What Is Rpp Contribution
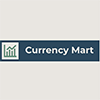
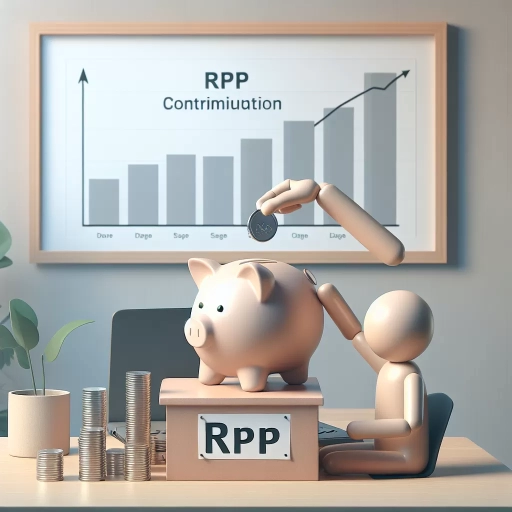
The concept of Radioactive Power Production (RPP) has emerged as a pivotal innovation in the realm of energy generation, offering a unique blend of sustainability and technological advancement. This article delves into the multifaceted contributions of RPP, exploring its historical context and development, technical aspects and mechanisms, and its impactful applications across various fields. By understanding the historical context and development of RPP, we gain insight into how this technology has evolved over time. The technical aspects and mechanisms of RPP reveal the intricate processes that make this form of energy production possible. Finally, examining the impact and applications of RPP in various fields highlights its potential to transform industries and address global energy challenges. This comprehensive overview begins with an exploration of the historical context and development of RPP, setting the stage for a deeper understanding of its significance and future implications.
Historical Context and Development of RPP
The historical context and development of Radioisotope Power Systems (RPP) is a rich and complex narrative that spans several decades. This evolution is best understood through three key aspects: the origins and early applications, the evolution over time, and the key milestones and innovations that have shaped this technology. From its inception, RPP has been driven by the need for reliable and long-lasting power sources in remote and challenging environments. The early applications of RPP were largely driven by space exploration, where traditional power sources were insufficient. As the technology evolved, so did its applications, expanding into various fields such as medicine, industry, and environmental monitoring. Significant milestones and innovations have marked this journey, transforming RPP from a nascent technology into a robust and indispensable tool. To fully appreciate this development, it is essential to delve into the origins and early applications of RPP, which laid the foundation for its subsequent growth and diversification. Transitioning to the origins and early applications, we explore how the initial experiments and deployments set the stage for what would become a pivotal technology in modern science and engineering.
Origins and Early Applications
The origins and early applications of Radioisotope Power Systems (RPS), a key component of Radioisotope Power and Propulsion (RPP), trace back to the mid-20th century when nuclear energy began to be harnessed for various purposes beyond electricity generation. The concept emerged in the 1950s, driven by the need for reliable, long-lasting power sources for space exploration. The first radioisotope thermoelectric generators (RTGs) were developed in the United States, leveraging the heat produced by radioactive decay to generate electricity. These early RTGs were crucial for powering satellites and deep space missions where solar panels were impractical due to distance from the Sun or variable sunlight exposure. One of the earliest and most significant applications was the launch of the SNAP-3B (Systems for Nuclear Auxiliary Power) in 1961, which powered the Transit 4A satellite. This marked a milestone in demonstrating the feasibility of nuclear power in space. Subsequent missions, such as the Apollo program, utilized RTGs to power instruments on the lunar surface, providing critical data during the Moon landings. The success of these early deployments paved the way for more advanced RPS technologies, including the Multi-Mission Radioisotope Thermoelectric Generator (MMRTG) used in NASA's Curiosity Rover mission to Mars in 2012. The development of RPS was not only driven by technological advancements but also by strategic collaborations between government agencies, research institutions, and private industry. For instance, the U.S. Department of Energy and NASA have been instrumental in funding and coordinating research efforts to improve efficiency, safety, and durability of RTGs. These collaborations have led to significant improvements, such as better thermoelectric materials and more efficient heat management systems. Early applications also extended beyond space exploration to include terrestrial uses, particularly in remote or harsh environments where conventional power sources were unreliable or impractical. For example, RTGs have been used in lighthouses and weather stations in remote areas, ensuring continuous operation without the need for frequent maintenance or fuel replenishment. In summary, the origins and early applications of RPS were characterized by innovative technological developments and strategic partnerships aimed at harnessing nuclear energy for reliable and long-lasting power generation. These early successes laid the foundation for the continued evolution of RPP technologies, enabling deeper space exploration and supporting critical infrastructure in challenging environments.
