What Is Fabrication
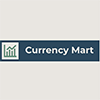
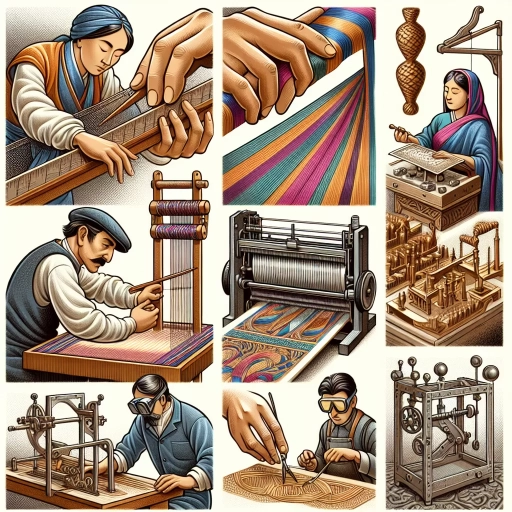
Fabrication, a cornerstone of modern manufacturing, is the process of creating products from raw materials through various techniques and technologies. This multifaceted field encompasses a wide range of activities, from simple assembly to complex engineering processes. At its core, fabrication involves transforming raw materials into finished goods, which are essential for numerous industries including aerospace, automotive, construction, and electronics. To fully grasp the significance of fabrication, it is crucial to delve into its fundamental principles, explore the diverse types and methods employed, and understand its far-reaching applications and impact. In this article, we will begin by **Understanding the Basics of Fabrication**, laying the groundwork for a comprehensive exploration of this critical manufacturing process. This foundational knowledge will then be expanded upon by examining **Types and Methods of Fabrication**, highlighting the various techniques and technologies that drive this industry. Finally, we will discuss **Applications and Impact of Fabrication**, revealing how this process shapes our world and drives innovation across multiple sectors. By exploring these key aspects, readers will gain a thorough understanding of the role fabrication plays in modern society.
Understanding the Basics of Fabrication
Understanding the basics of fabrication is a multifaceted journey that encompasses various dimensions, each crucial for a comprehensive grasp of the subject. To delve into this complex field, it is essential to start with a clear **Definition and Scope**, which outlines what fabrication entails and its boundaries within different industries. This foundational knowledge sets the stage for exploring the **Historical Context**, where we can trace the evolution of fabrication techniques from ancient civilizations to modern-day advancements. Additionally, familiarizing oneself with **Key Terminology** is vital, as it provides the vocabulary necessary to communicate effectively and understand the intricacies involved in the fabrication process. By beginning with a solid definition and scope, we can then seamlessly transition into the historical context and key terminology, ensuring a well-rounded understanding of fabrication's core principles. This structured approach will enable readers to appreciate the depth and breadth of fabrication, making it an engaging and informative exploration. Let us start by defining what fabrication is and its scope within various sectors.
Definition and Scope
**Definition and Scope** Fabrication, a cornerstone of modern manufacturing, is the process of constructing or assembling components into a finished product through various techniques and technologies. At its core, fabrication involves transforming raw materials such as metals, plastics, and composites into functional items by cutting, shaping, forming, and joining them. This multifaceted discipline encompasses a wide range of activities including machining, welding, casting, forging, and 3D printing. The scope of fabrication is vast and diverse, extending across multiple industries such as aerospace, automotive, construction, electronics, and healthcare. In the aerospace sector, fabrication plays a critical role in producing lightweight yet robust components for aircraft and spacecraft. Here, advanced materials like titanium alloys and carbon fiber composites are precision-crafted using sophisticated machining tools and specialized welding techniques. Similarly, in the automotive industry, fabrication is essential for manufacturing vehicle parts such as engine blocks, chassis components, and body panels. These parts are often produced through high-volume processes like stamping and casting to meet stringent quality and safety standards. The construction industry also heavily relies on fabrication for creating structural elements like beams, columns, and piping systems. Fabrication shops use large-scale machinery to cut, bend, and assemble these components according to precise specifications. In electronics manufacturing, fabrication involves the production of circuit boards and semiconductor devices through intricate processes such as etching and doping. Even in healthcare, medical devices like implants and surgical instruments are fabricated using biocompatible materials and advanced machining techniques. The advent of digital technologies has further expanded the scope of fabrication by introducing new methods such as additive manufacturing (3D printing) and computer-aided design (CAD). These tools enable rapid prototyping, increased precision, and reduced material waste. Additionally, automation in fabrication has improved efficiency by integrating robots and CNC machines into production lines. Understanding the basics of fabrication is crucial for anyone involved in product development or manufacturing. It requires a deep appreciation for material properties, manufacturing processes, and quality control measures. By grasping these fundamentals, individuals can better design products that are both functional and cost-effective while ensuring they meet industry standards for safety and performance. As technology continues to evolve, the field of fabrication remains dynamic and innovative, offering endless possibilities for creating complex products with unprecedented precision and speed.
