What Kind Of Star Is The Sun
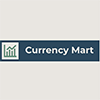
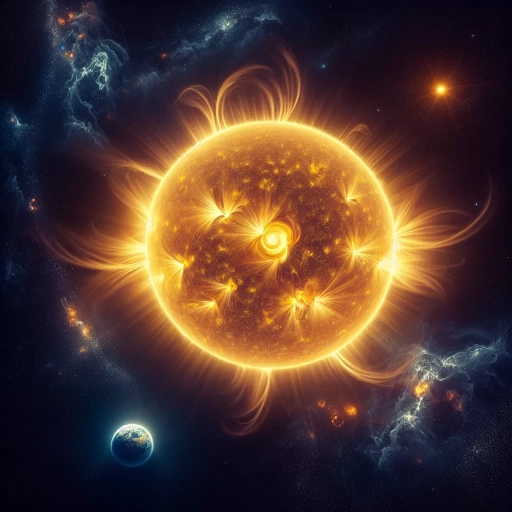
The Sun, the centerpiece of our solar system, is often taken for granted despite its critical role in sustaining life on Earth. However, understanding the Sun's nature and characteristics is essential for appreciating its significance. This article delves into the intricacies of what kind of star the Sun is, exploring three key aspects: its classification, physical and chemical properties, and how it compares to other stars. By examining the Sun's classification, we will uncover its place within the broader spectrum of stellar types, shedding light on why it is categorized as a G-type main-sequence star. We will also delve into its physical and chemical properties, including its size, temperature, and composition, which are crucial for understanding its life cycle and energy output. Finally, comparing the Sun to other stars will provide a broader context, highlighting both its uniqueness and commonalities with other celestial bodies. To begin this journey, let us first understand the Sun's classification, which sets the foundation for a deeper exploration of our star.
Understanding the Sun's Classification
The Sun, our celestial neighbor and the cornerstone of our solar system, is often taken for granted despite its profound impact on life and the universe. To truly appreciate the Sun's significance, it is essential to understand its classification within the broader context of stellar types. This involves delving into the various categories of stars, each with its unique characteristics and evolutionary stages. Specifically, we will explore the different stellar types and categories that help us categorize stars based on their properties. We will also examine the characteristics of main sequence stars, a group to which the Sun belongs, highlighting their defining features such as mass, luminosity, and surface temperature. Finally, we will place the Sun within the Hertzsprung-Russell Diagram, a fundamental tool in astrophysics that maps stars according to their luminosity and temperature. By understanding these concepts, we can gain a deeper insight into the Sun's classification and its role in the vast expanse of the cosmos. This article aims to provide a comprehensive overview of these key aspects, ultimately leading to a clearer understanding of the Sun's classification.
1. Stellar Types and Categories
Understanding the Sun's classification begins with an exploration of stellar types and categories, which are fundamental in astronomy for categorizing stars based on their physical properties. Stars are primarily classified using the Harvard Spectral Classification Scheme, which divides them into seven main categories: O, B, A, F, G, K, and M. These categories are determined by the star's surface temperature, with O being the hottest and M being the coolest. **O-type stars** are the most massive and luminous, with surface temperatures exceeding 30,000 Kelvin. These blue giants burn through their hydrogen fuel rapidly, leading to short lifetimes. **B-type stars** are also hot and luminous but less so than O-type stars, with surface temperatures ranging from 10,000 to 30,000 Kelvin. **A-type stars**, such as Sirius, have surface temperatures between 7,500 and 10,000 Kelvin and are known for their white color. **F-type stars** are cooler than A-type stars, with surface temperatures between 6,000 and 7,500 Kelvin. They appear yellow-white and are less luminous than A-type stars. **G-type stars**, like our Sun, have surface temperatures between 5,500 and 6,000 Kelvin. These yellow dwarf stars are stable and long-lived, making them ideal for supporting life as we know it. **K-type stars**, or orange dwarfs, have surface temperatures between 3,500 and 5,500 Kelvin. They are cooler and less luminous than G-type stars but still stable enough to potentially support life. **M-type stars**, the coolest and least luminous of the main sequence stars, have surface temperatures below 3,500 Kelvin. These red dwarfs are the most common type of star in the galaxy but are too cool to support life as we understand it. In addition to spectral classification, stars can also be categorized by their evolutionary stage. **Main Sequence stars** are those that fuse hydrogen into helium in their cores and include all the spectral types mentioned above. **Red Giants** are stars that have exhausted their hydrogen fuel and expanded significantly, often becoming much cooler and more luminous. **White Dwarfs** are the remnants of stars that have shed their outer layers after exhausting their fuel sources, leaving behind a hot core that cools over time. **Neutron Stars** and **Black Holes** represent the end stages of more massive stars that undergo supernova explosions. Neutron stars are incredibly dense objects composed almost entirely of neutrons, while black holes are regions of space where gravity is so strong that nothing, including light, can escape once it falls within a certain boundary called the event horizon. Understanding these stellar types and categories provides a comprehensive framework for classifying the Sun and other stars, highlighting their unique characteristics and evolutionary paths. The Sun, as a G-type main sequence star, fits neatly into this classification system, offering insights into its life cycle and the conditions necessary for life to thrive on Earth.
