What Is A Prodrug
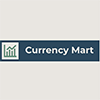
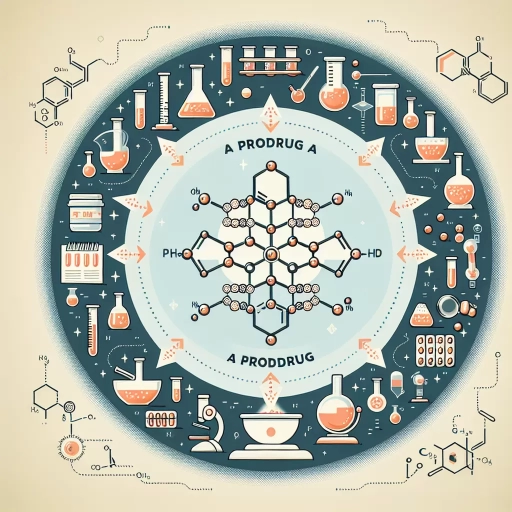
In the realm of pharmaceuticals, the concept of prodrugs has revolutionized the way we approach drug delivery and efficacy. A prodrug is a compound that, after administration, is metabolized into a pharmacologically active drug. This innovative approach addresses several critical issues in drug development, including poor bioavailability, toxicity, and targeted delivery. The advantages of prodrugs are multifaceted; they can enhance the therapeutic index of a drug by reducing side effects and improving patient compliance. Furthermore, prodrugs have diverse applications across various therapeutic areas, from cancer treatment to neurological disorders. To fully understand the significance of prodrugs, it is essential to delve into their definition and underlying concept. This article will explore the definition and concept of prodrugs, highlighting their advantages and benefits, as well as providing examples of their applications in modern medicine. By grasping these fundamental aspects, we can appreciate the transformative potential of prodrugs in advancing healthcare outcomes. Let us begin by examining the definition and concept of prodrugs, which form the foundation of their utility and impact.
Definition and Concept of Prodrugs
Prodrugs are a fascinating class of compounds that have revolutionized the field of pharmacology by enhancing the therapeutic efficacy and reducing the side effects of various drugs. At their core, prodrugs are inactive or less active derivatives of pharmacologically active drugs, designed to undergo metabolic conversion in the body to release the active drug. This concept is multifaceted and can be understood through several key aspects. First, the **Chemical Structure and Modification** of prodrugs play a crucial role in their design, as specific modifications are made to the chemical structure of the parent drug to improve its pharmacokinetic properties. Second, the **Pharmacological Activation Process** is essential, as it involves the biochemical transformations that convert the prodrug into its active form. Lastly, understanding the **Historical Development and Evolution** of prodrugs provides insight into how this concept has evolved over time, from its early beginnings to the sophisticated designs seen today. By delving into these areas, we can appreciate the complexity and innovation behind prodrug development. Let us begin by examining the **Chemical Structure and Modification** that underpin the creation of these therapeutic agents.
Chemical Structure and Modification
Chemical structure and modification are pivotal concepts in the development of prodrugs, which are inactive compounds that undergo metabolic conversion in the body to release the active drug. Understanding the intricacies of chemical structure is essential because it directly influences the pharmacokinetic and pharmacodynamic properties of a prodrug. The molecular architecture of a prodrug, including its functional groups, stereochemistry, and overall molecular weight, determines how it is absorbed, distributed, metabolized, and excreted (ADME) in the body. Modification of the chemical structure can significantly enhance these properties. For instance, adding hydrophilic groups can improve solubility and bioavailability, while incorporating lipophilic moieties can enhance membrane permeability. The strategic placement of these groups ensures that the prodrug remains inactive until it reaches its target site, where specific enzymes or conditions trigger its conversion into the active drug. This targeted approach minimizes side effects and maximizes therapeutic efficacy. One common modification involves the use of ester or amide linkages, which are susceptible to hydrolysis by enzymes such as esterases or amidases. These linkages can be designed to be stable enough to withstand premature activation during absorption but labile enough to be cleaved at the desired site of action. Another strategy involves the use of pro-moieties that are cleaved through oxidative or reductive processes, leveraging the body's natural metabolic pathways to activate the drug. The design of prodrugs also considers the balance between stability and reactivity. A prodrug must be stable enough to survive the initial stages of ADME but reactive enough to be converted into the active form at the appropriate time and location. Advanced computational models and in vitro studies are often employed to predict and optimize these properties, ensuring that the prodrug's chemical structure aligns with its intended pharmacological profile. Furthermore, understanding the stereochemistry of a prodrug is crucial because it can affect both its pharmacokinetics and pharmacodynamics. Enantiomers (non-superimposable mirror images) of a prodrug may exhibit different rates of metabolism or varying degrees of interaction with target enzymes, leading to differences in efficacy and safety. Therefore, stereochemically pure prodrugs are often preferred to avoid unpredictable outcomes. In summary, the chemical structure and modification of prodrugs are critical elements in their design and development. By carefully engineering the molecular structure, scientists can create prodrugs that are tailored for optimal delivery, activation, and therapeutic effect while minimizing adverse reactions. This precision in chemical design underscores the sophisticated approach required to transform an inactive compound into a potent therapeutic agent, highlighting the complexity and innovation inherent in prodrug development.