Evolution Over Time
Evolution over time is a fundamental concept that underpins the development and refinement of various scientific theories, including those in the realm of Resource Planning and Production (RPP). Historically, RPP has evolved significantly, driven by technological advancements, changing market dynamics, and the need for more efficient and effective resource allocation. In the early stages, RPP was largely manual and relied on basic forecasting methods. However, with the advent of the Industrial Revolution, there was a shift towards more mechanized processes. The introduction of the assembly line by Henry Ford in the early 20th century marked a significant milestone, as it necessitated more sophisticated planning to manage resources efficiently. The post-World War II era saw the rise of Operations Research (OR), which brought scientific methods to the forefront of resource planning. Techniques such as linear programming and simulation became integral tools for optimizing production processes. The 1960s and 1970s witnessed the emergence of Material Requirements Planning (MRP) systems, which revolutionized inventory management by ensuring that materials were available when needed. The 1980s introduced Manufacturing Resource Planning (MRP II), an extension of MRP that integrated additional functions such as capacity planning and financial management. This period also saw the beginning of Enterprise Resource Planning (ERP) systems, which further expanded the scope to include all aspects of business operations. In recent decades, advancements in technology have propelled RPP into the digital age. The advent of cloud computing, artificial intelligence (AI), and the Internet of Things (IoT) has enabled real-time data analytics, predictive maintenance, and dynamic resource allocation. Modern RPP systems leverage big data to forecast demand more accurately, optimize supply chains, and enhance overall operational efficiency. Moreover, the integration of Industry 4.0 technologies has transformed RPP into a highly interconnected and automated process. Smart factories equipped with sensors and AI-driven decision-making systems can adjust production schedules in real-time based on market conditions and resource availability. This evolution has not only improved productivity but also reduced costs and environmental impact. In summary, the evolution of RPP over time reflects a continuous quest for efficiency, accuracy, and adaptability. From manual processes to sophisticated AI-driven systems, each stage has built upon previous advancements to create a robust framework for managing resources effectively. This historical context underscores the significant contributions of RPP to modern industrial practices and highlights its ongoing role in driving innovation and competitiveness in various sectors.
Key Milestones and Innovations
The development of Radioisotope Power Systems (RPS) has been marked by several key milestones and innovations, each contributing significantly to their evolution and effectiveness. One of the earliest and most pivotal milestones was the launch of the first nuclear-powered satellite, SNAP-3A (Systems for Nuclear Auxiliary Power), in 1961. This mission demonstrated the feasibility of using radioisotope thermoelectric generators (RTGs) to power space missions, setting the stage for future advancements. In the 1960s and 1970s, RTGs became integral to several high-profile space missions, including the Apollo missions to the Moon. The Apollo 11 mission, for instance, utilized RTGs to power scientific instruments left on the lunar surface, providing crucial data long after the astronauts returned to Earth. This period also saw significant improvements in RTG design and efficiency, such as the development of the Multi-Mission Radioisotope Thermoelectric Generator (MMRTG), which has been used in more recent missions like the Mars Curiosity Rover. The 1980s witnessed further innovations with the introduction of the General Purpose Heat Source (GPHS) module, designed to improve safety and efficiency. This module has been a cornerstone of modern RTG design, ensuring that radioisotopes are safely contained and efficiently converted into electricity. The Cassini-Huygens mission to Saturn, launched in 1997, was another landmark event, as it relied on RTGs for power during its nearly two-decade journey and extensive exploration of the Saturnian system. In recent years, advancements in materials science and engineering have led to more efficient and durable RTGs. For example, the development of advanced thermoelectric materials has increased the power output per unit of radioisotope fuel, making RPS more viable for longer-duration missions. Additionally, the introduction of new safety features and containment systems has enhanced the overall reliability and safety of these systems. The historical context of RPS development underscores a continuous push for innovation driven by the need for reliable, long-lasting power sources in space exploration. Each milestone has built upon previous achievements, leading to the sophisticated RPS technologies that are now integral to many space missions. These innovations not only reflect technological progress but also highlight the critical role that RPS has played in expanding our understanding of the universe.
