What Does A Resonator Do
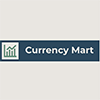
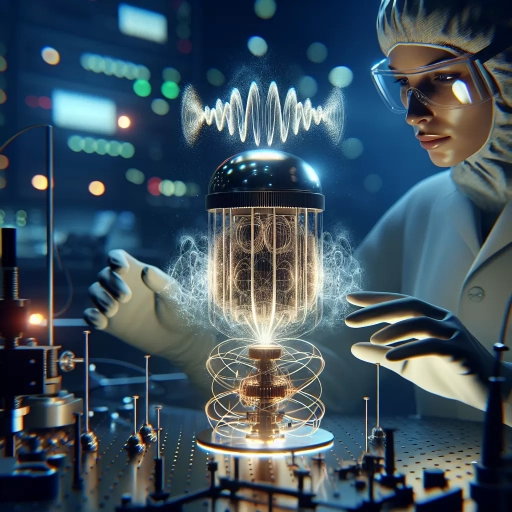
In the realm of physics and engineering, resonators play a crucial role in amplifying and stabilizing vibrations or oscillations, making them indispensable components in various technological applications. A resonator is essentially a device or system that resonates at specific frequencies, enhancing the efficiency and precision of numerous processes. To fully grasp the significance of resonators, it is essential to delve into their fundamental principles, explore their diverse applications across different fields, and understand the technical mechanisms behind their operation. This article will guide you through these key aspects, starting with **Understanding the Basics of a Resonator**, where we will uncover the core concepts that define how resonators function. From there, we will examine **Applications of Resonators in Various Fields**, highlighting their critical roles in fields such as electronics, acoustics, and medical technology. Finally, **How Resonators Work: Technical Insights** will provide a detailed look into the technical aspects that make resonators so effective. By the end of this journey, you will have a comprehensive understanding of what a resonator does and why it is such a vital component in modern technology. Let's begin by **Understanding the Basics of a Resonator**.
Understanding the Basics of a Resonator
Understanding the basics of a resonator is crucial for grasping its fundamental role in various technological and scientific applications. A resonator, at its core, is a device or system that vibrates or oscillates at specific frequencies, often used to amplify or filter signals. To delve into the intricacies of resonators, it is essential to explore three key aspects: their definition and function, the diverse types of resonators available, and their historical development. Firstly, understanding the definition and function of a resonator provides the foundational knowledge necessary for appreciating its utility. This involves recognizing how resonators operate within different contexts, such as in electronic circuits, mechanical systems, or even musical instruments. Secondly, examining the various types of resonators reveals the versatility and adaptability of these devices. From LC circuits in electronics to acoustic resonators in music, each type serves unique purposes and offers distinct advantages. Lastly, tracing the historical development of resonators highlights the evolutionary journey from simple mechanical devices to sophisticated modern technologies. This historical perspective underscores the continuous innovation and refinement that have shaped our understanding and application of resonators. By starting with a clear definition and function, we can better appreciate the broader landscape of resonator technology and its multifaceted applications. Let us begin by exploring the definition and function of a resonator in more detail.
Definition and Function
In the realm of physics and engineering, a resonator is a crucial component that plays a pivotal role in various systems, from musical instruments to electronic circuits. At its core, a resonator is defined as a device or structure that vibrates or oscillates at specific frequencies, often referred to as resonant frequencies. These frequencies are inherent to the physical properties of the resonator itself, such as its mass, stiffness, and dimensions. The primary function of a resonator is to amplify or enhance the energy at these resonant frequencies while dampening or rejecting energy at other frequencies. This selective amplification is what makes resonators indispensable in numerous applications. For instance, in musical instruments like guitars and violins, the body of the instrument acts as a resonator, amplifying the vibrations of the strings to produce a richer and fuller sound. Similarly, in electronic circuits, resonators such as LC circuits (comprising inductors and capacitors) are used to filter signals, allowing only those at the resonant frequency to pass through while rejecting others. The principle behind this functionality lies in the concept of resonance itself. When an external force is applied to a resonator at its resonant frequency, it causes the system to oscillate with maximum amplitude. This phenomenon is due to the synchronization between the external force and the natural frequency of the system, leading to efficient energy transfer and amplification. In practical terms, this means that a resonator can be tuned to specific frequencies, making it highly versatile across different fields. For example, in telecommunications, resonators are used in filters and antennas to ensure that signals are transmitted and received efficiently at the desired frequencies. In medical imaging technologies like MRI (Magnetic Resonance Imaging), hydrogen nuclei in the body act as tiny resonators that respond to magnetic fields at specific frequencies, allowing for detailed imaging of internal structures. Moreover, resonators are not limited to mechanical or electrical systems; they can also be found in optical systems. Optical resonators, such as Fabry-Pérot cavities, consist of mirrors that reflect light back and forth, creating standing waves at particular wavelengths. These devices are crucial in lasers and spectroscopy, enabling precise control over light frequencies. Understanding the basics of a resonator is essential for designing and optimizing systems that rely on frequency-specific operations. By leveraging the inherent properties of materials and structures to create resonant conditions, engineers can develop more efficient, precise, and powerful technologies. Whether it's enhancing sound quality in music or ensuring accurate signal transmission in communication networks, the role of a resonator is fundamental and multifaceted, making it a cornerstone in various scientific and engineering disciplines.
