What Is A Centriole
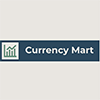
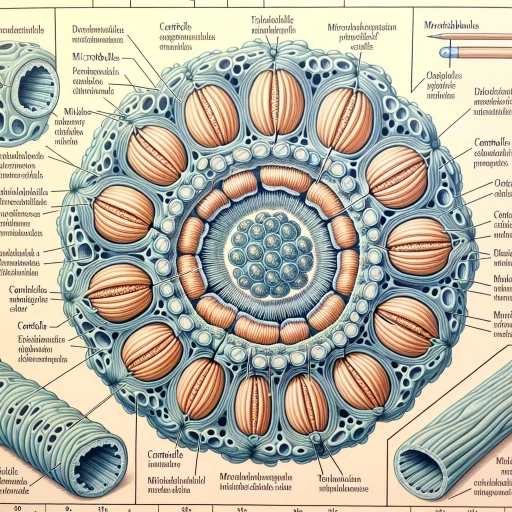
Centrioles are microscopic, cylindrical organelles that play a pivotal role in the structure and function of eukaryotic cells. These tiny structures, typically found in pairs near the nucleus, are crucial for various cellular processes. In this article, we will delve into the world of centrioles, exploring their fundamental nature, their multifaceted functions, and their significant role in cellular activities. First, we will introduce the basic characteristics and components of centrioles, providing a foundational understanding of these organelles. Next, we will examine the diverse functions of centrioles, including their involvement in the formation of cilia, flagella, and the spindle fibers during cell division. Finally, we will discuss the broader role of centrioles in cellular processes such as cell division, signaling, and the maintenance of cellular integrity. By understanding these aspects, we can appreciate the intricate mechanisms by which centrioles contribute to the health and function of cells. Let us begin by exploring the **Introduction to Centrioles**.
Introduction to Centrioles
Centrioles are microscopic, cylindrical organelles that play a crucial role in the cellular structure and function of eukaryotic cells. These tiny but vital components are involved in the formation of cilia, flagella, and the spindle fibers that separate chromosomes during cell division. To fully understand the significance of centrioles, it is essential to delve into their definition and discovery, their structural composition, and their historical significance. **Definition and Discovery** will explore how centrioles were first identified and the evolving understanding of their function over time. This section will provide a foundational knowledge of what centrioles are and how they were discovered, setting the stage for a deeper exploration of their intricate structure and historical impact. By examining the **Structural Composition** of centrioles, we can appreciate the precise arrangement of microtubules and associated proteins that enable these organelles to perform their diverse roles. This detailed look at their architecture will highlight the complexity and efficiency of centriole function. Lastly, the **Historical Significance** of centrioles will reveal how these organelles have contributed to our broader understanding of cellular biology and the development of various scientific disciplines. This historical context will underscore the importance of centrioles in advancing our knowledge of cell biology. Transitioning to the **Definition and Discovery** section, we will begin by tracing the origins of centriole research and the key milestones that have shaped our current understanding of these fascinating organelles.
Definition and Discovery
**Definition and Discovery** Centrioles, tiny cylindrical organelles found in most eukaryotic cells, play a pivotal role in the cellular structure and function. The term "centriole" was coined by Theodor Boveri and Walther Flemming in the late 19th century, derived from the Latin word "centrum," meaning center, due to their central position within the cell. These microtubule-based structures are approximately 200 nanometers in length and 250 nanometers in diameter, often arranged in pairs to form a centrosome. The discovery of centrioles is closely tied to the development of microscopy techniques. Early observations by Boveri and Flemming using light microscopy revealed these enigmatic structures, but it wasn't until the advent of electron microscopy in the mid-20th century that their detailed architecture could be fully appreciated. Electron micrographs showed that centrioles are composed of nine triplets of microtubules arranged in a radial pattern, a configuration that has become a hallmark of their structure. The definition of centrioles extends beyond their morphology; it encompasses their multifaceted roles within the cell. They are crucial for the formation of cilia, flagella, and the spindle fibers that separate chromosomes during cell division. In interphase cells, centrioles reside at the poles of the centrosome, which acts as a microtubule-organizing center (MTOC), regulating cytoskeletal dynamics and maintaining cellular polarity. During mitosis, centrioles duplicate and separate to form the spindle poles, ensuring accurate chromosome segregation. Their involvement in ciliogenesis is equally significant; centrioles serve as basal bodies from which cilia and flagella extend, facilitating sensory perception and motility in various cell types. The discovery of centrioles has been a gradual process, with each technological advancement revealing new aspects of their biology. From initial light microscopic observations to detailed electron microscopic studies and more recent live-cell imaging techniques, our understanding of centrioles has evolved significantly. Modern research continues to unravel the complex mechanisms by which centrioles function, including their role in signaling pathways and their potential involvement in disease states such as cancer and ciliopathies. This ongoing exploration underscores the importance of centrioles as key components of cellular architecture and function, making them a fascinating subject for continued scientific investigation. As we delve deeper into the intricacies of centriole biology, we gain a broader appreciation for the intricate machinery that governs cellular life.