Pharmacological Activation Process
The pharmacological activation process is a critical component in the concept and definition of prodrugs, which are inactive compounds that undergo metabolic conversion in the body to release the active drug. This process involves several key steps that ensure the prodrug is transformed into its therapeutic form, thereby exerting its intended pharmacological effect. Initially, the prodrug is administered via a suitable route, such as oral, intravenous, or topical, depending on its formulation and intended use. Once inside the body, the prodrug is subject to various enzymatic and non-enzymatic transformations facilitated by metabolic pathways. These transformations can occur in various tissues, including the liver, where cytochrome P450 enzymes play a significant role, or in other organs and tissues where specific enzymes are present. The activation process typically involves hydrolysis, reduction, or oxidation reactions that cleave or modify the prodrug molecule to reveal the active drug. For instance, ester prodrugs are often hydrolyzed by esterases to release the active moiety, while amide prodrugs may undergo hydrolysis by amidases. The specificity and efficiency of these enzymatic reactions are crucial for ensuring that the prodrug is converted into its active form at the desired site and rate. This targeted activation helps in minimizing side effects and improving bioavailability, as the active drug is released only where it is needed. Moreover, the pharmacological activation process can be influenced by factors such as pH, temperature, and the presence of cofactors or inhibitors. For example, some prodrugs are designed to be activated in specific pH environments, such as the acidic conditions found in certain tumors or the alkaline environment of the small intestine. This pH-dependent activation allows for more precise targeting and reduces systemic exposure to the active drug, thereby enhancing therapeutic efficacy while reducing toxicity. In addition to enzymatic activation, some prodrugs undergo non-enzymatic transformations, such as chemical hydrolysis or photodegradation, which can be triggered by light or other environmental factors. These mechanisms further expand the versatility of prodrug design, enabling researchers to tailor the activation process to specific therapeutic needs. Overall, the pharmacological activation process is a sophisticated and highly regulated series of biochemical events that convert an inactive prodrug into its active therapeutic form. By understanding and manipulating these processes, scientists can develop prodrugs that offer improved pharmacokinetics, enhanced efficacy, and reduced side effects, ultimately leading to better patient outcomes. This intricate interplay between prodrug design, metabolic pathways, and enzymatic transformations underscores the complexity and potential of prodrug technology in modern pharmacotherapy.
