What Is Power Engineering
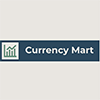
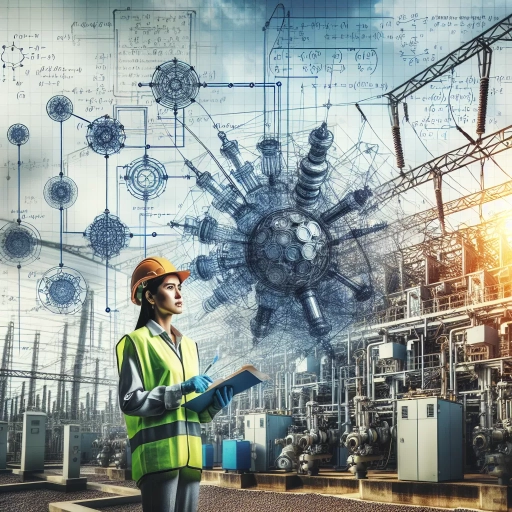
Power engineering is a dynamic and critical field that underpins modern society by ensuring the reliable and efficient generation, transmission, and distribution of electrical power. This discipline is not only essential for powering homes and industries but also drives technological advancements and economic growth. In this article, we will delve into the multifaceted world of power engineering, starting with its definition and scope. We will explore how power engineering encompasses a broad range of activities from power generation to distribution, highlighting its importance in everyday life. Next, we will examine the core principles and technologies that form the backbone of power engineering, including electrical circuits, power systems analysis, and control systems. Finally, we will discuss modern trends and future directions in power engineering, such as renewable energy integration, smart grids, and energy storage solutions. By understanding these aspects, we can appreciate the complexity and innovation within this field. Let us begin by defining and understanding the scope of power engineering.
Definition and Scope of Power Engineering
Power engineering, a field that encompasses the generation, transmission, and distribution of electrical power, is a cornerstone of modern society. This high-quality, informative, and engaging article delves into the definition and scope of power engineering, providing a comprehensive overview of its historical development, key disciplines, and global impact. Historically, power engineering has evolved significantly from its early beginnings with the invention of the first electrical generators to the sophisticated systems we see today. The article explores this historical development in detail, highlighting key milestones and innovations that have shaped the field. Additionally, it breaks down the key disciplines within power engineering, such as electrical machines, power electronics, and control systems, which are crucial for understanding the intricacies of power generation and distribution. Finally, it examines the global impact and applications of power engineering, illustrating how this field affects economies, environments, and daily life worldwide. By understanding these aspects, readers gain a deeper appreciation for the complexity and importance of power engineering. Transitioning seamlessly into the historical development of power engineering, we begin our journey through time to see how this vital field has progressed over the years.
Historical Development of Power Engineering
The historical development of power engineering is a rich and transformative journey that spans centuries, marked by significant technological advancements and innovative breakthroughs. The earliest forms of power engineering date back to ancient civilizations, where simple machines like the waterwheel and windmill were used to harness natural energy sources for tasks such as grinding grain and pumping water. These primitive technologies laid the groundwork for more sophisticated systems. In the Industrial Revolution, power engineering underwent a profound transformation with the invention of the steam engine by James Watt in the late 18th century. This innovation revolutionized industry by providing a reliable and consistent source of mechanical power, enabling mass production and industrial growth. The subsequent development of electrical power systems in the 19th century, spearheaded by pioneers like Michael Faraday, James Clerk Maxwell, and Thomas Edison, introduced the concept of electrical power generation, transmission, and distribution. Edison's direct current (DC) system and Nikola Tesla's alternating current (AC) system set the stage for modern electrical grids. The early 20th century saw the rise of hydroelectric power plants, which harnessed the energy of rivers to generate electricity on a large scale. This period also witnessed significant advancements in thermal power plants, including coal-fired and gas-fired units, which became central to meeting increasing global energy demands. The mid-20th century introduced nuclear power as a new source of electricity generation, offering a cleaner alternative to fossil fuels but also presenting unique challenges related to safety and waste management. In recent decades, power engineering has continued to evolve with a focus on sustainability and efficiency. The development of renewable energy technologies such as solar photovoltaic systems, wind turbines, and geothermal power plants has become increasingly important as the world seeks to mitigate climate change. Advances in smart grid technologies and energy storage solutions like batteries have improved the reliability and flexibility of power systems. Additionally, advancements in materials science have led to more efficient power generation and transmission equipment. Today, power engineering is at the forefront of addressing global energy challenges through innovative solutions such as grid-scale energy storage, advanced nuclear reactors, and enhanced grid resilience. The integration of artificial intelligence and IoT technologies into power systems is further optimizing energy distribution and consumption patterns. As the world transitions towards cleaner energy sources and more efficient use of resources, the historical development of power engineering serves as a testament to human ingenuity and the relentless pursuit of technological progress. This evolution underscores the critical role that power engineering plays in shaping our modern world and ensuring a sustainable future for generations to come.