Structural Composition
**Structural Composition** Centrioles, crucial components of the centrosome, are highly organized and complex structures that play pivotal roles in cellular processes such as cell division, cilia formation, and the maintenance of cellular polarity. Composed primarily of microtubules, centrioles are cylindrical organelles typically measuring 200 nanometers in length and 250 nanometers in diameter. Each centriole is made up of nine triplet microtubules arranged in a radial pattern, forming a characteristic "9+0" configuration. This precise arrangement is essential for the structural integrity and function of centrioles. The microtubules are stabilized by various proteins, including those belonging to the tubulin family, which form the backbone of these structures. Additionally, centrioles are surrounded by a cloud of pericentriolar material (PCM), which is rich in proteins that facilitate microtubule nucleation and anchorage. This PCM is critical for the centrosome's ability to organize the microtubule cytoskeleton, thereby influencing cellular shape, movement, and division. The structural composition of centrioles also includes specific proteins that regulate their duplication and segregation during the cell cycle, ensuring that each daughter cell receives the appropriate number of centrioles. This intricate architecture underscores the importance of centrioles in maintaining cellular homeostasis and facilitating various cellular functions, making them a fascinating subject for study in cell biology. Understanding the structural composition of centrioles provides a foundational insight into their multifaceted roles within the cell, setting the stage for a deeper exploration of their functions and significance in cellular processes.
Historical Significance
The historical significance of centrioles is deeply intertwined with the evolution of cellular biology and our understanding of cellular structure and function. The discovery of centrioles dates back to the late 19th century, a period marked by significant advancements in microscopy and cellular research. In 1888, Theodor Boveri, a German zoologist, first identified these organelles while studying the division of sea urchin eggs. Boveri's observations were pivotal as they introduced the concept that centrioles play a crucial role in the formation of the spindle apparatus during mitosis, thereby ensuring accurate chromosome segregation. As microscopy techniques improved, so did our understanding of centrioles. By the early 20th century, scientists like E.B. Wilson and E.G. Conklin had further elucidated the role of centrioles in cell division, highlighting their involvement in the organization of microtubules and the formation of cilia and flagella. The development of electron microscopy in the mid-20th century allowed for even more detailed studies, revealing the intricate structure of centrioles and their relationship with other cellular components. The historical significance of centrioles extends beyond their role in cell division; they have also been central to debates about cellular evolution and the origins of eukaryotic cells. The presence of centrioles in eukaryotic cells but not in prokaryotic cells has led to hypotheses about the evolutionary divergence of these cellular types. Moreover, research on centrioles has contributed to our understanding of developmental biology, particularly in the context of ciliogenesis and the formation of the primary cilium, which is crucial for signaling pathways in embryonic development. In recent decades, the study of centrioles has become increasingly interdisciplinary, integrating insights from molecular biology, genetics, and biophysics. Advances in live-cell imaging and super-resolution microscopy have enabled real-time observations of centriole dynamics, providing new insights into their assembly, duplication, and function. This ongoing research has not only deepened our understanding of cellular processes but also opened avenues for exploring the implications of centriole dysfunction in human diseases, such as cancer and ciliopathies. In summary, the historical significance of centrioles lies in their discovery and subsequent study, which have been instrumental in shaping our current understanding of cellular biology. From their initial identification to the present day, research on centrioles has been a cornerstone of scientific progress, influencing fields ranging from developmental biology to disease pathology. As we continue to unravel the complexities of cellular life, the study of centrioles remains a vibrant and essential area of inquiry.
