What Is The Difference Between An Element And A Compound
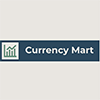
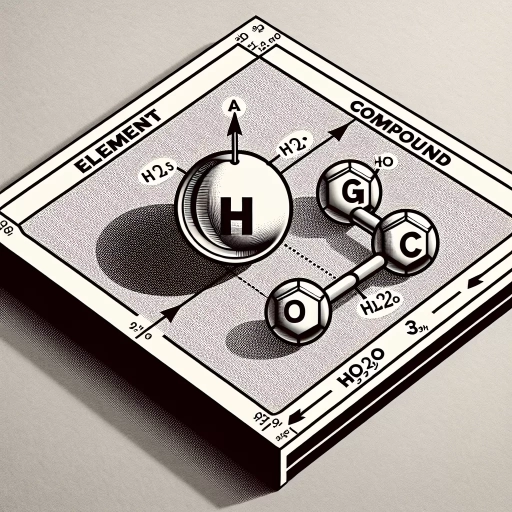
In the realm of chemistry, understanding the fundamental distinctions between elements and compounds is crucial for grasping the building blocks of matter and how they interact. Elements and compounds, though often discussed together, represent two distinct categories with unique properties and compositions. This article delves into the key differences between these two concepts, exploring their definitions and compositions, properties and characteristics, and formation processes along with illustrative examples. By examining these aspects, we will uncover how elements—pure substances consisting of a single type of atom—differ from compounds—substances formed by the chemical bonding of two or more different elements. We will begin by defining what constitutes an element and a compound, laying the groundwork for a deeper exploration of their properties and how they are formed. This foundational understanding is essential for navigating the complex world of chemistry, where the distinctions between elements and compounds are pivotal. Let us start with the **Definition and Composition** of these chemical entities to establish a clear understanding of their nature.
Definition and Composition
Understanding the fundamental concepts of definition and composition is crucial in the realm of chemistry, as it lays the groundwork for comprehending the intricate nature of substances and their interactions. At its core, chemistry revolves around the study of elements, compounds, and the bonds that form between them. This article delves into these essential components by first exploring **Elements: Pure Substances**, where we examine the building blocks of matter that cannot be broken down into simpler substances. Next, we discuss **Compounds: Mixture of Elements**, highlighting how elements combine in fixed ratios to form new substances with distinct properties. Finally, we delve into **Chemical Bonds in Compounds**, explaining the forces that hold these elements together within a compound. By grasping these concepts, we can better appreciate the definition and composition of substances, enabling a deeper understanding of their structure, properties, and behaviors. This comprehensive overview will provide a clear and engaging insight into the foundational principles of chemistry, ultimately illuminating the intricate world of definition and composition.
Elements: Pure Substances
Elements are the fundamental building blocks of matter, consisting of pure substances that cannot be broken down into simpler substances by chemical means. Each element is composed of atoms that have the same number of protons in their atomic nuclei, known as the atomic number, which uniquely identifies the element. For instance, hydrogen atoms all have one proton, while carbon atoms have six protons. This uniformity in atomic structure is what distinguishes elements from compounds. The purity and simplicity of elements are key to their definition. Unlike compounds, which are formed by the chemical bonding of different elements in fixed ratios, elements consist solely of one type of atom. For example, oxygen (O₂) is a molecule composed of two oxygen atoms and is considered an element because it consists entirely of oxygen. In contrast, water (H₂O) is a compound made up of hydrogen and oxygen atoms in a specific ratio. The composition of an element can be described in terms of its atomic mass, which is the total number of protons and neutrons in the nucleus of an atom. However, elements can also exist in different isotopes, which are atoms of the same element with varying numbers of neutrons. These isotopes have the same chemical properties due to their identical number of protons but differ in their physical properties such as mass. Understanding elements is crucial for grasping the broader concepts of chemistry and the natural world. Elements form the basis for all chemical reactions and interactions, and their unique properties determine how they react with other elements to form compounds. The periodic table of elements, which organizes elements by their atomic number and recurring chemical properties, is a powerful tool for chemists and scientists to predict and understand these interactions. In summary, elements are pure substances that consist solely of one type of atom and cannot be decomposed into simpler substances. Their composition is defined by their atomic number and can vary slightly through isotopes without changing their fundamental chemical nature. This distinction between elements and compounds is essential for understanding chemical reactions, material properties, and the fundamental structure of matter itself.