Key Disciplines Within Power Engineering
Power engineering encompasses a diverse array of disciplines that collectively ensure the efficient generation, transmission, distribution, and utilization of electrical power. At its core, **Electrical Power Generation** involves the conversion of various forms of energy (such as thermal, nuclear, hydro, wind, and solar) into electrical energy. This discipline focuses on the design, operation, and maintenance of power plants to optimize energy output while minimizing environmental impact. **Power Transmission** is another critical discipline, dealing with the high-voltage transmission lines and substations that transport electrical energy from power plants to load centers. Engineers in this field must ensure reliable and efficient transmission over long distances, managing issues like voltage regulation, power flow control, and grid stability. **Power Distribution** follows transmission, focusing on the delivery of electricity from substations to end-users through a network of medium and low-voltage lines. This involves designing distribution systems that are resilient to faults, efficient in energy delivery, and adaptable to changing load demands. **Power Systems Engineering** integrates these disciplines by analyzing the overall performance of the power grid. It involves studying power flow, fault analysis, stability assessment, and control systems to ensure the reliability and security of the electrical grid. This includes advanced technologies such as smart grids and grid modernization initiatives. **Electrical Machines and Drives** is a fundamental discipline that deals with the design, development, and application of electrical machines like generators, motors, and transformers. These machines are crucial for converting electrical energy into mechanical energy or vice versa, and their efficiency directly impacts the overall performance of power systems. **Control Systems** play a vital role in power engineering by enabling real-time monitoring and control of power generation, transmission, and distribution. Advanced control systems utilize technologies such as SCADA (Supervisory Control and Data Acquisition) and IoT (Internet of Things) to enhance grid reliability and efficiency. **Energy Storage** has become increasingly important as renewable energy sources gain prominence. This discipline involves developing technologies like batteries, supercapacitors, and other storage solutions to stabilize the grid by balancing supply and demand fluctuations. **High Voltage Engineering** deals with the design and operation of high-voltage equipment such as transmission lines, substations, and insulators. It requires a deep understanding of electrical insulation, corona effects, and electromagnetic interference to ensure safe and reliable operation at high voltages. **Electromagnetic Compatibility (EMC)** ensures that electrical devices and systems operate without interfering with each other or the environment. In power engineering, EMC is crucial for preventing electromagnetic interference that could disrupt power system operations. Each of these disciplines within power engineering is interconnected and essential for maintaining a robust, efficient, and sustainable electrical power infrastructure. Together, they form the backbone of modern society's energy needs, driving innovation and ensuring reliable access to electricity.
Global Impact and Applications
Power engineering, a discipline that encompasses the generation, transmission, distribution, and utilization of electrical power, has a profound global impact and diverse applications. Globally, power engineering plays a crucial role in economic development by providing the energy infrastructure necessary for industrialization and modernization. In developing countries, access to reliable electricity can significantly improve living standards by enabling the use of essential appliances, lighting homes, and powering healthcare facilities. Conversely, in developed nations, advanced power engineering technologies such as smart grids and renewable energy systems help mitigate climate change by reducing carbon emissions and promoting sustainable energy practices. The applications of power engineering are vast and multifaceted. In the industrial sector, power engineers design and maintain the electrical systems that drive manufacturing processes, ensuring efficiency and reliability. In transportation, power engineering is pivotal in the development of electric vehicles and high-speed rail systems, which are transforming the way people travel. Additionally, power engineers are at the forefront of integrating renewable energy sources like solar and wind into the grid, enhancing energy security and reducing dependence on fossil fuels. In the residential sector, smart home technologies powered by advanced power engineering allow for real-time energy monitoring and optimization, leading to significant energy savings. Furthermore, power engineering supports critical infrastructure such as hospitals, data centers, and emergency services by ensuring uninterrupted power supply through backup systems and grid resilience measures. From a technological standpoint, advancements in power electronics and control systems have enabled more efficient power conversion and transmission. This has led to the widespread adoption of technologies like high-voltage direct current (HVDC) transmission lines, which can transport electricity over long distances with minimal loss. The integration of IoT (Internet of Things) devices into power systems also enhances grid management by providing real-time data for predictive maintenance and fault detection. In summary, the global impact of power engineering is substantial, driving economic growth, improving quality of life, and supporting sustainable development. Its applications span across various sectors, from industry and transportation to residential and critical infrastructure, making it an indispensable field in modern society. As technology continues to evolve, the role of power engineering will only become more critical in shaping a sustainable and energy-efficient future.