2. Characteristics of Main Sequence Stars
Main Sequence stars, like our Sun, are characterized by several key attributes that define their lifecycle and behavior. One of the primary characteristics is their stable nuclear fusion process. These stars undergo a continuous process of converting hydrogen into helium in their cores, releasing a tremendous amount of energy in the form of light and heat. This process is what sustains their luminosity and surface temperature, making them shine steadily over millions to billions of years. Another crucial characteristic is their position on the Hertzsprung-Russell diagram, a graphical representation of stars' luminosities versus their surface temperatures. Main Sequence stars form a diagonal band on this diagram, known as the Main Sequence, where they are plotted according to their mass and evolutionary stage. The mass of a Main Sequence star is a determining factor in its properties. More massive stars have higher core pressures and temperatures, leading to faster fusion rates and thus higher luminosities. Conversely, less massive stars have lower core pressures and temperatures, resulting in slower fusion rates and lower luminosities. This mass dependency also influences their lifetimes; more massive stars burn through their hydrogen fuel more quickly, while less massive ones can live for billions of years. For instance, the Sun, with its mass approximately equal to one solar mass (M⊙), has a lifespan of about 10 billion years. Main Sequence stars also exhibit specific spectral types based on their surface temperatures. These spectral types range from O (the hottest) to M (the coolest), with the Sun classified as a G-type main-sequence star (G2V). This classification indicates that the Sun has a surface temperature around 5,500 degrees Celsius, which is optimal for supporting life on Earth. The spectral type further influences the star's color; hotter stars appear blue or white, while cooler ones appear red or orange. In addition to these intrinsic properties, Main Sequence stars can be distinguished by their evolutionary stage. They are in the middle phase of their lifecycle, having exhausted their protostar phase but not yet exhausted their hydrogen fuel. This stability allows them to maintain consistent energy output over long periods, making them ideal for supporting planetary systems with stable climates. Understanding these characteristics of Main Sequence stars provides valuable insights into the nature of our Sun and its role within our solar system. By recognizing that the Sun is a G-type main-sequence star, we can better appreciate its unique position in the universe and why it is capable of sustaining life on Earth. This understanding also helps in identifying other potentially habitable exoplanets orbiting similar stars elsewhere in the cosmos.
3. The Sun's Place in the Hertzsprung-Russell Diagram
The Sun's place in the Hertzsprung-Russell (H-R) diagram is a pivotal aspect of understanding its classification and role within the broader context of stellar evolution. The H-R diagram, a fundamental tool in astrophysics, plots stars according to their luminosity (or brightness) against their surface temperature. This graphical representation allows astronomers to categorize stars into various types based on these two critical parameters. The Sun, with a surface temperature of approximately 5,500 degrees Celsius (9,932 degrees Fahrenheit), falls into the category of main-sequence stars. On the H-R diagram, main-sequence stars form a diagonal band known as the main sequence, where the Sun is situated near the middle. This positioning indicates that the Sun is in the prime of its life, fusing hydrogen into helium in its core—a process that sustains its luminosity and temperature. In terms of luminosity, the Sun is classified as a G-type main-sequence star (G2V), meaning it is a medium-sized star with moderate energy output. Its luminosity is about 3.8 x 10^26 watts, which is relatively modest compared to more massive and luminous stars but sufficient to support life on Earth. The "G" designation signifies that the Sun's surface temperature places it among yellow dwarf stars, which are neither too hot nor too cool and are known for their stability over billions of years. Understanding the Sun's position on the H-R diagram also provides insights into its evolutionary stage. As a main-sequence star, the Sun has already burned through about half of its hydrogen fuel since its formation about 4.6 billion years ago. It will continue to fuse hydrogen for another approximately 5 billion years before exhausting this fuel source and evolving into a red giant phase. This knowledge helps astronomers predict future changes in the Sun's behavior and its impact on the solar system. In summary, the Sun's placement on the Hertzsprung-Russell diagram as a G-type main-sequence star underscores its unique characteristics and evolutionary status. This classification not only helps in understanding the Sun's current state but also provides a framework for predicting its future evolution and its role in sustaining life on Earth. By analyzing the Sun within this broader stellar context, scientists can better appreciate its significance and the conditions necessary for life to thrive in our solar system.