Compounds: Mixture of Elements
Compounds are fundamental entities in chemistry, distinguished by their composition of two or more different elements chemically bonded together. Unlike elements, which consist of only one type of atom, compounds are formed when atoms of different elements share or exchange electrons to form a stable molecule. This process results in a substance with properties distinct from those of its constituent elements. For instance, water (H₂O) is a compound composed of hydrogen and oxygen atoms. Individually, hydrogen is highly flammable and oxygen supports combustion, but when combined, they form water, which is neither flammable nor combustible. The composition of a compound is defined by its chemical formula, which specifies the exact number and type of atoms present in the molecule. For example, the formula for carbon dioxide (CO₂) indicates that one carbon atom is bonded to two oxygen atoms. This precise ratio is a hallmark of compounds; it ensures that the chemical and physical properties of the compound remain consistent regardless of its source or method of preparation. The law of definite proportions, also known as the law of constant composition, states that a chemical compound always contains its component elements in fixed ratio by mass, reinforcing the idea that compounds have a specific and unchanging composition. In contrast to mixtures, where components can be present in varying proportions and can often be separated by physical means, compounds cannot be separated into their constituent elements without breaking the chemical bonds that hold them together. This requires chemical reactions such as decomposition or synthesis. For example, water can be decomposed into hydrogen and oxygen through electrolysis, but this process involves breaking the covalent bonds between the hydrogen and oxygen atoms. Understanding the difference between elements and compounds is crucial for various scientific and practical applications. In chemistry, recognizing whether a substance is an element or a compound helps in predicting its behavior under different conditions and in designing experiments to synthesize new materials. In everyday life, this distinction is important for understanding the properties of substances we encounter, from the air we breathe (a mixture primarily composed of nitrogen and oxygen) to the food we eat (which often contains compounds like carbohydrates and proteins). In summary, compounds are unique substances formed by the chemical bonding of different elements in fixed ratios. Their properties are distinct from those of their constituent elements, and their composition is defined by their chemical formula. This fundamental concept underpins much of chemistry and has significant implications for both scientific research and practical applications.
Chemical Bonds in Compounds
Chemical bonds are the fundamental forces that hold atoms together to form compounds, distinguishing them from elements. These bonds arise due to the interactions between electrons and nuclei of different atoms, leading to stable molecular structures. In a compound, chemical bonds are formed when atoms share or exchange electrons to achieve a more stable electronic configuration, often resulting in a full outer energy level. There are primarily three types of chemical bonds: ionic, covalent, and metallic. Ionic bonds form when one or more electrons are transferred from one atom to another, resulting in the formation of ions with opposite charges that attract each other. This type of bonding is common in compounds composed of metals and nonmetals, such as sodium chloride (NaCl), where sodium loses an electron to become a positively charged ion (cation), and chlorine gains an electron to become a negatively charged ion (anion). The electrostatic attraction between these oppositely charged ions holds them together. Covalent bonds, on the other hand, involve the sharing of electron pairs between atoms. This type of bonding is prevalent in molecules composed of nonmetals, such as oxygen (O₂) and methane (CH₄). In covalent bonds, atoms share one or more pairs of electrons to achieve a stable octet configuration, which enhances their stability. The strength and nature of covalent bonds can vary significantly depending on the atoms involved and the number of electron pairs shared. Metallic bonds occur in metals and involve the delocalization of electrons among a lattice of metal ions. In this scenario, electrons are not localized between specific atoms but are free to move throughout the metal lattice, creating a "sea" of electrons that holds the metal ions together. This delocalization of electrons is responsible for the high electrical conductivity and malleability of metals. The formation of chemical bonds is crucial for understanding the composition and properties of compounds. Unlike elements, which consist of single types of atoms, compounds are made up of different elements chemically bonded together. The specific arrangement and type of chemical bonds in a compound determine its physical and chemical properties, such as melting point, boiling point, solubility, and reactivity. For instance, water (H₂O) is a compound formed by covalent bonds between hydrogen and oxygen atoms, giving it unique properties that are essential for life. In summary, chemical bonds are the essence of compound formation, enabling atoms to combine in specific ways to create new substances with distinct properties. Understanding these bonds is vital for appreciating the differences between elements and compounds and for predicting the behavior of various substances in different environments.
Properties and Characteristics
In the realm of chemistry, understanding the properties and characteristics of elements and compounds is fundamental to grasping the intricate world of matter. This article delves into the unique properties of elements, which define their individual behaviors and interactions. It also explores how these properties change when elements combine to form compounds, highlighting the distinct characteristics that emerge from these chemical unions. Additionally, we will examine the physical and chemical differences that distinguish elements from compounds, providing a comprehensive view of their diverse attributes. By understanding these concepts, we can transition seamlessly into a deeper exploration of the definition and composition of substances, enabling a more nuanced appreciation of the chemical landscape. This journey through the properties and characteristics of elements and compounds will equip readers with a solid foundation for further chemical inquiry.