Core Principles and Technologies in Power Engineering
Power engineering is a multifaceted field that relies on several core principles and technologies to ensure the efficient and reliable supply of electrical energy. At its foundation, power engineering encompasses three critical components: Electrical Power Generation Systems, Transmission and Distribution Networks, and Power System Control and Automation. Electrical Power Generation Systems are the starting point, where various methods such as thermal, nuclear, and renewable energy sources convert raw energy into electrical power. This generated power then needs to be transmitted over long distances through Transmission and Distribution Networks, which involve complex infrastructure and technologies to minimize energy loss and ensure safe delivery. Finally, Power System Control and Automation play a crucial role in managing the entire system, optimizing performance, and maintaining stability. Understanding these interconnected elements is essential for advancing power engineering and meeting the increasing global demand for electricity. By delving into each of these areas, we can appreciate the intricate mechanisms that underpin our modern energy landscape, beginning with the fundamental processes of Electrical Power Generation Systems.
Electrical Power Generation Systems
Electrical power generation systems are the backbone of modern society, providing the energy necessary to power homes, industries, and infrastructure. At the core of these systems are several key technologies and principles that ensure efficient and reliable electricity production. The primary methods of electrical power generation include thermal, hydroelectric, nuclear, wind, solar, and geothermal power. Thermal power plants, which account for a significant portion of global electricity production, operate by burning fossil fuels such as coal, natural gas, or oil to produce steam that drives turbines connected to generators. Hydroelectric power plants harness the kinetic energy of water flowing through dams to drive turbines, offering a renewable and clean source of energy. Nuclear power plants generate electricity through nuclear fission reactions that produce steam to drive turbines. Renewable energy sources like wind and solar power are increasingly important, with wind turbines converting wind kinetic energy into electrical energy and solar panels converting sunlight into electricity through photovoltaic cells. Geothermal power plants utilize heat from the Earth's interior to produce steam for turbines. The core principles underlying these systems include the conversion of mechanical energy into electrical energy via electromagnetic induction in generators. This process is governed by Faraday's law of electromagnetic induction, which states that an electric current is generated in a conductor by changing the magnetic field around it. Power engineering also involves the management of transmission and distribution networks to ensure that generated power is delivered efficiently to consumers. This includes the use of high-voltage transmission lines, substations for voltage transformation, and distribution lines that bring electricity directly to homes and businesses. Advanced technologies such as smart grids and grid management systems enhance the reliability and efficiency of these networks by integrating real-time data and automation. In addition to these technical aspects, power engineering must also consider environmental impact, safety standards, and economic viability. For instance, the shift towards renewable energy sources is driven by the need to reduce greenhouse gas emissions and mitigate climate change. Safety protocols are stringent to prevent accidents and ensure continuous operation without disruptions. Economic considerations involve balancing the cost of generating electricity with consumer affordability and market demand. Overall, electrical power generation systems are complex networks that rely on a combination of traditional and emerging technologies to meet the world's growing energy demands. Understanding the core principles and technologies in power engineering is crucial for developing sustainable, efficient, and reliable energy solutions for the future.