The Sun's Physical and Chemical Properties
The Sun, the central star of our solar system, is a fascinating celestial body that has captivated human curiosity for centuries. Its physical and chemical properties are crucial for understanding its role in sustaining life on Earth and its place within the broader universe. This article delves into three key aspects of the Sun: its mass, radius, and surface temperature; its composition and elemental abundance; and the process of energy production through nuclear fusion. By examining these fundamental characteristics, we gain a deeper insight into the Sun's structure and function. Understanding these properties not only enhances our knowledge of the Sun itself but also provides a foundation for classifying it within the context of stellar evolution and the broader classification of stars. This comprehensive exploration will ultimately lead us to a clearer understanding of the Sun's classification, shedding light on its unique position among other celestial bodies.
1. Mass, Radius, and Surface Temperature
The Sun, our solar system's central star, is characterized by several key physical properties that define its nature and behavior. Among these, mass, radius, and surface temperature are particularly significant. **Mass:** The Sun's mass is approximately 1.989 x 10^30 kilograms, which is about 330,000 times the mass of Earth. This immense mass is crucial because it determines the gravitational pull that holds the solar system together. The Sun's mass also influences its internal pressure and temperature, which in turn affect its energy output through nuclear fusion reactions in its core. **Radius:** The Sun's radius is about 696,000 kilometers, roughly 109 times larger than Earth's radius. This size is typical for a main-sequence star like the Sun, which is in the middle of its life cycle and fusing hydrogen into helium in its core. The radius affects the Sun's surface area and volume, impacting how much energy it can emit and how it interacts with the surrounding space. **Surface Temperature:** The surface temperature of the Sun, often referred to as the photosphere, is approximately 5,500 degrees Celsius (9,932 degrees Fahrenheit). This temperature is what we observe as sunlight and is a result of the energy generated by nuclear fusion in the core being radiated outward. The surface temperature is also indicative of the Sun's spectral type (G2V), which classifies it as a yellow dwarf star. This classification helps astronomers understand its luminosity, color, and evolutionary stage. Understanding these properties—mass, radius, and surface temperature—is essential for grasping the Sun's overall structure and function. They collectively influence the Sun's energy output, its impact on the planets, and its place within the broader context of stellar evolution. For instance, the Sun's mass ensures that it maintains a stable gravitational hold on the solar system, while its radius and surface temperature dictate how much solar energy is available to support life on Earth. These physical characteristics make the Sun an ideal star for supporting life as we know it, highlighting its unique role in our cosmic neighborhood.
2. Composition and Elemental Abundance
The Sun's composition and elemental abundance are crucial aspects of its physical and chemical properties, providing insights into its formation, evolution, and the broader context of stellar chemistry. The Sun is primarily composed of hydrogen and helium, with hydrogen making up approximately 75% by mass and helium about 25%. These light elements dominate the Sun's structure due to their abundance in the interstellar medium from which the Sun formed. The remaining 1% consists of heavier elements such as oxygen, carbon, nitrogen, iron, neon, magnesium, silicon, and sulfur, among others. These elements are produced through various nuclear processes within the Sun itself or inherited from the solar nebula. The elemental abundance in the Sun is often used as a standard reference for comparing the chemical composition of other stars and celestial objects. This is because the Sun's composition reflects the average elemental abundance of the solar system at the time of its formation about 4.6 billion years ago. The precise measurement of these abundances is achieved through spectroscopic analysis of the Sun's photosphere and corona, as well as through studies of solar wind and meteorites. Understanding the Sun's elemental composition is vital for several reasons. Firstly, it helps in modeling stellar evolution and nucleosynthesis processes that occur within stars. For instance, the abundance of certain elements like lithium and beryllium can provide clues about the Sun's internal mixing processes and its age. Secondly, it aids in the study of planetary formation and the delivery of volatile elements to terrestrial planets like Earth. The presence of certain elements in the Sun also influences its energy output and spectral characteristics, which in turn affect Earth's climate and habitability. Furthermore, comparing the Sun's elemental abundance with that of other stars can reveal insights into the chemical evolution of the galaxy. Stars with different metallicities (abundances of elements heavier than hydrogen and helium) reflect different epochs of star formation and the enrichment history of the interstellar medium. This comparative study is essential for understanding how stars like the Sun contribute to the overall chemical enrichment of galaxies over cosmic time scales. In summary, the composition and elemental abundance of the Sun are fundamental to understanding its internal dynamics, its role in the solar system, and its place within the broader context of stellar astrophysics. These properties not only define the Sun's current state but also offer a window into its past and future evolution, as well as the evolutionary history of our galaxy.