Historical Development and Evolution
The historical development and evolution of prodrugs are deeply intertwined with advancements in pharmaceutical science and the quest for improved drug efficacy and safety. The concept of prodrugs, which involves designing inactive compounds that are metabolized in the body to release the active drug, has its roots in the early 20th century. However, it wasn't until the 1950s and 1960s that the term "prodrug" began to gain traction. During this period, researchers started exploring ways to enhance the bioavailability, solubility, and stability of drugs by modifying their chemical structures. One of the earliest and most influential examples of prodrug development is the synthesis of sulfasalazine, a compound used in the treatment of rheumatoid arthritis and ulcerative colitis. Discovered in the 1940s, sulfasalazine is a prodrug that is cleaved in the colon to release its active components: sulfapyridine and 5-aminosalicylic acid. This pioneering work laid the groundwork for further research into prodrug design. The 1970s and 1980s saw significant strides in prodrug technology, particularly with the introduction of ester and amide linkages. These linkages allowed for the creation of prodrugs that could be enzymatically cleaved in specific tissues or organs, thereby targeting the active drug to its site of action while minimizing systemic side effects. For instance, the development of valaciclovir, a prodrug of acyclovir, revolutionized the treatment of herpes simplex virus infections by improving oral bioavailability and reducing dosing frequency. In recent decades, advances in biotechnology and molecular biology have further propelled the evolution of prodrugs. The use of recombinant enzymes and gene therapy has enabled the design of more sophisticated prodrug systems. For example, the development of antibody-directed enzyme prodrug therapy (ADEPT) involves conjugating enzymes to antibodies that target specific cancer cells. Once at the tumor site, these enzymes activate prodrugs into their cytotoxic forms, significantly enhancing therapeutic efficacy while reducing harm to healthy tissues. Moreover, the integration of nanotechnology and targeted drug delivery systems has opened new avenues for prodrug development. Nanoparticles and liposomes can be engineered to encapsulate prodrugs, allowing for controlled release and enhanced targeting capabilities. This approach has shown promise in treating various diseases, including cancer, where the ability to deliver high concentrations of active drugs directly to tumor cells is crucial. In conclusion, the historical development and evolution of prodrugs reflect a continuous effort to improve drug therapy through innovative chemical modifications and advanced delivery systems. From early examples like sulfasalazine to modern approaches involving nanotechnology and gene therapy, the field of prodrug research has evolved significantly, offering potential solutions to long-standing challenges in drug delivery and efficacy. As pharmaceutical science continues to advance, it is likely that prodrugs will play an increasingly important role in the development of new therapeutic agents.
Advantages and Benefits of Prodrugs
Prodrugs, a class of compounds designed to be metabolized into their active form within the body, offer several significant advantages that enhance their therapeutic efficacy and patient experience. One of the primary benefits of prodrugs is their ability to improve bioavailability and solubility, which can be particularly crucial for drugs that are poorly absorbed or have limited solubility in their native form. Additionally, prodrugs can be engineered for targeted delivery, reducing side effects by ensuring that the active drug is released only at the desired site of action. This targeted approach not only minimizes adverse reactions but also increases the therapeutic index of the drug. Furthermore, prodrugs can improve patient compliance and efficacy by simplifying dosing regimens and enhancing the overall pharmacokinetic profile of the medication. By addressing these key areas, prodrugs provide a multifaceted solution to common challenges in drug development and administration. Let's delve deeper into how prodrugs enhance bioavailability and solubility, a critical aspect that sets them apart from traditional drugs.
Enhanced Bioavailability and Solubility
Enhanced bioavailability and solubility are pivotal advantages of prodrugs, significantly contributing to their therapeutic efficacy. Bioavailability refers to the fraction of a drug that reaches systemic circulation in its active form, while solubility determines how easily a drug can be absorbed by the body. Prodrugs are designed to overcome the limitations of their parent compounds by improving these critical pharmacokinetic properties. By modifying the chemical structure of a drug, prodrugs can enhance solubility, particularly for lipophilic (fat-soluble) drugs that struggle with aqueous solubility. This modification allows for better dissolution in biological fluids, facilitating more efficient absorption across biological membranes. For instance, prodrugs like valacyclovir (a prodrug of acyclovir) exhibit higher aqueous solubility compared to their active metabolites, leading to improved oral bioavailability and subsequently higher plasma concentrations of the active drug. This enhanced bioavailability translates into better therapeutic outcomes, as more of the active drug is available at the site of action. Additionally, improved solubility can reduce the need for large volumes of solvent or co-solvents, making the formulation process simpler and more patient-friendly. Furthermore, enhanced bioavailability can lead to reduced dosing frequency, which improves patient compliance and reduces the risk of adverse effects associated with peak drug concentrations. Overall, the strategic design of prodrugs to enhance bioavailability and solubility underscores their potential to revolutionize drug delivery by ensuring that therapeutic agents reach their targets more effectively, thereby optimizing treatment outcomes.