Historical Context
Understanding the historical context of fabrication is crucial for appreciating its evolution and significance in modern manufacturing. Fabrication, the process of constructing or assembling components into a finished product, has roots that stretch back thousands of years. In ancient civilizations, such as Egypt, Greece, and Rome, artisans and craftsmen employed various techniques to fabricate tools, weapons, and architectural structures. For instance, the Egyptians developed sophisticated methods for metalworking and woodworking, evident in the intricate designs found in their tombs and temples. Similarly, ancient Greeks were renowned for their skill in forging metals and crafting marble sculptures. The Industrial Revolution marked a pivotal moment in the history of fabrication. With the advent of mechanized tools and mass production techniques, fabrication transitioned from a labor-intensive craft to an industrial process. Innovations like the steam engine and interchangeable parts enabled factories to produce goods on a larger scale and with greater precision. This period saw the rise of new materials such as steel and aluminum, which further expanded the possibilities for fabrication. In the 20th century, advancements in technology continued to transform fabrication practices. The introduction of computer-aided design (CAD) software and computer numerical control (CNC) machines revolutionized the industry by allowing for precise and rapid production. This era also witnessed the development of new materials like plastics and composites, which offered unique properties that could be leveraged in various applications. Today, fabrication is a highly sophisticated field that incorporates cutting-edge technologies such as 3D printing, laser cutting, and robotic assembly. These advancements have not only improved efficiency but also enabled the creation of complex geometries and customized products that were previously impossible to produce. Additionally, the integration of artificial intelligence and machine learning into fabrication processes is enhancing quality control and predictive maintenance. Understanding this historical context is essential for grasping the basics of fabrication because it highlights how technological innovations have continuously shaped and refined the process. By recognizing the milestones and challenges faced by past generations of fabricators, modern practitioners can better appreciate the tools and techniques available to them today. This historical perspective also underscores the importance of ongoing innovation and adaptation in meeting future manufacturing demands. As fabrication continues to evolve with emerging technologies, its rich history serves as a foundation upon which new breakthroughs are built.
Key Terminology
**Understanding the Basics of Fabrication: Key Terminology** To delve into the world of fabrication, it is crucial to grasp the fundamental terminology that underpins this complex process. Fabrication, at its core, involves the creation of products through various manufacturing processes, and understanding the key terms can significantly enhance your comprehension and engagement with the subject. **1. ** **Material Selection:** This refers to the choice of raw materials used in fabrication, such as metals, plastics, composites, or textiles. Each material has unique properties that influence the final product's performance, durability, and cost. **2. ** **Tooling:** Tooling encompasses the molds, dies, and other specialized equipment necessary for shaping and forming materials. High-quality tooling is essential for achieving precise dimensions and surface finishes. **3. ** **Machining:** Machining involves using machine tools to remove material from a workpiece to achieve the desired shape and size. Common machining processes include turning, milling, drilling, and grinding. **4. ** **Welding:** Welding is a fabrication technique that joins two or more pieces of material together by applying heat, pressure, or both. Common welding methods include MIG (Metal Inert Gas), TIG (Tungsten Inert Gas), and Arc welding. **5. ** **Cutting:** Cutting processes, such as laser cutting, water jet cutting, and CNC (Computer Numerical Control) machining, are used to separate materials into desired shapes and sizes. **6. ** **Assembly:** Assembly involves bringing together various fabricated components to form the final product. This can include fastening techniques like riveting, bolting, or adhesive bonding. **7. ** **Quality Control:** Quality control measures ensure that fabricated products meet specified standards and requirements. This includes inspections, testing, and certification processes. **8. ** **CAD/CAM:** Computer-Aided Design (CAD) and Computer-Aided Manufacturing (CAM) software are integral tools in modern fabrication. CAD software allows designers to create detailed digital models, while CAM software translates these designs into instructions for CNC machines. **9. ** **Prototyping:** Prototyping involves creating a preliminary version of a product to test its functionality, aesthetics, and performance before full-scale production begins. **10.** **Lean Manufacturing:** Lean manufacturing principles aim to minimize waste and maximize efficiency in the fabrication process by optimizing workflows, reducing inventory, and enhancing continuous improvement. By familiarizing yourself with these key terms, you gain a solid foundation for understanding the intricacies of fabrication. Whether you are involved in engineering, manufacturing, or simply curious about how products are made, mastering this terminology will help you navigate the complex landscape of fabrication with confidence and clarity. Understanding these concepts not only enhances your technical knowledge but also allows you to appreciate the meticulous craftsmanship and technological advancements that go into creating everything from simple tools to sophisticated machinery.