Unique Properties of Elements
Elements, the building blocks of matter, exhibit a wide range of unique properties that distinguish them from one another and from compounds. These properties are intrinsic to each element and are determined by the arrangement of electrons within their atoms. One of the most fundamental properties is atomic number, which is the number of protons in an atom's nucleus and defines the element's position in the periodic table. This unique identifier allows elements to be classified and understood in terms of their chemical behavior. Another critical property is atomic mass, which includes both protons and neutrons in the nucleus, influencing physical characteristics such as density and melting points. For instance, carbon has an atomic mass of approximately 12 units due to its six protons and six neutrons, while oxygen has an atomic mass of about 16 units with eight protons and eight neutrons. These differences in atomic mass contribute to the diverse physical states and reactivity of elements. Electron configuration is another key property that dictates how elements interact with other atoms. The arrangement of electrons in energy levels or shells determines an element's valency (the number of electrons it can gain, lose, or share) and its position in the periodic table. For example, elements in Group 1 (alkali metals) have one electron in their outermost shell, making them highly reactive as they readily lose this electron to form a positive ion. In contrast, elements in Group 17 (halogens) have seven electrons in their outermost shell, making them highly reactive as they seek to gain one electron to achieve a stable noble gas configuration. Chemical reactivity is also a unique property of elements. Some elements are highly reactive due to their electron configuration, such as fluorine and sodium, which readily form compounds with other elements. Others are less reactive, like noble gases (Group 18), which have full outer energy levels and thus do not easily form compounds. Physical properties such as melting and boiling points, conductivity, and malleability also vary significantly among elements. For instance, mercury is a liquid at room temperature due to its weak intermolecular forces, whereas tungsten has one of the highest melting points due to its strong metallic bonds. Conductivity varies widely; metals like copper are excellent conductors because their electrons are free to move, while nonmetals like carbon in its diamond form are poor conductors. In summary, the unique properties of elements—ranging from atomic number and mass to electron configuration and chemical reactivity—make each element distinct and essential for understanding their roles in forming compounds and participating in chemical reactions. These properties are fundamental to chemistry and underpin our understanding of the periodic table and the behavior of matter at its most basic level.
Combined Properties in Compounds
When elements combine to form compounds, the resulting substances exhibit properties that are distinct from those of their individual components. This phenomenon is known as combined properties in compounds. Unlike elements, which retain their inherent characteristics, compounds display new and often unique attributes that arise from the chemical bonds between the constituent elements. For instance, sodium (Na) and chlorine (Cl) are highly reactive elements when isolated, but when they combine to form sodium chloride (NaCl), or common table salt, the resulting compound is stable and non-reactive. This transformation highlights how the properties of a compound can differ significantly from those of its constituent elements. Another example is water (H₂O), which is composed of hydrogen (H) and oxygen (O). Hydrogen is highly flammable and oxygen supports combustion, yet water extinguishes fires rather than igniting them. This stark contrast underscores the principle that compounds can exhibit entirely new physical and chemical properties compared to their elemental counterparts. The molecular structure of a compound, including the type and arrangement of chemical bonds, plays a crucial role in determining these combined properties. For example, the polarity of water molecules allows them to form hydrogen bonds, which contribute to its high boiling point and surface tension—properties that are essential for life on Earth. The combined properties of compounds also extend to their reactivity. Compounds often react differently with other substances compared to their individual elements. Carbon dioxide (CO₂), for instance, is a stable gas that can be dissolved in water to form carbonic acid, whereas elemental carbon and oxygen do not react in this manner. This reactivity is influenced by the electronic configuration and bonding patterns within the compound, which can lead to novel chemical behaviors. In addition, the physical properties of compounds such as melting and boiling points, solubility, and density are typically different from those of their constituent elements. For example, methane (CH₄) has a much lower boiling point than either carbon or hydrogen alone. These differences are critical in various industrial and biological processes, where the specific properties of compounds are harnessed for practical applications. Understanding combined properties in compounds is essential for appreciating the diversity and complexity of chemical substances. It underscores the fundamental principle that the whole is more than the sum of its parts, as the interactions between elements give rise to new and unique characteristics that define the compound's behavior and utility. This concept is central to fields such as chemistry, materials science, and biology, where the manipulation and application of compounds rely on their distinct properties.