3. Energy Production through Nuclear Fusion
Nuclear fusion, the process that powers the Sun, is a fundamental aspect of its physical and chemical properties. This energy production mechanism involves the fusion of atomic nuclei to form a heavier nucleus, releasing a significant amount of energy in the process. In the Sun, this occurs primarily through the proton-proton chain reaction and the CNO cycle. The proton-proton chain reaction is the dominant process, where hydrogen nuclei (protons) fuse to form helium nuclei, releasing positrons, neutrinos, and gamma rays. This chain of reactions begins with the collision of two protons to form deuterium, which then combines with another proton to produce helium-3. Finally, two helium-3 nuclei combine to form helium-4 and two protons. The CNO cycle, though less prevalent, also contributes to the Sun's energy output by involving carbon, nitrogen, and oxygen as catalysts in the fusion process. These cycles take place in the Sun's core, where temperatures exceed 15 million degrees Celsius and pressures are incredibly high, providing the necessary conditions for nuclear fusion to occur. The energy generated from these reactions is what sustains the Sun's luminosity and supports life on Earth by providing the solar radiation that drives our climate and ecosystems. Understanding nuclear fusion in the context of the Sun's properties highlights its importance in stellar evolution and the broader universe. Stars like the Sun are classified as main-sequence stars, meaning they are in the middle of their life cycle and derive their energy from nuclear fusion. This process not only powers the Sun but also determines its mass, size, and surface temperature, all of which are critical factors in defining its place within the Hertzsprung-Russell diagram—a tool used to classify stars based on their luminosity and temperature. Moreover, studying nuclear fusion in stars like the Sun has significant implications for human endeavors. For instance, replicating this process on Earth could provide a nearly limitless source of clean energy, a goal being pursued in various fusion research projects around the world. While achieving controlled nuclear fusion remains a technological challenge, understanding its role in the Sun's energy production underscores its potential as a future energy solution. In summary, nuclear fusion is the cornerstone of the Sun's energy production, driving its luminosity and influencing its physical and chemical properties. This process is not only essential for understanding stellar dynamics but also holds promise for advancing human technology and addressing global energy needs. As we continue to explore and study the Sun, we gain deeper insights into the fundamental processes that govern our universe and inspire new avenues for scientific discovery and innovation.
Comparing the Sun to Other Stars
In the vast expanse of the universe, stars come in a myriad of sizes, luminosities, and evolutionary stages, each with its own unique characteristics. The Sun, our closest and most studied star, is often the benchmark against which other stars are compared. To fully appreciate the Sun's place in the cosmos, it is essential to compare it to other celestial bodies. This article delves into three key areas of comparison: the Sun's size and luminosity relative to other stars, its lifespan and evolutionary stage, and its unique features that set it apart from similar stars. By examining these aspects, we gain a deeper understanding of the Sun's classification within the broader context of stellar astronomy. This comparative analysis not only highlights the Sun's distinctiveness but also underscores its significance in our solar system, ultimately leading to a more nuanced understanding of the Sun's classification and its role in the universe.
1. Size and Luminosity Relative to Other Stars
When comparing the Sun to other stars, its size and luminosity stand out as significant factors that define its place within the vast celestial landscape. The Sun is classified as a G-type main-sequence star, often referred to as a G2V star. In terms of size, the Sun is neither exceptionally large nor small; it falls squarely in the middle range. With a radius of approximately 696,000 kilometers, it is about 109 times larger than Earth but dwarfed by the behemoths known as red giants and supergiants. For instance, the red supergiant VY Canis Majoris has a radius that could encompass the entire orbit of Saturn around the Sun. Luminosity is another critical aspect where the Sun's characteristics become evident. The Sun emits about 3.8 x 10^26 watts of power, which is moderate compared to other stars. This energy output is what makes life on Earth possible, providing the necessary warmth and light for photosynthesis and other biological processes. In contrast, blue giants like R136a1 in the Large Magellanic Cloud are thousands of times more luminous than the Sun, while red dwarfs like Proxima Centauri emit only a fraction of the Sun's energy. The Sun's luminosity also places it in a stable phase of its life cycle; it has already burned through about half of its hydrogen fuel since its formation about 4.6 billion years ago and will continue to shine for another 5 billion years before exhausting its fuel and expanding into a red giant. The balance between size and luminosity makes the Sun an ideal candidate for supporting life on planets within its habitable zone. Stars that are too large or too luminous tend to have shorter lifetimes and may not provide stable conditions for life to evolve over billions of years. Conversely, smaller and less luminous stars may not emit enough energy to sustain complex life forms. The Sun's moderate size and luminosity thus position it as a "Goldilocks" star—neither too big nor too small, but just right for fostering life on Earth. Understanding these characteristics not only helps us appreciate the uniqueness of our solar system but also informs our search for exoplanets and potentially habitable worlds elsewhere in the universe. By comparing the Sun to other stars, scientists can better identify which stars might host planets with conditions similar to those of Earth, thereby narrowing down the search for extraterrestrial life. This comparative analysis underscores the importance of the Sun's size and luminosity in making our planet a haven for life amidst an otherwise vast and diverse stellar landscape.