Types and Methods of Fabrication
Fabrication, the process of transforming raw materials into finished products, is a multifaceted field that encompasses a wide range of techniques and technologies. In this article, we delve into the diverse types and methods of fabrication, highlighting their unique characteristics and applications. We begin by exploring **Material-Specific Fabrication Techniques**, which tailor processes to the inherent properties of different materials, ensuring optimal results. Next, we examine **Advanced Fabrication Technologies**, such as 3D printing and laser cutting, which have revolutionized the industry with their precision and efficiency. Finally, we discuss **Traditional vs. Modern Fabrication Methods**, comparing the time-tested approaches with the innovative solutions of today. Each of these aspects plays a crucial role in understanding the complexities and advancements in fabrication. By understanding these different facets, manufacturers can select the most appropriate methods to produce high-quality products efficiently. Let's start by diving into the nuances of **Material-Specific Fabrication Techniques**, where the specific characteristics of materials dictate the fabrication process.
Material-Specific Fabrication Techniques
Material-specific fabrication techniques are crucial in the broader context of fabrication, as they tailor the manufacturing process to the unique properties and requirements of different materials. This approach ensures that each material is processed in a way that maximizes its inherent strengths while minimizing potential weaknesses. For instance, metal fabrication often involves techniques such as machining, casting, forging, and welding. Machining, which includes processes like milling and turning, is used to remove material and achieve precise dimensions. Casting involves pouring molten metal into a mold to create complex shapes, while forging shapes metal through high-pressure deformation. Welding, on the other hand, joins metal parts together using heat and pressure. In contrast, fabricating plastics requires different methods due to their distinct physical properties. Techniques such as injection molding, extrusion, and 3D printing are commonly used. Injection molding involves forcing molten plastic into a mold to produce high-volume parts with consistent quality. Extrusion shapes plastic into continuous profiles like pipes or sheets by pushing it through a die. 3D printing, or additive manufacturing, builds plastic parts layer by layer from digital designs, allowing for complex geometries that traditional methods cannot achieve. For composites, such as carbon fiber reinforced polymers (CFRP), specialized techniques are necessary due to their anisotropic nature and sensitivity to processing conditions. Methods include hand layup, vacuum bagging, and resin transfer molding (RTM). Hand layup involves manually placing layers of composite material in a mold before curing under pressure or heat. Vacuum bagging uses a vacuum to consolidate layers before curing in an oven or autoclave. RTM involves injecting resin into a mold containing pre-placed fibers under pressure to ensure uniform distribution. Ceramic fabrication also demands unique approaches due to the brittle nature of these materials. Techniques include slip casting, where a liquid ceramic suspension is poured into a mold and allowed to solidify before firing at high temperatures. Pressing involves compacting ceramic powders into desired shapes using mechanical or hydraulic presses before firing. 3D printing can also be adapted for ceramics by layering ceramic powders or pastes. Wood fabrication, while often less complex than other materials, still requires specific techniques tailored to its natural variability and grain structure. Methods include cutting, drilling, and joining using nails, screws, or adhesives. Advanced techniques such as CNC machining allow for precise cutting and shaping of wood components. In summary, material-specific fabrication techniques are essential for optimizing the performance and quality of final products by leveraging the unique characteristics of each material type. By understanding these specialized methods, manufacturers can produce components that meet stringent requirements across various industries ranging from aerospace to consumer goods. This tailored approach not only enhances product reliability but also reduces waste and improves overall efficiency in the fabrication process.