Targeted Delivery and Reduced Side Effects
**Targeted Delivery and Reduced Side Effects** One of the most significant advantages of prodrugs is their ability to achieve targeted delivery and minimize side effects, thereby enhancing the therapeutic efficacy of the active drug. Prodrugs are designed to be inactive or less active until they reach the specific site of action, where they are metabolized into their active form. This targeted approach allows for a more precise delivery of the therapeutic agent, reducing exposure to healthy tissues and organs. For instance, prodrugs can be engineered to be activated by enzymes that are overexpressed in cancer cells, ensuring that the active drug is released primarily at the tumor site. This not only increases the concentration of the drug at the target location but also diminishes its presence in non-target areas, leading to a substantial reduction in side effects. Moreover, prodrugs can be tailored to exploit specific physiological conditions or biochemical pathways present in diseased tissues. For example, certain prodrugs are activated by the acidic environment found in solid tumors or by the presence of specific enzymes that are elevated in diseased states. This targeted activation ensures that the active drug is released where it is needed most, while sparing normal tissues from unnecessary exposure. Additionally, prodrugs can be formulated to improve solubility, stability, and bioavailability, which further enhances their delivery profile and reduces systemic toxicity. The reduction in side effects is particularly beneficial for patients undergoing long-term treatment regimens. By minimizing the exposure of healthy tissues to the active drug, prodrugs can significantly lower the risk of adverse reactions such as nausea, fatigue, and organ damage. This improved safety profile not only enhances patient compliance but also allows for higher doses to be administered when necessary, potentially leading to better treatment outcomes. Furthermore, the targeted delivery mechanism of prodrugs can also reduce the development of drug resistance, as the active drug is delivered directly to the site of action without being metabolized prematurely. In summary, the ability of prodrugs to achieve targeted delivery and reduce side effects is a critical advantage that underscores their potential in modern pharmacotherapy. By leveraging specific biochemical and physiological characteristics of diseased tissues, prodrugs can enhance therapeutic efficacy while minimizing harm to healthy tissues, thereby improving patient outcomes and quality of life. This precision in drug delivery highlights the innovative potential of prodrugs in addressing some of the most challenging aspects of drug therapy and underscores their importance in the development of next-generation pharmaceuticals.
Improved Patient Compliance and Efficacy
Improved patient compliance and efficacy are pivotal advantages of prodrugs, significantly enhancing the therapeutic outcomes of various medications. Prodrugs, by design, are inactive compounds that undergo metabolic conversion in the body to release the active drug. This transformation can address several challenges associated with traditional drug formulations, thereby boosting patient adherence and treatment effectiveness. One of the primary benefits of prodrugs is their ability to improve bioavailability. Many drugs suffer from poor absorption or rapid metabolism, leading to reduced efficacy. Prodrugs can be engineered to overcome these barriers by enhancing solubility, stability, or permeability, ensuring that the active drug reaches its target site in optimal concentrations. For instance, valacyclovir, a prodrug of acyclovir, exhibits higher bioavailability compared to its parent compound, making it more effective in treating herpes infections. Another significant advantage is the reduction in side effects. Traditional drugs often cause adverse reactions due to their immediate release and high peak concentrations. Prodrugs, however, can be designed to release the active drug in a controlled manner, minimizing peak concentrations and thus reducing the risk of side effects. This is particularly beneficial for drugs with narrow therapeutic windows, such as certain chemotherapeutic agents. For example, the prodrug capecitabine converts to 5-fluorouracil (5-FU) preferentially in tumor tissues, reducing systemic toxicity and improving tolerability. Prodrugs also enhance patient compliance by simplifying dosing regimens. Drugs with short half-lives or those requiring frequent administration can be burdensome for patients, leading to non-adherence. Prodrugs can be formulated to provide sustained release of the active drug, reducing the frequency of dosing and making treatment more manageable. This is evident with drugs like oseltamivir phosphate, a prodrug of oseltamivir carboxylate, which is used to treat influenza and requires less frequent dosing compared to other antiviral medications. Furthermore, prodrugs can be tailored to target specific tissues or cells, improving therapeutic selectivity. This targeted delivery reduces off-target effects and enhances the therapeutic index of the drug. For example, the prodrug lisinopril is converted to its active form primarily in the liver and kidneys, where it exerts its antihypertensive effects without causing significant systemic side effects. In addition, prodrugs offer a versatile platform for drug development. They can be designed to overcome various pharmacokinetic and pharmacodynamic limitations of existing drugs, extending their therapeutic utility. This flexibility allows pharmaceutical companies to breathe new life into older drugs by reformulating them as prodrugs, thereby improving their safety and efficacy profiles. In conclusion, the use of prodrugs significantly enhances patient compliance and efficacy by improving bioavailability, reducing side effects, simplifying dosing regimens, targeting specific tissues, and offering a versatile drug development platform. These advantages make prodrugs an invaluable tool in modern pharmacotherapy, enabling better treatment outcomes and improved patient care.