Functions of Centrioles
Centrioles are dynamic, microtubule-based organelles that play pivotal roles in various cellular processes, making them essential for the proper functioning of eukaryotic cells. This article delves into the multifaceted functions of centrioles, highlighting their critical involvement in three key areas: cilia and flagella formation, centrosome organization, and cell division and cytokinesis. Firstly, centrioles are instrumental in the formation of cilia and flagella, which are vital for cellular motility and sensory functions. These structures are composed of microtubules that originate from the centrioles, enabling cells to move through fluids or detect environmental signals. Secondly, centrioles serve as the core components of centrosomes, which are crucial for organizing the microtubule cytoskeleton and ensuring proper spindle formation during cell division. Lastly, their role in cell division and cytokinesis is indispensable, as they help in the formation of the mitotic spindle and the subsequent separation of chromosomes into daughter cells. Understanding these functions not only sheds light on the intricate mechanisms of cellular biology but also underscores the importance of centrioles in maintaining cellular integrity and function. This article will explore each of these roles in detail, starting with the critical process of cilia and flagella formation, which underscores the dynamic and versatile nature of centrioles in cellular biology.
Cilia and Flagella Formation
**Cilia and Flagella Formation** Centrioles play a crucial role in the formation of cilia and flagella, which are essential for cellular motility and sensory functions. These microtubule-based structures are vital for various biological processes, including locomotion, feeding, and sensory perception in eukaryotic cells. The process of cilia and flagella formation begins with the centrioles, which serve as basal bodies. Here’s how it unfolds: 1. **Centriole Duplication**: During the cell cycle, specifically in the G1 phase, centrioles duplicate to form two pairs. This duplication is essential for the subsequent formation of cilia and flagella. 2. **Basal Body Formation**: One pair of centrioles migrates to the cell surface where they become basal bodies. These basal bodies are oriented perpendicular to the cell membrane and act as templates for microtubule assembly. 3. **Microtubule Assembly**: The basal bodies nucleate microtubules that extend from the basal plate towards the cell surface. These microtubules are arranged in a specific pattern; for motile cilia and flagella, they typically form a 9+2 arrangement, where nine doublet microtubules surround a central pair. 4. **Ciliary/Flagellar Membrane**: As the microtubules grow, they become enveloped by a plasma membrane extension, forming the ciliary or flagellar membrane. This membrane is continuous with the cell membrane but can have distinct lipid and protein compositions. 5. **Axoneme Formation**: The axoneme, which is the core structure of cilia and flagella, consists of the microtubules and associated proteins such as dynein arms and radial spokes. These components are crucial for generating the bending motion necessary for motility. 6. **Maturation**: Once formed, cilia and flagella undergo maturation processes that include the addition of specific proteins and modifications that enhance their stability and function. 7. **Regulation**: The formation and maintenance of cilia and flagella are tightly regulated by various signaling pathways to ensure proper function in response to cellular needs and environmental cues. In summary, centrioles are indispensable for initiating the formation of cilia and flagella by serving as basal bodies that nucleate microtubules. Their precise duplication and positioning are critical steps in the assembly of these complex structures, which are vital for cellular motility and sensory functions across diverse eukaryotic organisms. Understanding the role of centrioles in cilia and flagella formation highlights their significance in maintaining cellular integrity and function.