Advanced Fabrication Technologies
Advanced fabrication technologies represent a significant leap forward in the field of manufacturing, enabling the creation of complex structures and materials with unprecedented precision and efficiency. These technologies encompass a range of innovative methods that have transformed traditional fabrication processes, allowing for greater customization, reduced production times, and enhanced product quality. One of the most prominent advanced fabrication technologies is 3D printing, also known as additive manufacturing. This process involves layering materials such as metals, plastics, and ceramics to build objects from digital designs. Unlike traditional subtractive manufacturing methods, 3D printing minimizes material waste and can produce intricate geometries that would be impossible to achieve with conventional techniques. Another key area is nanofabrication, which involves the manipulation of matter at the nanoscale to create materials with unique properties. This field leverages techniques such as lithography and etching to fabricate nanostructures that can be used in a variety of applications, including electronics, biomedical devices, and energy storage systems. Additionally, advanced machining technologies like laser cutting and water jet cutting offer high precision and versatility in fabricating complex shapes from a wide range of materials. Robotics and automation also play a crucial role in modern fabrication. Robotic systems equipped with advanced sensors and AI algorithms can perform tasks that require high precision and repeatability, such as welding, assembly, and inspection. These systems not only improve productivity but also enhance safety by reducing human exposure to hazardous environments. Furthermore, advanced materials science has led to the development of smart materials and composites that can be fabricated using specialized techniques. For instance, carbon fiber reinforced polymers (CFRP) are fabricated using processes like resin transfer molding (RTM) or vacuum-assisted resin transfer molding (VARTM), resulting in lightweight yet incredibly strong materials used in aerospace and automotive industries. The integration of digital technologies such as computer-aided design (CAD), computer-aided manufacturing (CAM), and the Internet of Things (IoT) has further revolutionized fabrication processes. These tools enable real-time monitoring, predictive maintenance, and data-driven decision-making, significantly improving the efficiency and reliability of fabrication operations. In summary, advanced fabrication technologies are redefining the boundaries of what is possible in manufacturing. By combining cutting-edge techniques with innovative materials and digital tools, these technologies are driving innovation across various industries, from aerospace to healthcare. As these technologies continue to evolve, they promise even greater advancements in precision, speed, and sustainability, shaping the future of fabrication in profound ways.
Traditional vs. Modern Fabrication Methods
In the realm of fabrication, the dichotomy between traditional and modern methods is stark yet fascinating. Traditional fabrication techniques, which have been honed over centuries, rely on manual skills and time-tested processes. These methods often involve craftsmanship that is both labor-intensive and highly personalized. For instance, blacksmithing, woodworking, and hand-welding are examples where artisans use tools and techniques passed down through generations to create unique pieces. These traditional methods not only preserve cultural heritage but also ensure a level of quality and detail that is hard to replicate with modern machinery. However, they are generally slower and more expensive due to the manual labor involved. On the other hand, modern fabrication methods leverage cutting-edge technology to streamline production processes. Computer-aided design (CAD) software allows for precise digital modeling before any physical work begins, reducing errors and enhancing efficiency. Advanced machinery such as CNC (Computer Numerical Control) machines, 3D printers, and laser cutters can execute complex designs with high accuracy and speed. These tools enable mass production while maintaining consistency and quality across batches. Additionally, modern fabrication often incorporates automation, reducing labor costs and increasing productivity. For example, robotic welding systems can perform repetitive tasks with precision, making them ideal for large-scale industrial applications. Despite their differences, both traditional and modern fabrication methods have their own merits and applications. Traditional techniques are often preferred for bespoke or heritage projects where the human touch is invaluable. In contrast, modern methods are better suited for high-volume production where speed and consistency are paramount. The integration of both approaches can also yield innovative results; for instance, combining traditional craftsmanship with modern tools can enhance precision while retaining the unique qualities of handmade products. The choice between traditional and modern fabrication ultimately depends on the specific needs of a project—whether it requires the personal touch of an artisan or the efficiency of advanced technology. As technology continues to evolve, it is likely that we will see further hybridization of these methods, allowing for even more versatile and effective fabrication processes in various industries. Understanding these different approaches not only appreciates the historical context of fabrication but also prepares us for the future where both old and new techniques will coexist to meet diverse manufacturing needs.