Technical Aspects and Mechanisms of RPP
Understanding the Technical Aspects and Mechanisms of Remote Patient Monitoring (RPM) is crucial for healthcare providers aiming to deliver high-quality, patient-centered care. This article delves into the core principles and theories, operational processes and steps, and technological advancements and tools that underpin RPM. At its core, RPM relies on **Core Principles and Theories** that guide the ethical, legal, and clinical frameworks necessary for effective patient monitoring. These principles ensure that data collection and analysis are conducted with integrity and patient safety in mind. The **Operational Processes and Steps** involve the systematic setup, data transmission, and continuous monitoring protocols that healthcare professionals must follow to ensure seamless care delivery. Finally, **Technological Advancements and Tools** such as wearable devices, mobile applications, and advanced analytics software play a pivotal role in enhancing the accuracy and efficiency of RPM. By grasping these interconnected elements, healthcare providers can optimize their RPM strategies. Let's begin by exploring the foundational **Core Principles and Theories** that serve as the backbone of this innovative healthcare approach.
Core Principles and Theories
The core principles and theories underlying the Technical Aspects and Mechanisms of Resource Planning and Production (RPP) are rooted in several key concepts that ensure efficient and effective resource allocation. **Optimization Theory** plays a crucial role, as it involves using mathematical models to maximize output while minimizing costs. This theory is applied through **Linear Programming**, which helps in allocating resources in the most efficient manner by solving complex equations that balance constraints and objectives. **Supply Chain Management (SCM) Theory** is another foundational principle, emphasizing the integration of all activities involved in the production process, from sourcing raw materials to delivering finished products. SCM ensures that each stage of the supply chain is optimized to reduce lead times, lower costs, and improve product quality. **Just-in-Time (JIT) Production** is a related concept that aims to produce and deliver products just in time to meet customer demand, thereby reducing inventory costs and improving responsiveness. **Lean Manufacturing** principles are also integral, focusing on eliminating waste and maximizing value-added activities. By identifying and eliminating non-value-added processes, organizations can streamline their operations, reduce variability, and enhance overall efficiency. **Total Quality Management (TQM)** complements these principles by emphasizing continuous improvement and customer satisfaction through rigorous quality control measures. **Economic Order Quantity (EOQ) Model** is another critical theory, helping organizations determine the optimal order quantity that minimizes total inventory costs. This model balances the trade-offs between holding costs and ordering costs to achieve cost-effectiveness. Additionally, **Material Requirements Planning (MRP)** systems are used to manage inventory levels and production schedules based on demand forecasts, ensuring that the right materials are available at the right time. **Theory of Constraints (TOC)** further enhances RPP by identifying and managing bottlenecks in the production process. By focusing on these constraints, organizations can optimize their entire system rather than just individual components. **Six Sigma** methodology also supports RPP by using data-driven approaches to reduce defects and variations, thereby improving process reliability and customer satisfaction. In summary, the technical aspects and mechanisms of RPP are underpinned by a robust set of principles and theories that collectively aim to optimize resource allocation, streamline operations, reduce costs, and enhance product quality. By integrating these concepts into their operations, organizations can achieve higher levels of efficiency, responsiveness, and customer satisfaction.
Operational Processes and Steps
Operational processes and steps are the backbone of any efficient system, including the Technical Aspects and Mechanisms of Risk-Based Performance Planning (RPP). RPP, a methodology aimed at optimizing performance by managing risks, relies heavily on well-defined operational processes to ensure its effectiveness. Here are the key operational steps involved: 1. **Risk Identification**: The first step involves identifying potential risks that could impact performance. This is typically done through workshops, interviews, and historical data analysis to pinpoint areas of vulnerability. 2. **Risk Assessment**: Once risks are identified, they need to be assessed in terms of likelihood and impact. This step often involves quantifying risks using probability and consequence matrices. 3. **Risk Prioritization**: Risks are then prioritized based on their assessed severity. This helps in focusing resources on the most critical risks first. 4. **Development of Mitigation Strategies**: For each identified risk, mitigation strategies are developed. These strategies could include preventive measures, contingency plans, or risk transfer mechanisms. 5. **Implementation and Monitoring**: The mitigation strategies are then implemented, and their effectiveness is continuously monitored. This involves setting up key performance indicators (KPIs) and regular review meetings. 6. **Review and Update**: The final step is a continuous review and update of the risk management plan. This ensures that new risks are identified promptly and existing strategies remain relevant. 7. **Stakeholder Engagement**: Throughout these steps, stakeholder engagement is crucial. Stakeholders provide valuable insights and ensure that the risk management plan aligns with organizational goals. 8. **Documentation and Reporting**: Detailed documentation of all steps taken and regular reporting to stakeholders are essential for transparency and accountability. By following these operational processes, organizations can ensure that their RPP is robust, effective, and aligned with their overall strategic objectives. These steps not only help in managing risks but also in enhancing overall performance by fostering a proactive and resilient organizational culture.
