fine balance within cells. Dephosphorylation, executed by phosphatases, does the opposite of kinases, removing the phosphate group and permitting a protein to return to its original function, effectively acting as an off-switch. Collectively, this dynamic and indispensable duo of Phosphorylation and dephosphorylation enables biochemical equilibrium inside our cells, governing life’s basic functions. It's akin to a relay race with the baton being the phosphate group, runners being the proteins, and kinases and phosphatases acting as the starters and finishers respectively, keeping the process cyclical and balanced. Never has the small phosphate group appeared more significant once its purpose within the realm of phosphorylation is understood. The seemingly simple act of adding or subtracting this group of atoms can, and does, command life as we know it. Digesting the vast implications initiated by this single biochemical process brings a newfound respect for nature’s ingenuity, a testament to the marvels of biology hidden within the microscopic world of our cells. The interplay between kinases, phosphate groups, and proteins reveals the effectively complex nature of this process, proving that cellular communication is more than just random interactions but is, in fact, a highly orchestrated symphony of biochemical reactions. This is the incredible story of phosphorylation, and it's just one of the many stories that lie at the heart of molecular biology.
Protein Phosphorylation and Signal Transduction
Understanding protein phosphorylation and its link to signal transduction is critical in comprehending the process of phosphorylation. Proteins are vital to nearly every activity in your cells, and their functionality heavily depends on small alterations, one vital type being protein phosphorylation. In simplest terms, protein phosphorylation is the addition of a phosphate group to a protein molecule. This seemingly nominal modification drastically changes the protein structure, creating a cascade of responses that can switch cellular activities on or off. This biological process is pivotal in controlling a myriad of cellular functions, including metabolism, transcription and translation, cell cycle progression, cytoskeletal rearrangement, protein–protein interactions, protein stability, cell movement, and apoptosis. Signal transduction, on the other hand, refers to the process through which external signals are transmitted into cells, triggering a response. Protein phosphorylation plays a crucial role in this process. The phosphate groups attached to proteins can be seen as coded messages being dispatched through the cellular network. The recipient of these messages can read and translate the code, enabling them to respond appropriately. In the signaling pathway, kinases are the primary writers of these coded messages. They are the enzymes responsible for transferring a phosphate group from high-energy, phosphate-donating molecules like ATP to proteins in a process known as phosphorylation. Once the protein is phosphorylated, it signals the next molecule and the message is propagated down a signaling cascade, leading finally to a cellular response. Protein phosphorylation and signal transduction are interwoven in a complex, yet precise regulatory network. They are integral parts of the phosphorylation process, acting as essential checks and balances for cellular function. Overally, understanding these two key components may open up multiple therapeutic options for diseases related to protein malfunction, including cancer, Alzheimer's, and diabetes.
Significance of Phosphorylation in Health and Diseases
Understanding the significant role that phosphorylation plays in both health and disease is key for groundbreaking healthcare breakthroughs. The process, a naturally occurring biochemical act within all human cells, allows for cellular function, signaling, and responsive capabilities. Its malfunction can lead to catastrophic health drifts, triggering a broad spectrum of diseases. Intriguing evidence in scientific literature reveals that irregularities in phosphorylation pathways are associated with cancer development, metabolic disorders, and neurodegenerative diseases. Unearthing this intricate symbiosis among phosphorylation and various disease pathologies holds the promise of enhancing and revolutionizing therapeutic strategies. The impending discourse focuses on the tri-dimensional correlation of phosphorylation, shedding light on its relationship with cancerous cells, the impact it has on metabolic problems, and how it connects with degenerative diseases damaging our nervous system. Delving first into how improper phosphorylation induces cancer progression, we shall explore this intricate balance where a single tip in the scale could potentially breed malignant cells.
Phosphorylation and Cancer
Phosphorylation is a crucial biochemical process, underlying various cell activities and vital bodily functions. It entails the addition of a phosphate group to a protein or other organic molecule within our body, driving a myriad of cellular processes, including metabolism, cell signaling, and DNA repair. However, its significance extends beyond these essential cellular functions, most notably impacting the development and progression of cancer—a severe life-threatening disease characterized by uncontrolled cell growth, and thus a focal point of extensive medical research. Abnormal phosphorylation patterns, often resulting from mutations in prominent kinase or phosphatases—responsible for adding and removing phosphate groups—are increasingly linked to cancer pathogenesis. Oncogenes and tumor suppressor genes, pivotal players in cancer development, are frequently the direct targets of these kinases and phosphatases. Mutations resulting in aberrant phosphorylation can trigger overactivation of oncogenes, driving uncontrolled cell proliferation—a hallmark of cancer. Alternatively, they can also weaken the function of tumor suppressors, dismantling the body’s natural defense against tumor growth. An example of this is found in the case of chronic myeloid leukemia (CML), where a fusion gene BCR-ABL constitutively activates a tyrosine kinase leading to abnormal protein phosphorylation, creating a malignant phenotype. Similarly, mutations in the BRAF gene, a kinase involved in the vital MAPK signaling pathway, lead to a form of phosphorylation that fuels the progression of various cancers, including malignant melanoma. Notably, better understanding of phosphorylation's role in cancer has paved the way for targeted therapies, primarily kinase inhibitors, offering new possibilities for cancer treatment. For example, Imatinib, a tyrosine-kinase inhibitor, effectively targets the BCR-ABL fusion protein in CML, exemplifying the promise of kinase-targeted cancer therapy. However, the complexity of the phosphorylation process necessitates further exploration to fully harness this potential. In essence, the significance of phosphorylation in health and diseases, particularly cancer, is multidimensional. It plays a crucial role in normal cellular functioning; however, aberrations can create a cascade of oncogenic triggers, substantiating the development and progression of cancer. Unfolding the complexities of phosphorylation is key to understanding cancer biology better and advancing our therapeutic strategies, ultimately bolstering our fight against this formidable disease.