Applications and Impact of Fabrication
Fabrication, the process of creating objects through various techniques such as cutting, shaping, and assembling materials, has far-reaching applications that span multiple industries. This versatile technology not only underpins modern manufacturing but also plays a crucial role in architectural and construction projects. Furthermore, its innovative applications are continually expanding into emerging fields, driving technological advancements and economic growth. In industrial and manufacturing contexts, fabrication enables the mass production of precision parts and components, enhancing efficiency and quality. Architecturally, it allows for the creation of complex structures and designs that were previously unimaginable. Additionally, emerging fields such as aerospace, biomedical engineering, and renewable energy are leveraging fabrication to push the boundaries of what is possible. This article will delve into these three key areas: Industrial and Manufacturing Uses, Architectural and Construction Applications, and Innovative and Emerging Fields, starting with the foundational role of fabrication in industrial and manufacturing processes.
Industrial and Manufacturing Uses
Industrial and manufacturing uses of fabrication are pivotal in modern production processes, driving efficiency, innovation, and quality across various sectors. Fabrication, the process of creating products from raw materials through cutting, shaping, and assembling, is a cornerstone in industries such as aerospace, automotive, construction, and consumer goods. In aerospace, for instance, fabrication techniques like CNC machining and 3D printing enable the precise creation of complex components such as engine parts and satellite components, ensuring high performance and reliability. In the automotive sector, fabrication is used to manufacture vehicle bodies, engine components, and other critical parts, leveraging technologies like laser cutting and robotic welding to enhance speed and accuracy. In construction, fabrication plays a crucial role in producing prefabricated building components such as steel beams, roofing materials, and modular housing units. This approach not only accelerates construction timelines but also improves structural integrity and reduces on-site labor costs. Consumer goods manufacturers also rely heavily on fabrication to produce a wide range of products from kitchen appliances to electronic devices. Here, techniques like sheet metal fabrication and plastic injection molding are employed to create durable and aesthetically pleasing products that meet stringent quality standards. Moreover, advancements in fabrication technologies have significantly impacted the manufacturing landscape. The integration of Industry 4.0 technologies such as artificial intelligence, IoT sensors, and data analytics has transformed traditional fabrication processes into smart manufacturing systems. These systems optimize production workflows, predict maintenance needs, and ensure real-time quality control, thereby enhancing productivity and reducing waste. Additionally, sustainable fabrication practices are gaining traction as manufacturers adopt eco-friendly materials and energy-efficient processes to minimize environmental footprint. The impact of fabrication extends beyond the production floor; it influences product design, supply chain management, and customer satisfaction. Modern fabrication techniques allow for rapid prototyping and iterative design improvements, enabling companies to bring innovative products to market faster. Furthermore, the precision and consistency offered by advanced fabrication methods ensure that final products meet or exceed customer expectations, fostering brand loyalty and market competitiveness. In conclusion, the industrial and manufacturing uses of fabrication are multifaceted and indispensable. By leveraging cutting-edge technologies and sustainable practices, industries can achieve higher levels of efficiency, quality, and innovation. As fabrication continues to evolve with technological advancements, its role in shaping the future of manufacturing will only become more pronounced, driving economic growth and societal progress.