Centrosome Organization
Centrosome organization is a critical aspect of cellular structure and function, particularly in relation to the roles of centrioles. The centrosome, often referred to as the microtubule-organizing center (MTOC), is a complex structure composed of two centrioles surrounded by a cloud of pericentriolar material (PCM). This organization is pivotal for several key cellular processes. At the heart of centrosome organization are the centrioles themselves, which are cylindrical structures made up of microtubules arranged in a specific pattern. These microtubules are essential for the formation of cilia, flagella, and the spindle fibers that separate chromosomes during cell division. The precise arrangement of centrioles within the centrosome ensures proper microtubule nucleation and stabilization, which in turn supports the integrity of the cytoskeleton. During interphase, the centrosome acts as a primary MTOC, orchestrating the organization of microtubules that provide structural support and facilitate intracellular transport. This function is crucial for maintaining cellular shape and enabling the movement of organelles and vesicles along microtubule tracks. In mitosis, the centrosome duplicates and separates to form two distinct poles of the mitotic spindle, ensuring accurate chromosome segregation. The PCM surrounding the centrioles contains proteins that regulate microtubule dynamics, such as γ-tubulin ring complexes (γ-TuRCs), which are essential for nucleating new microtubules. This intricate organization allows for rapid reorganization of the microtubule network in response to cellular needs, such as during cell migration or cytokinesis. Moreover, centrosome organization plays a significant role in ciliogenesis—the formation of cilia. In many cell types, one of the centrioles migrates to the cell surface and transforms into a basal body, which then templates the growth of a cilium. This process is vital for sensory perception and fluid movement in epithelial cells. Dysregulation of centrosome organization has been implicated in various diseases, including cancer and ciliopathies. For instance, aberrant centrosome duplication can lead to genomic instability, a hallmark of cancer cells. Conversely, defects in ciliogenesis due to centrosome dysfunction can result in developmental disorders such as polycystic kidney disease. In summary, the organization of the centrosome is a highly regulated and dynamic process that underpins several fundamental cellular activities. The precise arrangement of centrioles within the centrosome ensures proper microtubule organization, which is essential for maintaining cellular integrity, facilitating intracellular transport, and supporting accurate cell division. Understanding centrosome organization provides valuable insights into cellular biology and highlights the critical role that centrioles play in maintaining cellular health.
Cell Division and Cytokinesis
Cell division and cytokinesis are intricate processes that are crucial for the growth, repair, and reproduction of living organisms. At the heart of these processes are centrioles, which play a pivotal role in ensuring their successful execution. Cell division, specifically mitosis and meiosis, involves the replication of DNA followed by the precise distribution of genetic material into daughter cells. During this phase, centrioles duplicate to form a pair that migrates to opposite poles of the cell, where they organize the microtubules of the mitotic spindle. This spindle apparatus is essential for aligning chromosomes correctly and separating them into two sets, each destined for a new cell. Cytokinesis, the final stage of cell division, involves the physical separation of the cytoplasm to create two distinct daughter cells. In many eukaryotic cells, particularly in animal cells, cytokinesis occurs through a process known as cleavage. Here, the actin cytoskeleton contracts to form a cleavage furrow that gradually deepens until the cell splits into two. Centrioles contribute indirectly by helping to position the mitotic spindle correctly, ensuring that the cleavage furrow forms at the right location. In plant cells and some protists, cytokinesis occurs via a different mechanism involving the formation of a cell plate. This process begins with the assembly of a phragmoplast—a structure composed of microtubules and vesicles—at the center of the cell. The phragmoplast gradually expands outward until it reaches the cell wall, where it fuses to form a new cell wall separating the two daughter cells. While centrioles are not directly involved in this type of cytokinesis, their role in organizing the mitotic spindle ensures that chromosomes are properly aligned and separated before this stage begins. The precision and coordination required for cell division and cytokinesis underscore the importance of centrioles. These organelles not only facilitate accurate chromosome segregation but also contribute to maintaining cellular polarity and ensuring proper cytoplasmic division. Their dysfunction can lead to aberrant cell division patterns, which are often associated with developmental disorders or cancer. Thus, understanding the functions of centrioles provides valuable insights into both normal cellular processes and pathological conditions, highlighting their critical role in maintaining cellular integrity and function.