Transmission and Distribution Networks
Transmission and distribution networks are the backbone of modern power engineering, ensuring the reliable and efficient delivery of electricity from power plants to end-users. These networks operate under core principles that prioritize safety, efficiency, and reliability. **Transmission Networks** involve high-voltage lines (typically above 230 kV) that transport electricity over long distances with minimal energy loss. Key technologies include high-voltage transmission lines, substations, and transmission towers. These networks utilize advanced materials like high-temperature superconductors and smart grid technologies to enhance efficiency and reduce losses. **Distribution Networks**, on the other hand, are designed to deliver electricity at lower voltages (typically below 35 kV) to consumers through a complex web of power lines, substations, and distribution transformers. Here, technologies such as smart meters, grid automation systems, and renewable energy integration play crucial roles in optimizing energy distribution. The core principles guiding these networks include **reliability**, ensuring continuous power supply; **efficiency**, minimizing energy losses during transmission and distribution; **safety**, adhering to stringent safety standards to protect both the public and utility workers; and **sustainability**, integrating renewable energy sources to reduce environmental impact. Advanced technologies like **SCADA (Supervisory Control and Data Acquisition) systems** and **IoT (Internet of Things) devices** enable real-time monitoring and control, enhancing network performance. Additionally, **grid resilience** is a critical aspect, with utilities employing strategies such as microgrids and energy storage solutions to mitigate the impact of outages and natural disasters. Innovations in power engineering have significantly improved the functionality of transmission and distribution networks. For instance, **high-voltage direct current (HVDC) transmission** allows for more efficient long-distance transmission with lower losses compared to traditional alternating current (AC) systems. Furthermore, **smart grid technologies** enable dynamic pricing, demand response management, and predictive maintenance, contributing to a more efficient and responsive grid. The integration of **renewable energy sources** like solar and wind power into these networks is also becoming increasingly important, necessitating advanced grid management systems to handle variable power outputs. Overall, the design and operation of transmission and distribution networks are grounded in robust engineering principles and cutting-edge technologies. These networks are essential for maintaining the reliability and efficiency of the power supply chain, ensuring that electricity reaches consumers safely and efficiently. As power engineering continues to evolve with new technologies and challenges, the importance of well-designed transmission and distribution networks will only grow.
Power System Control and Automation
Power System Control and Automation is a critical component of modern power engineering, ensuring the reliable, efficient, and safe operation of electrical power systems. At its core, this discipline involves the use of advanced technologies to monitor, control, and optimize power generation, transmission, and distribution. The core principles include real-time monitoring through Supervisory Control and Data Acquisition (SCADA) systems, which gather data from various points in the power grid to provide a comprehensive view of system performance. This data is then analyzed using advanced algorithms and artificial intelligence to predict potential issues and optimize energy flow. Automation technologies such as Programmable Logic Controllers (PLCs) and Intelligent Electronic Devices (IEDs) play a pivotal role in executing control commands rapidly and accurately. These devices can automatically adjust parameters like voltage levels, frequency, and power flow to maintain stability and efficiency within the grid. Additionally, the integration of smart grid technologies enables two-way communication between utilities and consumers, allowing for dynamic load management and demand response strategies that can mitigate peak demand periods. Advanced control techniques such as Model Predictive Control (MPC) and adaptive control are also employed to handle the complexities of modern power systems, which include variable renewable energy sources like solar and wind. These techniques ensure that the grid can adapt to changing conditions in real-time, maintaining stability even under fluctuating power inputs. Furthermore, cybersecurity is a growing concern in power system control and automation due to the increasing reliance on digital technologies. Implementing robust security measures such as encryption, firewalls, and intrusion detection systems is essential to protect against potential cyber threats that could compromise the integrity of the power grid. In summary, Power System Control and Automation leverages cutting-edge technologies to enhance the reliability, efficiency, and security of electrical power systems. By integrating real-time monitoring, advanced automation, smart grid technologies, and robust cybersecurity measures, power engineers can ensure that modern power grids operate optimally, meeting the evolving demands of a dynamic energy landscape. This field is continuously evolving with new innovations and advancements in technology, making it a vital area of focus within the broader discipline of power engineering.
Modern Trends and Future Directions in Power Engineering
The landscape of power engineering is undergoing a significant transformation, driven by modern trends that are shaping the future of energy production, distribution, and consumption. At the forefront of these changes are three key areas: Renewable Energy Integration and Sustainability, Smart Grid Technologies and IoT Applications, and Energy Storage Solutions and Grid Resilience. As the world shifts towards cleaner and more sustainable energy sources, the integration of renewable energy into existing grids becomes paramount. This transition is crucial for reducing carbon footprints and ensuring long-term sustainability. Additionally, the adoption of smart grid technologies and IoT applications is revolutionizing how energy is managed, making it more efficient and responsive to real-time demands. Meanwhile, advancements in energy storage solutions are enhancing grid resilience by providing backup power during outages and stabilizing the grid during peak usage periods. These interconnected trends are not only transforming the power engineering sector but also paving the way for a more sustainable and reliable energy future. As we delve deeper into these innovations, it becomes clear that renewable energy integration and sustainability are foundational to this new era in power engineering.