Architectural and Construction Applications
In the realm of architectural and construction applications, fabrication plays a pivotal role in transforming design concepts into tangible structures. This process involves the precise creation of building components, such as steel beams, glass panels, and prefabricated modules, which are then assembled on-site. Fabrication enhances the efficiency and accuracy of construction projects by allowing for the production of complex elements in controlled environments. For instance, modular construction, a subset of fabrication, enables the assembly of entire rooms or floors in a factory before being transported to the building site. This approach reduces on-site labor, minimizes waste, and accelerates project timelines. The use of advanced fabrication techniques also allows for greater design flexibility and innovation. Architects can now conceptualize and execute intricate designs that would be impractical or impossible to achieve through traditional construction methods. For example, 3D printing technology is being increasingly used to fabricate complex architectural elements such as walls, roofs, and even entire buildings. This method not only speeds up the construction process but also offers unprecedented opportunities for customization and sustainability. Moreover, fabrication contributes significantly to the sustainability of construction projects. By manufacturing components in a factory setting, fabricators can optimize material usage, reduce waste, and implement more stringent quality control measures. This results in structures that are not only aesthetically pleasing but also environmentally friendly. Additionally, prefabricated components can be designed with energy efficiency in mind, incorporating features such as insulation and renewable energy systems that reduce the building's carbon footprint over its lifespan. From a structural integrity perspective, fabricated components offer superior strength and durability compared to those constructed on-site. The controlled environment of a fabrication facility ensures that each component meets precise specifications and undergoes rigorous testing before being integrated into the final structure. This attention to detail is particularly crucial in high-rise buildings, bridges, and other critical infrastructure where safety is paramount. In conclusion, the applications of fabrication in architecture and construction are multifaceted and transformative. By leveraging advanced technologies and manufacturing techniques, fabricators can deliver projects that are faster, greener, more innovative, and safer. As the industry continues to evolve, the role of fabrication will only become more integral to the creation of modern buildings and infrastructure, driving innovation and excellence in every aspect of construction.
Innovative and Emerging Fields
In the realm of fabrication, innovative and emerging fields are revolutionizing the way we design, produce, and interact with materials. One of the most significant areas is **Additive Manufacturing (3D Printing)**, which allows for the creation of complex geometries and customized products with unprecedented precision. This technology has transformed industries such as aerospace, healthcare, and automotive by enabling rapid prototyping, reducing material waste, and producing lightweight yet robust structures. For instance, in aerospace engineering, 3D printing is used to fabricate intricate components like engine parts and satellite components that cannot be produced through traditional methods. Another burgeoning field is **Nanofabrication**, which involves the manipulation of materials at the nanoscale to create novel materials with unique properties. This area has seen significant advancements in fields like electronics, energy storage, and biomedical engineering. Nanofabricated materials are being used to develop more efficient solar cells, advanced drug delivery systems, and ultra-sensitive biosensors. The ability to control material properties at such a fine scale opens up new avenues for innovation in various sectors. **Soft Robotics** is another emerging domain that leverages fabrication techniques to create flexible robots capable of interacting with delicate environments. These robots are particularly useful in healthcare for tasks such as minimally invasive surgery and rehabilitation therapy. By fabricating soft actuators and sensors, researchers can design robots that mimic biological systems, offering a more gentle and adaptive approach to robotic interaction. The intersection of fabrication with **Artificial Intelligence (AI)** and **Machine Learning (ML)** is also yielding groundbreaking results. AI-driven fabrication systems can optimize production processes in real-time, predict material behavior, and automate quality control. This synergy enables faster production cycles, improved product quality, and reduced costs. For example, AI can be used to predict the optimal parameters for 3D printing based on the desired material properties, ensuring consistent outcomes without extensive trial and error. Furthermore, **Bioprinting** is an innovative field that combines fabrication techniques with biological sciences to create living tissues and organs. This area holds immense potential for regenerative medicine, allowing for the creation of personalized tissue models for drug testing and organ transplantation. Bioprinted tissues can mimic the structure and function of natural tissues more accurately than traditional methods, paving the way for new therapeutic approaches. Lastly, **Quantum Fabrication** is on the horizon, promising to revolutionize material science by enabling the precise control of quantum states in materials. This could lead to the development of superconductors, ultra-efficient energy storage devices, and quantum computing components. Although still in its infancy, quantum fabrication has the potential to transform various industries by unlocking new material properties that were previously unimaginable. These emerging fields in fabrication not only expand our technological capabilities but also have profound implications for societal impact. They offer solutions to pressing global challenges such as sustainable energy, healthcare accessibility, and environmental sustainability. As these technologies continue to evolve, they will undoubtedly reshape industries and improve quality of life across the globe.