2. Lifespan and Evolutionary Stage
The lifespan and evolutionary stage of a star are crucial factors in understanding its place within the universe, particularly when comparing the Sun to other stars. The Sun, a G-type main-sequence star, is currently in its prime, having already burned through about half of its hydrogen fuel since its formation approximately 4.6 billion years ago. This stage is characterized by the stable fusion of hydrogen into helium in its core, a process that maintains a balance between the inward pull of gravity and the outward pressure from nuclear reactions. This equilibrium allows the Sun to sustain life on Earth and other planets within its habitable zone. In contrast, other stars exhibit diverse lifespans and evolutionary stages. Red dwarf stars, for instance, are the most common type of star in the galaxy and have much longer lifespans than the Sun—often exceeding 100 billion years. These stars burn their hydrogen fuel very slowly due to their lower masses, which means they can sustain life-supporting conditions for an extended period. On the other end of the spectrum, massive blue giant stars have extremely short lifespans, typically ranging from a few million to tens of millions of years. These stars burn through their fuel rapidly due to their high masses and intense nuclear reactions, leading them to evolve quickly through various stages such as red supergiants before ending their lives in spectacular supernovae explosions. The evolutionary stage of a star also influences its impact on surrounding planets. For example, as the Sun ages and exhausts its hydrogen fuel, it will expand into a red giant in about 5 billion years, potentially engulfing the inner planets including Earth. This phase marks a significant transition where the star's outer layers swell while its core contracts and heats up, eventually shedding its outer layers to form a planetary nebula and leaving behind a white dwarf remnant. In contrast, stars like red dwarfs may never reach this stage due to their slow evolution. Understanding these differences in lifespan and evolutionary stages provides valuable insights into the unique characteristics of the Sun compared to other stars. While the Sun's relatively moderate size and stable main-sequence phase support life as we know it, other stars offer varying conditions that could either foster or hinder life elsewhere in the universe. This comparison underscores the importance of studying stellar evolution to better comprehend the broader cosmic context in which our solar system exists.
3. Unique Features of the Sun Compared to Similar Stars
The Sun, our solar system's central star, exhibits several unique features that distinguish it from other similar stars. One of the most notable characteristics is its relatively stable energy output. Unlike many other stars, which can experience significant variations in brightness due to internal dynamics or external influences, the Sun maintains a remarkably consistent luminosity. This stability is crucial for life on Earth, as it ensures a steady supply of solar energy that supports our planet's climate and biological processes. In contrast, some stars like Delta Scuti variables can fluctuate in brightness by as much as 10% over short periods, making them less hospitable to life. Another unique feature of the Sun is its position within the main sequence stage of stellar evolution. The main sequence is the phase where stars fuse hydrogen into helium in their cores, and the Sun is right in the middle of this stage. This means it has already burned through about half of its hydrogen fuel since its formation about 4.6 billion years ago but still has enough to sustain life for another 5 billion years. Many other stars, such as red dwarfs or blue giants, are either at earlier or later stages of their life cycles, making them less conducive to supporting life as we know it. Lastly, the Sun's metallicity—a measure of the abundance of elements heavier than hydrogen and helium—is another distinguishing factor. With a metallicity slightly higher than that of the average star in the Milky Way galaxy, the Sun has a richer composition of heavier elements. This higher metallicity is thought to be a result of being born in a region of the galaxy where previous generations of stars had already enriched the interstellar medium with these elements. This enrichment is essential for planetary formation and the development of complex life forms because heavier elements are necessary for creating diverse planetary environments and biological systems. These unique features—stable energy output, mid-main sequence status, and higher metallicity—collectively make the Sun an ideal star for supporting life on Earth. While other stars may share some of these characteristics, the combination and specific values of these traits in our Sun are particularly favorable for sustaining life as we understand it. This highlights why Earth's position in the universe is often referred to as a "Goldilocks zone" not just in terms of distance from the Sun but also in terms of having a star with just the right properties to nurture life.