Role in Cellular Processes
In the intricate world of cellular processes, several key mechanisms work in harmony to ensure the proper functioning and survival of cells. At the heart of these processes are three critical components: mitotic spindle formation, microtubule organization, and cell signaling and regulation. Each of these elements plays a distinct yet interconnected role in maintaining cellular integrity. Mitotic spindle formation is essential for accurate chromosome segregation during cell division, ensuring genetic material is distributed evenly between daughter cells. Microtubule organization underpins the structural framework of cells, facilitating transport, division, and overall cellular architecture. Meanwhile, cell signaling and regulation govern the communication networks that dictate cellular behavior, influencing everything from growth and differentiation to apoptosis. Understanding these interrelated processes is crucial for grasping the complex dynamics of cellular life. By delving into these aspects, we can appreciate the precision and coordination required for cells to function optimally. Let us begin by examining the pivotal role of mitotic spindle formation in cellular division.
Mitotic Spindle Formation
Mitotic spindle formation is a critical process in cellular division, ensuring the precise segregation of chromosomes during mitosis. This intricate structure, composed of microtubules and associated proteins, emerges from the centrosome, a region of the cell that houses the centrioles. At the onset of mitosis, the centrosome duplicates and separates, with each centrosome migrating to opposite poles of the cell. This separation is crucial as it establishes the bipolar nature of the mitotic spindle, allowing for the balanced distribution of chromosomes. The formation of the mitotic spindle involves several key steps. Initially, microtubules nucleate from the centrosomes and extend towards the center of the cell. These microtubules can be categorized into three types: kinetochore microtubules, which attach to the kinetochores on sister chromatids; interpolar microtubules, which overlap at the spindle equator; and astral microtubules, which extend towards the cell cortex. The dynamic instability of microtubules allows them to rapidly assemble and disassemble, facilitating their search-and-capture mechanism to attach to kinetochores. The attachment of kinetochore microtubules to kinetochores is mediated by specific proteins that ensure proper chromosome alignment at the metaphase plate. This alignment is essential for accurate chromosome segregation; misalignment can lead to aneuploidy, a condition associated with various diseases and developmental disorders. The tension generated by the opposing forces of kinetochore microtubules and the cohesion between sister chromatids maintains this alignment until the onset of anaphase. During anaphase, the sister chromatids separate as the kinetochore microtubules shorten, pulling the chromosomes towards the poles. Simultaneously, interpolar microtubules slide past each other, contributing to spindle elongation and further separating the poles. This coordinated movement ensures that each daughter cell receives a complete and identical set of chromosomes. The regulation of mitotic spindle formation is tightly controlled by various checkpoints and signaling pathways. The spindle assembly checkpoint (SAC) monitors the attachment of kinetochores to microtubules and delays anaphase onset until all chromosomes are properly aligned. This checkpoint prevents premature separation of chromosomes, thereby maintaining genomic integrity. In summary, mitotic spindle formation is a highly regulated process that relies on the precise coordination of centrosome separation, microtubule dynamics, and kinetochore attachment. This complex structure plays a pivotal role in ensuring accurate chromosome segregation during mitosis, underscoring its critical importance in maintaining cellular and genomic integrity. The dysregulation of mitotic spindle components has been implicated in various diseases, including cancer, highlighting the significance of understanding these mechanisms in both normal cellular processes and pathological conditions.