Impact of Phosphorylation on Metabolic Disorders
Phosphorylation is a crucial biological process that plays a pivotal role in various functions, such as protein synthesis, cell division, and metabolism. Its impact on metabolic disorders is profound, and it's increasingly becoming a focal point for researchers aiming at understanding and treating such conditions. Phosphorylation is essentially a means of protein regulation, where a phosphate group is added to an enzyme or protein, thus altering its function. This switching on and off of proteins controls numerous metabolic reactions. In the context of metabolic disorders, inappropriate or abnormal phosphorylation can result in the maladjustment of metabolic processes, leading to health complications like diabetes, neurodegenerative diseases, and cancer. An illustrative example is insulin resistance, a hallmark of type 2 diabetes. Here, impaired phosphorylation of the insulin receptor substrate contributes to a decrease in insulin sensitivity, leading to high blood glucose levels. Anomalies in phosphorylation are also linked to obesity, another metabolic disorder. Obesity stems from energy imbalance due to the dysregulation of lipid and carbohydrate metabolism. Inflated fat mass leads to the alteration in phosphorylation of several proteins involved in energy balance, further exacerbating the condition. Furthermore, irregularities in phosphorylation are at the core of some cancers, which in essence, are metabolic diseases. Several tumor cells exhibit abnormal metabolic pathways and rely on certain nutrients for growth. Misregulated phosphorylation of proteins involved in these metabolic pathways may contribute to the survival and proliferation of cancer cells. Decoding the nuances of phosphorylation, therefore, holds immense potential for the marker-driven diagnosis and targeted treatment of metabolic disorders. Innovative techniques like mass spectrometry and next-generation sequencing offer a window into the complex relationships between phosphorylation and metabolic diseases. These findings could potentially lead to tailored therapies aimed at rectifying abnormal phosphorylation, thereby helping manage or even cure metabolic disorders. Indeed, the significance of phosphorylation in health and diseases cannot be overstated. Its understanding allows us to piece together the intricate puzzle of metabolic disorders, paving the way for novel therapeutic interventions.
The Role of Phosphorylation in Neurodegenerative Diseases
Phosphorylation plays a pivotal role in neurodegenerative disorders, a subtype of diseases that primarily influence the neurons in the human brain. Neurons are crucial for a plethora of tasks, including everything from thought processing to muscle coordination. Therefore, proper neuronal function is quintessential to maintaining a healthy cognitive state. However, neurodegenerative diseases, including Alzheimer's, Parkinson's, and Multiple Sclerosis cause neuron degradation and functional impairment, often culminating in severe cognitive decline. The process of Phosphorylation is notably impactful in the progression and mitigation of neurodegenerative disorders. As a post-translational modification, Phosphorylation adds a phosphate group to proteins changing its structure and ultimately influencing its function. Pathologically hyperphosphorylated proteins are a common element in most neurodegenerative disorders. In Alzheimer’s disease, for instance, hyperphosphorylation of the tau protein leads to the formation of neurofibrillary tangles, one of the signature pathological hallmarks of the disease. While in Parkinson's disease, phosphorylation of α-synuclein contributes to forming Lewy bodies, toxic aggregates contributing to neuronal death. Meanwhile, Phosphorylation also plays a part in neuroprotection and disease mitigation. Properly regulated phosphorylation processes can positively influence neuronal survival pathways and hence has potential therapeutic implications. For instance, recent studies suggest that increase phosphorylation of heat shock protein 27 can promote neuronal resistance to oxidative stress, a common denominator in neurodegenerative diseases. In the course of defining the role of phosphorylation in health and disease, it's clear that understanding and mapping these diverse phosphorylation events can offer profound insight into the pathogenesis of neurodegenerative diseases. Therefore, future research should be directed towards exploring this association in detail. This potentially enables the development of targeted drugs that modulate these phosphorylation pathways and offer relief to millions suffering from debilitating neurodegenerative diseases. The role of Phosphorylation in these diseases inherently exemplifies their significance in our health, thus reinforcing the importance of understanding such phenomenal biological processes.