Physical and Chemical Differences
When delving into the distinctions between elements and compounds, it is crucial to understand the physical and chemical differences that set them apart. Elements are pure substances composed of only one type of atom, each with its unique atomic number. This uniformity in atomic composition results in consistent physical properties such as melting and boiling points, density, and conductivity. For instance, pure gold (an element) has a specific melting point of 1,064°C and a density of 19.3 g/cm³, which remain constant regardless of its form or location. In contrast, compounds are formed when two or more different elements chemically bond together in fixed ratios. This combination leads to new substances with distinct physical properties that differ from those of their constituent elements. For example, water (a compound made of hydrogen and oxygen) has a melting point of 0°C and a boiling point of 100°C at standard pressure, which are vastly different from the properties of hydrogen and oxygen gases. Chemically, elements exhibit characteristic reactivity based on their electron configuration and position in the periodic table. Elements like sodium and potassium are highly reactive due to their single electron in the outermost shell, making them prone to losing electrons to form positive ions. On the other hand, compounds exhibit reactivity based on the interactions between their constituent atoms. The chemical bonds within a compound determine its stability and reactivity. For instance, carbon dioxide (a compound of carbon and oxygen) is relatively stable under normal conditions but can react with water to form carbonic acid, illustrating how the chemical properties of compounds arise from the interactions of their component elements. Furthermore, the formation and decomposition of compounds involve chemical reactions that alter the arrangement of atoms without changing their identities. This is evident in the combustion of methane (a compound of carbon and hydrogen), where methane reacts with oxygen to produce carbon dioxide and water, releasing energy in the process. In contrast, elements cannot be broken down into simpler substances by chemical means; they can only be transformed into other elements through nuclear reactions. Understanding these physical and chemical differences is essential for appreciating the fundamental distinctions between elements and compounds. While elements are characterized by their uniform atomic composition and consistent properties, compounds are defined by their unique combinations of atoms and the resultant properties that emerge from these interactions. This knowledge forms the basis for various scientific disciplines, including chemistry and materials science, where the manipulation of elements and compounds is crucial for advancing technological and industrial applications.
Formation and Examples
In the vast and intricate world of chemistry, understanding the formation and examples of elements and compounds is crucial for grasping the fundamental principles that govern our universe. This article delves into the processes behind the creation of these chemical entities, providing a comprehensive overview that bridges theoretical concepts with real-world applications. We begin by exploring the **Formation of Elements**, examining how these basic building blocks of matter come into existence through various natural and synthetic methods. Next, we delve into the **Formation of Compounds**, discussing how elements combine to form new substances with distinct properties. Finally, we illustrate these concepts with **Real-World Examples of Elements and Compounds**, highlighting their significance in everyday life and industrial processes. By understanding these foundational aspects, readers will gain a deeper appreciation for the intricate dance between elements and compounds, setting the stage for a more nuanced exploration of their **Definition and Composition**.
Formation of Elements
The formation of elements is a fundamental process that underpins the very fabric of our universe. Elements are the simplest substances in chemistry, consisting of atoms with the same number of protons in their atomic nuclei. The creation of these elements can be traced back to the earliest moments of the universe, particularly during the Big Bang and subsequent stellar nucleosynthesis. During the Big Bang, approximately 13.8 billion years ago, the universe was a hot, dense plasma where protons, neutrons, and electrons were formed. As the universe expanded and cooled, these particles began to combine into the lightest elements: hydrogen (H), helium (He), and trace amounts of lithium (Li) and beryllium (Be). This period, known as Big Bang nucleosynthesis, laid the groundwork for the elemental composition of the cosmos. However, heavier elements were not formed during this initial phase due to the universe's rapid expansion and cooling. Instead, they were created within the hearts of stars through a process called stellar nucleosynthesis. Stars fuse lighter elements into heavier ones through nuclear reactions, releasing energy in the form of light and heat. For instance, hydrogen nuclei fuse to form helium in the core of stars like our Sun. More massive stars can fuse helium into carbon (C), nitrogen (N), oxygen (O), and even heavier elements up to iron (Fe) through various nuclear reactions. When these massive stars exhaust their fuel and explode as supernovae, they scatter these newly formed elements into space. These elements then become part of new stars, planets, and other celestial bodies. Additionally, certain heavy elements beyond iron are produced during these supernovae explosions through rapid neutron capture processes known as r-process nucleosynthesis. On Earth, elements can also be formed through artificial means in particle accelerators and nuclear reactors. For example, scientists have synthesized elements like technetium (Tc) and promethium (Pm) by bombarding existing elements with high-energy particles. Understanding the formation of elements is crucial for grasping how our universe evolved from simple hydrogen and helium to the diverse array of substances we see today. This knowledge also underscores the dynamic nature of matter, highlighting how elements are continuously being created and transformed across cosmic scales. In contrast to compounds, which are formed by chemical bonding between different elements, the formation of elements themselves involves nuclear processes that alter the atomic nucleus itself—a distinction that underscores the fundamental difference between these two chemical concepts.