Microtubule Organization
Microtubule organization is a critical aspect of cellular structure and function, particularly in relation to the role of centrioles. Centrioles, small cylindrical organelles found in the cytoplasm of eukaryotic cells, serve as the primary microtubule organizing centers (MTOCs). These structures are essential for the nucleation and stabilization of microtubules, which are dynamic filaments composed of tubulin proteins. The precise organization of microtubules by centrioles underpins various cellular processes, including cell division, intracellular transport, and the maintenance of cell shape. During cell division, centrioles play a pivotal role in organizing the mitotic spindle, a complex structure composed of microtubules that separates chromosomes into daughter cells. Each centriole duplicates to form a pair, which then migrates to opposite poles of the cell to anchor the spindle fibers. This precise alignment ensures accurate chromosome segregation, a process crucial for genetic integrity. Additionally, centrioles contribute to the formation of the centrosome, a region around the centrioles where microtubules nucleate and radiate outward, further stabilizing the spindle apparatus. Beyond cell division, microtubules organized by centrioles facilitate intracellular transport. Motor proteins such as kinesin and dynein move along microtubule tracks, transporting vesicles, organelles, and other cargo throughout the cell. This transport system is vital for maintaining cellular homeostasis by ensuring that essential molecules and organelles reach their correct destinations. For instance, in neurons, microtubules organized by centrioles at the cell body extend into axons and dendrites, enabling the transport of synaptic vesicles and other components necessary for neuronal function. The organization of microtubules also influences cell morphology and polarity. In epithelial cells, for example, the centrosome acts as a hub from which microtubules extend towards the cell periphery, helping to maintain the cell's shape and polarity. This structural framework supports the positioning of organelles and the establishment of cell junctions, which are critical for tissue integrity and function. Moreover, centriole-organized microtubules are involved in cilia formation and function. Cilia are microtubule-based structures that protrude from the cell surface and are essential for sensory perception and motility in certain cell types. The basal body, a modified centriole, anchors cilia at the cell surface and organizes the microtubule doublets that comprise the cilium's axoneme. This precise organization is necessary for ciliary movement and signaling, which are crucial in contexts such as respiratory epithelial function and embryonic development. In summary, the organization of microtubules by centrioles is fundamental to a wide range of cellular processes. From ensuring accurate chromosome segregation during cell division to facilitating intracellular transport and maintaining cell shape, the role of centrioles in microtubule organization underscores their importance in cellular biology. Understanding these mechanisms provides insights into how cells maintain their structural integrity and functional capabilities, highlighting the intricate interplay between centrioles, microtubules, and overall cellular health.
Cell Signaling and Regulation
Cell signaling and regulation are pivotal components in the intricate dance of cellular processes, ensuring that cells respond appropriately to their environment and maintain homeostasis. At the heart of this complex system lies a network of molecular pathways that facilitate communication between cells and their surroundings. This communication is mediated by signaling molecules, such as hormones, growth factors, and neurotransmitters, which bind to specific receptors on the cell surface or within the cell. Upon binding, these receptors trigger a cascade of downstream signaling events that involve various proteins, including kinases, phosphatases, and adapter proteins. These proteins interact in a highly regulated manner to transmit the signal through the cell, often culminating in changes to gene expression, protein activity, or cellular behavior. One of the key aspects of cell signaling is its ability to be finely tuned and regulated. This is achieved through feedback loops, where the output of a signaling pathway can modulate its own input, either by enhancing or inhibiting the signal. For instance, in the case of the mitogen-activated protein kinase (MAPK) pathway, which is crucial for cell proliferation and differentiation, feedback mechanisms ensure that the signal is not overly amplified or sustained, preventing uncontrolled cell growth. Additionally, cells employ mechanisms such as receptor desensitization and degradation to terminate signals once they have fulfilled their purpose, thereby maintaining cellular homeostasis. The role of cell signaling in cellular processes is multifaceted. It plays a critical role in cell growth and division by regulating the cell cycle. For example, growth factors like epidermal growth factor (EGF) bind to their receptors, triggering a signaling cascade that promotes cell proliferation. Similarly, signaling pathways are essential for cell survival and apoptosis (programmed cell death), with pathways like the PI3K/AKT pathway promoting survival by inhibiting pro-apoptotic proteins. Moreover, cell signaling is integral to cellular differentiation and development. During embryonic development, signaling pathways such as the Wnt/β-catenin pathway guide the formation of tissues and organs by specifying cell fate decisions. In adult tissues, these pathways continue to play roles in maintaining tissue homeostasis and regenerating damaged tissues. In the context of centrioles, which are microtubule-based structures involved in the formation of cilia, flagella, and the spindle poles during cell division, cell signaling pathways are crucial for their proper function. For instance, signaling through the Hedgehog pathway can influence ciliogenesis (the formation of cilia), while other pathways regulate centriole duplication and separation during the cell cycle. Dysregulation in these signaling pathways can lead to abnormalities in centriole function, contributing to diseases such as ciliopathies and cancer. In summary, cell signaling and regulation are fundamental to the dynamic and responsive nature of cellular processes. By orchestrating a complex interplay of molecular interactions, these systems ensure that cells adapt to their environment, grow, differentiate, and survive in a highly regulated manner. Understanding these mechanisms provides valuable insights into cellular biology and has significant implications for the diagnosis and treatment of various diseases.