Technological Advancements and Tools
Technological advancements and tools have significantly enhanced the technical aspects and mechanisms of Remote Patient Monitoring (RPP), transforming the way healthcare is delivered and managed. At the forefront of these advancements are wearable devices and mobile health applications, which enable continuous monitoring of vital signs such as heart rate, blood pressure, and glucose levels. These devices transmit real-time data to healthcare providers, allowing for prompt interventions and personalized care plans. Advanced algorithms and machine learning technologies analyze this data to predict potential health risks, enabling proactive measures to prevent complications. Telehealth platforms have also become integral, facilitating virtual consultations and reducing the need for hospital visits. Additionally, Internet of Things (IoT) devices integrate various medical equipment, ensuring seamless communication and data exchange between different healthcare systems. Cloud computing and big data analytics further support RPP by providing secure storage and efficient processing of large volumes of patient data, enhancing decision-making capabilities for healthcare professionals. Overall, these technological tools not only improve patient outcomes but also streamline clinical workflows, making RPP a cornerstone of modern healthcare delivery.
Impact and Applications of RPP in Various Fields
The impact and applications of Radioisotope Power Systems (RPP) are multifaceted and far-reaching, influencing various fields in profound ways. This article delves into the economic and industrial contributions, environmental and social benefits, and future prospects of RPP. Economically, RPP has revolutionized industries by providing reliable and long-lasting power sources, particularly in remote or space exploration contexts. Industrially, these systems have enabled the development of advanced technologies that require consistent energy supply. Environmentally, RPP offers a cleaner alternative to traditional power sources, reducing carbon footprints and mitigating pollution. Socially, it enhances safety and reliability in critical applications such as medical devices and emergency response systems. Looking ahead, future prospects and emerging trends suggest even greater integration of RPP into diverse sectors, promising further innovation and sustainability. This article will explore these aspects in detail, starting with the significant economic and industrial contributions of RPP.
Economic and Industrial Contributions
The economic and industrial contributions of RPP (Recycled Plastics and Polymers) are multifaceted and significant, driving sustainability and efficiency across various sectors. In the manufacturing industry, RPP reduces raw material costs by substituting virgin plastics with recycled alternatives, thereby lowering production expenses and enhancing profit margins. This cost savings can be passed on to consumers, making products more affordable and competitive in the market. Additionally, the use of RPP helps in conserving natural resources such as petroleum, water, and energy that are otherwise required for producing new plastics. This conservation not only mitigates environmental impacts but also ensures a more stable supply chain by reducing dependence on finite resources. In terms of job creation and economic growth, the recycling industry itself is a substantial employer. The process of collecting, sorting, processing, and manufacturing products from recycled plastics generates employment opportunities at multiple levels, from manual labor to skilled engineering roles. This employment boost contributes to local economies and stimulates economic activity through payroll spending and tax revenues. Furthermore, the development of advanced recycling technologies and innovative applications for RPP fosters innovation and entrepreneurship, leading to the establishment of new businesses and industries focused on sustainable practices. From an environmental perspective, the economic benefits of RPP are closely tied to reduced waste management costs. By recycling plastics instead of sending them to landfills or incinerators, communities save on disposal fees and avoid the environmental hazards associated with improper waste disposal. This approach also helps in reducing greenhouse gas emissions linked to the production of new plastics, contributing to global efforts to combat climate change. In agriculture, RPP is used in the production of durable and lightweight farming equipment, irrigation systems, and packaging materials. These products offer improved resistance to weather conditions and pests, enhancing crop yields and reducing the need for frequent replacements. In construction, recycled plastics are used in making insulation materials, composite decking, and other building components that are more resilient and require less maintenance than traditional materials. The automotive sector also benefits significantly from RPP. Recycled plastics are used in manufacturing car parts such as dashboards, bumpers, and interior components. These parts are lighter than their metal counterparts, contributing to fuel efficiency and reducing carbon emissions. Additionally, the use of RPP in automotive manufacturing supports the industry's shift towards more sustainable practices while maintaining performance standards. Overall, the economic and industrial contributions of RPP are pivotal in driving a circular economy that values resource efficiency, sustainability, and innovation. By leveraging recycled plastics and polymers across diverse industries, we can achieve significant economic benefits while minimizing environmental impacts. This dual advantage underscores the importance of integrating RPP into various sectors as a key strategy for sustainable development.