Renewable Energy Integration and Sustainability
Renewable energy integration is a pivotal aspect of modern power engineering, driving the transition towards sustainability and mitigating climate change. As the world shifts away from fossil fuels, the integration of renewable sources such as solar, wind, hydro, and geothermal energy into the grid has become increasingly crucial. This integration involves advanced technologies and strategies to ensure a stable and efficient energy supply. Smart grids, equipped with IoT sensors and AI algorithms, play a key role in managing the variability of renewable energy sources by predicting output and optimizing distribution. Energy storage solutions, including batteries and other innovative technologies, help stabilize the grid by storing excess energy generated during peak production periods for use during demand spikes or when renewable sources are less active. Sustainability is at the heart of renewable energy integration. By leveraging renewable resources, power engineering can significantly reduce greenhouse gas emissions and other environmental impacts associated with traditional energy production. Policy frameworks and incentives are being implemented globally to encourage the adoption of renewable energy technologies. For instance, feed-in tariffs and tax credits have been instrumental in driving investment in solar and wind projects. Additionally, advancements in technology have made renewable energy more cost-competitive with fossil fuels, further accelerating its adoption. Future directions in power engineering will likely involve even greater emphasis on decentralized energy systems, where local communities generate their own power through microgrids. This approach not only enhances energy security but also promotes community engagement and economic development. Electric vehicles are also set to play a significant role, with vehicle-to-grid (V2G) technology allowing cars to act as energy storage units that can supply power back to the grid when needed. In conclusion, the integration of renewable energy into power systems is a cornerstone of modern power engineering, underpinning efforts towards sustainability and reducing our reliance on non-renewable resources. As technology continues to evolve and policy support strengthens, the future of power engineering looks increasingly green and resilient.
Smart Grid Technologies and IoT Applications
Smart Grid Technologies and IoT Applications are revolutionizing the field of power engineering, transforming traditional power systems into intelligent, adaptive, and efficient networks. At the heart of this transformation is the integration of advanced technologies such as smart meters, grid management systems, and IoT devices. Smart meters enable real-time monitoring and control of energy consumption, allowing utilities to manage demand more effectively and consumers to make informed decisions about their energy usage. Grid management systems leverage data analytics and machine learning to optimize energy distribution, predict potential outages, and ensure grid stability. IoT applications further enhance these capabilities by connecting a vast array of devices—from smart home appliances to industrial sensors—creating a comprehensive network that can respond dynamically to changing conditions. This interconnectedness facilitates the integration of renewable energy sources, such as solar and wind power, into the grid more seamlessly, promoting sustainability and reducing reliance on fossil fuels. Additionally, IoT-enabled devices can detect anomalies and perform predictive maintenance, reducing downtime and improving overall grid reliability. As these technologies continue to evolve, they will drive future directions in power engineering towards greater automation, resilience, and customer-centric service models, ultimately shaping a more sustainable and efficient energy future.
Energy Storage Solutions and Grid Resilience
Energy storage solutions are pivotal in enhancing grid resilience, a critical aspect of modern power engineering. As the world transitions towards a more sustainable and renewable energy mix, the need for reliable and efficient energy storage technologies becomes increasingly important. Battery energy storage systems (BESS), such as lithium-ion batteries, lead-acid batteries, and flow batteries, are among the most prevalent solutions. These systems help stabilize the grid by absorbing excess energy during periods of high production and releasing it during demand peaks or when renewable sources are intermittent. For instance, pumped hydro storage (PHS) and compressed air energy storage (CAES) offer large-scale storage capabilities that can support grid stability over extended periods. Advanced technologies like solid-state batteries and sodium-ion batteries are emerging as promising alternatives with enhanced safety, efficiency, and cost-effectiveness. Additionally, innovative solutions such as hydrogen storage and thermal energy storage are being explored to diversify the energy storage landscape. The integration of these technologies with smart grid systems enables real-time monitoring and optimization of energy distribution, thereby improving grid resilience. The deployment of energy storage solutions also facilitates the integration of distributed energy resources (DERs) such as solar and wind power into the grid. By mitigating the variability associated with these renewable sources, energy storage ensures a more stable and reliable power supply. Furthermore, energy storage can provide ancillary services like frequency regulation, voltage support, and backup power during outages, thereby enhancing overall grid resilience. In the future, advancements in materials science and battery chemistry are expected to drive down costs and increase the efficiency of energy storage systems. The integration of artificial intelligence (AI) and machine learning (ML) will further optimize energy storage operations by predicting energy demand patterns and optimizing charging/discharging cycles. As power engineering continues to evolve, the role of energy storage in ensuring grid resilience will become even more central, enabling a more sustainable, efficient, and reliable energy ecosystem.