Formation of Compounds
The formation of compounds is a fundamental process in chemistry that involves the combination of two or more different elements to create a new substance with distinct properties. This process is governed by the principles of chemical bonding, where atoms share or exchange electrons to achieve a stable electronic configuration. Compounds can be formed through various types of chemical bonds, including ionic, covalent, and metallic bonds. **Ionic Bonds:** These bonds are formed when one or more electrons are transferred from one atom to another, resulting in the formation of ions with opposite charges. The electrostatic attraction between these oppositely charged ions holds them together, forming an ionic compound. For example, sodium (Na) and chlorine (Cl) combine to form sodium chloride (NaCl), commonly known as table salt. In this reaction, sodium loses an electron to become a positively charged ion (Na⁺), while chlorine gains an electron to become a negatively charged ion (Cl⁻). The strong electrostatic attraction between these ions results in the formation of a stable ionic compound. **Covalent Bonds:** These bonds are formed when atoms share one or more pairs of electrons to achieve a stable electronic configuration. This type of bonding is prevalent in molecules where the atoms involved are nonmetals. For instance, hydrogen (H) and oxygen (O) combine to form water (H₂O). In this molecule, each hydrogen atom shares its single electron with the oxygen atom, which has six valence electrons. The sharing of these electrons results in a covalent bond that holds the hydrogen and oxygen atoms together. **Metallic Bonds:** These bonds are characteristic of metals and involve the delocalization of electrons among a lattice of metal ions. In metallic compounds, the electrons are not localized between specific atoms but are free to move throughout the metal lattice. This delocalization of electrons is responsible for the high electrical conductivity and malleability of metals. The formation of compounds can also be influenced by factors such as the reactivity of the elements involved and the conditions under which the reaction occurs. For example, the reaction between hydrogen gas (H₂) and oxygen gas (O₂) to form water requires an ignition source to initiate the combustion process. Understanding how compounds are formed is crucial for various fields such as chemistry, materials science, and pharmaceuticals, as it allows scientists to synthesize new materials with specific properties and applications. In summary, the formation of compounds is a complex yet fascinating process that underpins much of modern chemistry. By understanding the different types of chemical bonds and the conditions necessary for their formation, scientists can create a wide range of compounds with diverse properties, from simple salts like sodium chloride to complex molecules like DNA. This knowledge not only enhances our understanding of the natural world but also enables the development of new technologies and materials that improve our daily lives.
Real-World Examples of Elements and Compounds
In the real world, elements and compounds are ubiquitous and play crucial roles in various aspects of our lives. Elements, which are substances consisting of only one type of atom, can be found in their pure form or combined with other elements to form compounds. For instance, **oxygen (O₂)** is an essential element for human respiration. It is a diatomic molecule, meaning it exists naturally as two oxygen atoms bonded together. In contrast, **water (H₂O)** is a compound composed of two hydrogen atoms and one oxygen atom. This simple compound is vital for all known forms of life and is used in countless industrial processes. Another example is **carbon (C)**, an element that forms the basis of all organic life. Carbon can exist in various forms such as graphite, diamond, and fullerenes, each with unique properties. When carbon combines with oxygen, it forms **carbon dioxide (CO₂)**, a compound that plays a significant role in the Earth's climate system and is a byproduct of combustion processes. **Iron (Fe)** is another element that is widely used in construction and manufacturing due to its strength and durability. When iron reacts with oxygen in the presence of moisture, it forms **iron oxide (rust)**, a compound that can weaken the structural integrity of iron-based materials. Understanding the difference between elements and compounds is crucial for developing strategies to prevent rust formation and extend the lifespan of iron structures. In agriculture, **nitrogen (N₂)** is an essential element for plant growth. However, nitrogen in its elemental form is not readily available to plants. Instead, plants absorb nitrogen through compounds like **ammonia (NH₃)** and **nitrate (NO₃⁻)**, which are formed when nitrogen reacts with other elements such as hydrogen and oxygen. The distinction between elements and compounds also has significant implications in medicine. For example, **sodium (Na)** is an element that is crucial for maintaining proper fluid balance in the human body. However, when sodium combines with chlorine to form **sodium chloride (NaCl or table salt)**, it becomes a compound that is essential for various bodily functions but can be harmful in excessive amounts. These real-world examples illustrate how elements and compounds are intertwined in our daily lives, from the air we breathe to the materials we use and the food we eat. Understanding their formation and properties is fundamental to advancing various fields such as chemistry, biology, engineering, and medicine.