Environmental and Social Benefits
The implementation of Recycled Polypropylene (RPP) across various fields yields significant environmental and social benefits. From a environmental perspective, RPP reduces the need for virgin polypropylene production, which in turn decreases the consumption of non-renewable resources such as petroleum and natural gas. This reduction minimizes greenhouse gas emissions associated with the extraction, processing, and transportation of these resources. Additionally, recycling polypropylene helps to conserve landfill space by diverting plastic waste from landfills and oceans, thereby mitigating marine pollution and protecting biodiversity. The recycling process itself is more energy-efficient compared to producing new polypropylene, further lowering carbon footprints. On the social front, the adoption of RPP promotes sustainable practices and raises awareness about the importance of recycling. It supports local economies by creating jobs in the recycling industry and stimulates innovation in waste management technologies. Communities benefit from cleaner environments and healthier living conditions, as reduced plastic waste leads to less pollution in waterways and soil. Moreover, the use of RPP in consumer products can influence consumer behavior towards more sustainable choices, fostering a culture of environmental responsibility. In industries such as packaging, automotive, and construction, RPP offers cost-effective alternatives without compromising on performance, making sustainable practices more accessible and affordable for businesses and consumers alike. Overall, the integration of RPP into various sectors not only enhances environmental sustainability but also contributes to social well-being by supporting economic growth and community health.
Future Prospects and Emerging Trends
As we delve into the future prospects and emerging trends of Radioisotope Power Systems (RPP), it becomes evident that these technologies are poised to revolutionize various fields. In the realm of space exploration, RPPs are expected to play a crucial role in powering missions to distant planets and moons, where solar energy is insufficient. For instance, NASA's Perseverance rover on Mars relies on a radioisotope thermoelectric generator (RTG) for its power needs, highlighting the reliability and efficiency of RPPs in extreme environments. In the medical sector, advancements in RPP technology could lead to more compact and durable power sources for implantable devices such as pacemakers and neurostimulators. This would significantly enhance patient care by reducing the need for frequent surgeries to replace batteries. Additionally, the development of smaller-scale RPPs could enable the creation of autonomous medical sensors and monitoring devices that can operate for extended periods without maintenance. The environmental monitoring field is also set to benefit from emerging trends in RPPs. Autonomous weather stations and oceanographic sensors powered by RPPs can provide continuous data collection in remote and harsh environments, contributing significantly to climate research and natural disaster prediction. Furthermore, these power systems can be integrated into IoT devices for real-time monitoring of air quality, water pollution, and other environmental parameters. In terms of energy policy and sustainability, RPPs offer a promising alternative for powering remote communities or disaster relief operations where traditional energy infrastructure is lacking. Their long-lasting nature and low maintenance requirements make them ideal for such applications. Moreover, as research continues to improve the efficiency and safety of RPPs, they may also find roles in supporting renewable energy systems by providing reliable backup power during periods of low energy production. From an economic perspective, the future prospects of RPPs are tied closely to advancements in materials science and manufacturing processes. Innovations in these areas could lead to more cost-effective production methods, making RPPs more accessible for a wider range of applications. This could stimulate economic growth by enabling new industries and creating jobs related to the development, deployment, and maintenance of these power systems. In conclusion, the future of RPPs is marked by significant potential across multiple domains. As technology continues to evolve, we can expect these power systems to become even more efficient, safe, and versatile. Their impact will be felt from the depths of space to the heart of our communities, driving innovation and sustainability in ways that were previously unimaginable.