Examples and Applications of Prodrugs
Prodrugs, compounds that undergo metabolic conversion to release the active drug, have revolutionized the field of pharmacology by enhancing the efficacy, safety, and patient compliance of various therapeutic agents. This article delves into the multifaceted applications and examples of prodrugs, highlighting their clinical use in diverse therapeutic areas, showcasing case studies of successful prodrugs, and exploring future directions and research opportunities. By examining the clinical use of prodrugs across different therapeutic areas, we can understand how these compounds are tailored to address specific medical needs, such as improving bioavailability, reducing side effects, and targeting specific disease mechanisms. Case studies of successful prodrugs will illustrate the practical benefits and outcomes of these strategies, providing real-world examples that underscore their potential. Finally, looking ahead to future directions and research opportunities will reveal the exciting possibilities for further innovation in prodrug design and development. This comprehensive approach will provide a thorough understanding of the current state and future prospects of prodrugs, beginning with their clinical use in various therapeutic areas.
Clinical Use in Various Therapeutic Areas
Prodrugs have revolutionized the field of pharmacotherapy by enhancing the efficacy, safety, and patient compliance of various therapeutic agents. Their clinical use spans across multiple therapeutic areas, each leveraging the unique advantages of prodrug technology to overcome traditional drug limitations. In oncology, prodrugs like capecitabine and irinotecan are designed to be activated specifically within tumor cells, reducing systemic toxicity and improving therapeutic outcomes. For instance, capecitabine is converted into 5-fluorouracil (5-FU) by thymidine phosphorylase, an enzyme overexpressed in many tumors, thereby targeting cancer cells more selectively. In the realm of infectious diseases, prodrugs such as valacyclovir and valganciclovir offer enhanced bioavailability and reduced dosing frequency compared to their active metabolites acyclovir and ganciclovir. This not only improves patient adherence but also maintains therapeutic levels over longer periods, crucial for managing chronic infections like herpes simplex virus and cytomegalovirus. Similarly, in cardiovascular medicine, prodrugs like lisinopril and enalapril are converted into their active forms lisinoprilat and enalaprilat, respectively, which are potent angiotensin-converting enzyme (ACE) inhibitors used to treat hypertension and heart failure. Neurological disorders also benefit from prodrug technology. For example, levodopa, a precursor to dopamine, is often administered as a prodrug in combination with carbidopa to manage Parkinson's disease. Carbidopa inhibits the peripheral conversion of levodopa to dopamine, allowing more levodopa to cross the blood-brain barrier where it can exert its therapeutic effect. Additionally, in the treatment of epilepsy, prodrugs like fosphenytoin offer rapid conversion to phenytoin with fewer side effects compared to traditional intravenous phenytoin administration. In gastrointestinal diseases, prodrugs like balsalazide and olsalazine are designed to remain inactive until they reach the colon where they are cleaved by bacterial azoreductases into their active forms, aminosalicylates. This targeted delivery minimizes systemic absorption and reduces side effects while effectively treating conditions such as ulcerative colitis. Furthermore, in ophthalmology, prodrugs like latanoprost are used to treat glaucoma by being converted into their active prostaglandin analogs within the eye, thereby reducing intraocular pressure with minimal systemic exposure. The versatility of prodrugs extends into dermatology as well, where they are used to enhance topical delivery. For instance, adapalene is a retinoid prodrug that is converted into its active form upon application to the skin, reducing irritation while maintaining efficacy in treating acne and other skin disorders. Overall, the strategic use of prodrugs across various therapeutic areas has significantly improved drug therapy by enhancing bioavailability, reducing toxicity, and improving patient outcomes. This innovative approach continues to expand the therapeutic potential of existing drugs and opens new avenues for drug development in diverse clinical settings.
Case Studies of Successful Prodrugs
Case studies of successful prodrugs highlight the strategic design and clinical efficacy of these compounds, transforming pharmacologically active but poorly bioavailable molecules into therapeutically effective drugs. One exemplary case is that of oseltamivir (Tamiflu), a prodrug of the antiviral agent oseltamivir carboxylate. Oseltamivir itself is poorly absorbed, but upon oral administration, it is efficiently converted by carboxylesterases in the liver to its active metabolite, which then inhibits the influenza virus neuraminidase enzyme. This prodrug strategy significantly enhances bioavailability and patient compliance, making oseltamivir a cornerstone in the treatment of influenza A and B. Another notable example is valacyclovir (Valtrex), a prodrug of acyclovir. Acyclovir, an antiviral drug used to treat herpes simplex virus infections, has limited oral bioavailability. Valacyclovir, however, is rapidly converted to acyclovir by the enzyme valacyclovir hydrolase in the liver, resulting in higher plasma concentrations of acyclovir compared to direct administration of acyclovir. This improved bioavailability translates into enhanced therapeutic efficacy and reduced dosing frequency, making valacyclovir a preferred treatment option for herpes infections. The prodrug approach has also been successfully applied in oncology with drugs like capecitabine (Xeloda). Capecitabine is a prodrug of 5-fluorouracil (5-FU), a chemotherapeutic agent used to treat various cancers. Upon ingestion, capecitabine undergoes a series of enzymatic conversions, ultimately yielding 5-FU preferentially in tumor tissues due to the higher expression of thymidine phosphorylase in these cells. This targeted delivery minimizes systemic side effects associated with 5-FU while maintaining its antitumor activity, thereby improving the therapeutic index. Furthermore, the prodrug strategy has been instrumental in overcoming solubility issues, as seen with the development of fosamprenavir (Lexiva). Fosamprenavir is a prodrug of amprenavir, an HIV protease inhibitor with poor aqueous solubility. The phosphate ester modification in fosamprenavir enhances its solubility, facilitating easier formulation and administration. Once ingested, fosamprenavir is hydrolyzed to amprenavir, which then inhibits HIV protease effectively. This design not only improves the pharmacokinetic profile but also enhances patient adherence due to reduced pill burden. These case studies underscore the versatility and clinical utility of prodrugs in addressing various pharmacokinetic and pharmacodynamic challenges. By leveraging metabolic pathways to convert inactive or poorly bioavailable compounds into their active forms, prodrugs can significantly enhance drug efficacy, reduce side effects, and improve patient outcomes. As such, the prodrug approach remains a valuable tool in drug development, offering innovative solutions to complex therapeutic challenges.
Future Directions and Research Opportunities
As we continue to explore the vast potential of prodrugs, several future directions and research opportunities emerge that promise to revolutionize the field of pharmacology. One of the most promising areas is the development of targeted prodrugs, which are designed to activate only in specific tissues or cells, thereby minimizing side effects and enhancing therapeutic efficacy. This could be achieved through the use of stimuli-responsive prodrugs that react to environmental cues such as pH, temperature, or the presence of specific enzymes, allowing for precise drug delivery and activation. Another significant direction is the integration of prodrug technology with nanotechnology and gene therapy. By encapsulating prodrugs within nanoparticles or liposomes, researchers can improve drug stability, enhance bioavailability, and facilitate targeted delivery to diseased sites. Additionally, combining prodrugs with gene editing tools like CRISPR/Cas9 could enable the creation of personalized therapies where the prodrug is activated by a genetically engineered enzyme, offering a new frontier in precision medicine. The application of artificial intelligence (AI) and machine learning (ML) in prodrug design is also an exciting area of research. AI algorithms can predict the metabolic pathways of prodrugs, identify potential activation sites, and optimize the chemical structure for better pharmacokinetics and pharmacodynamics. This computational approach can significantly accelerate the drug discovery process and reduce the need for extensive experimental screening. Furthermore, there is a growing interest in developing prodrugs for neglected diseases and rare conditions where traditional drug development may not be economically viable. By leveraging prodrug technology, researchers can create cost-effective treatments that are tailored to the specific needs of these patient populations, thereby addressing significant unmet medical needs. In addition to these technological advancements, there is a critical need for more comprehensive studies on the long-term safety and efficacy of prodrugs. Longitudinal clinical trials and post-marketing surveillance will be essential in understanding the full spectrum of benefits and risks associated with prodrug therapy. This data will not only inform clinical practice but also guide future research directions. Lastly, interdisciplinary collaboration between chemists, pharmacologists, clinicians, and engineers will be crucial in driving innovation in prodrug research. By pooling their expertise, researchers can overcome the complex challenges associated with prodrug development and translation into clinical practice. This collaborative approach will ensure that the full potential of prodrugs is realized, leading to improved patient outcomes and advancements in healthcare. In summary, the future of prodrug research is rich with opportunities for innovation and discovery. From targeted delivery systems to AI-driven design, and from addressing neglected diseases to ensuring long-term safety, the field is poised for significant advancements that will transform the way we approach drug therapy. As we continue to explore these avenues, we are likely to see a new generation of prodrugs that are more effective, safer, and more personalized than ever before.