Types of Resonators
Resonators are fundamental components in various engineering and scientific applications, and understanding their types is crucial for harnessing their full potential. A resonator is essentially a device or system that exhibits resonance, meaning it vibrates or oscillates at a specific frequency, often with minimal damping. There are several types of resonators, each tailored to specific needs and environments. **Mechanical Resonators** are among the most intuitive forms, where physical structures like springs, pendulums, or tuning forks resonate at characteristic frequencies. These are commonly used in clocks, musical instruments, and vibration isolation systems. For instance, a guitar string is a mechanical resonator that vibrates at specific frequencies to produce sound. **Electrical Resonators**, on the other hand, involve circuits that resonate due to the interaction between inductive and capacitive components. The most common type is the LC circuit (inductor-capacitor circuit), which is pivotal in radio transmitters and receivers for tuning into specific frequencies. These circuits can be found in everything from simple AM radios to sophisticated radar systems. **Optical Resonators** play a critical role in photonics and laser technology. These include optical cavities where light is confined and resonates between mirrors or other reflective surfaces. Lasers rely on optical resonators to amplify light through stimulated emission, while optical fiber resonators are used in telecommunications for signal processing and filtering. **Acoustic Resonators** deal with sound waves and are essential in audio engineering. Helmholtz resonators, for example, are used in musical instruments like guitars and violins to enhance certain frequencies. In architectural acoustics, acoustic resonators help in designing concert halls and recording studios to optimize sound quality. **Nuclear Resonators** involve the interaction of nuclear particles and are crucial in nuclear magnetic resonance (NMR) spectroscopy and magnetic resonance imaging (MRI). Here, nuclei resonate when exposed to specific magnetic fields, providing detailed information about molecular structures and tissue composition. **Piezoelectric Resonators** leverage the piezoelectric effect, where certain materials generate an electric charge in response to mechanical stress. These are widely used in sensors, actuators, and energy harvesting devices due to their ability to convert mechanical energy into electrical energy efficiently. Each type of resonator has unique characteristics and applications, but they all share the common trait of amplifying or filtering signals at specific frequencies. Understanding these different types is essential for engineers and scientists to design and optimize systems across various fields, from electronics and mechanics to optics and acoustics. By selecting the appropriate resonator type, one can achieve precise control over frequency responses, leading to improved performance and efficiency in a wide range of technological applications.
Historical Development
The historical development of resonators is a fascinating narrative that spans centuries, reflecting the evolution of scientific understanding and technological innovation. The concept of resonance itself has ancient roots, with early civilizations observing and utilizing natural phenomena such as the resonance of strings in musical instruments. However, the systematic study and application of resonators began to take shape during the Renaissance period. In the 17th century, scientists like Galileo Galilei and Christiaan Huygens laid foundational work on the principles of vibration and oscillation, which are central to the functioning of resonators. Their discoveries about pendulums and springs provided the groundwork for later advancements. The 19th century saw significant strides with the work of Hermann von Helmholtz, who extensively studied sound waves and their resonance properties. His book "On the Sensations of Tone" (1863) remains a seminal work in acoustics. The early 20th century witnessed a surge in the development of electronic resonators, particularly with the invention of the quartz crystal oscillator by Joseph W. Horton and Warren A. Marrison in 1920. This innovation revolutionized timekeeping and communication technologies by providing highly stable frequency sources. The advent of radio communication further accelerated research into resonant circuits, leading to the development of tuned circuits that could select specific frequencies from a broad spectrum. In the mid-20th century, advancements in materials science led to the creation of more sophisticated resonators. The discovery of piezoelectric materials, which generate an electric charge in response to mechanical stress, enabled the development of piezoelectric resonators. These devices found applications in ultrasonic cleaning, medical imaging, and even space exploration. The latter half of the 20th century saw significant advancements in microelectromechanical systems (MEMS) technology, allowing for the miniaturization of resonators. This led to their integration into consumer electronics such as smartphones and GPS devices, where they play crucial roles in signal processing and frequency stabilization. Today, resonators continue to evolve with cutting-edge technologies like nanotechnology and quantum mechanics. Researchers are exploring new materials and designs that can operate at higher frequencies or under extreme conditions, opening up possibilities for applications in fields ranging from telecommunications to quantum computing. Understanding these historical developments is essential for appreciating the complexity and versatility of modern resonators. From their humble beginnings in ancient musical instruments to their current roles in advanced technologies, resonators have come a long way. Their ability to amplify or filter specific frequencies makes them indispensable components in a wide array of devices, underscoring their importance in our increasingly interconnected world. By grasping the historical context behind these devices, one can better comprehend their fundamental principles and appreciate their multifaceted applications across various disciplines.
Applications of Resonators in Various Fields
Resonators, devices that amplify or filter specific frequencies, have far-reaching applications across diverse fields, each leveraging their unique properties to enhance performance and efficiency. In **Electrical and Electronic Systems**, resonators play a crucial role in filtering signals, stabilizing frequencies, and improving the overall quality of electronic circuits. They are integral in the design of antennas, oscillators, and filters, ensuring precise signal transmission and reception. Moving beyond electronics, **Acoustic and Musical Instruments** rely on resonators to produce rich, resonant sounds. From the body of a guitar to the chambers of a pipe organ, these devices amplify and shape sound waves, contributing to the distinctive timbres of various instruments. Additionally, **Medical and Scientific Research** benefit from resonators in imaging technologies like MRI machines and in the study of molecular structures. These applications highlight the versatility and importance of resonators in modern technology. As we delve into the specifics, we will first explore how resonators are indispensable in **Electrical and Electronic Systems**.
Electrical and Electronic Systems
Electrical and electronic systems are the backbone of modern technology, enabling a wide range of applications from simple household appliances to complex industrial control systems. At the heart of these systems often lie resonators, which play a crucial role in filtering, amplifying, and stabilizing signals. A resonator is essentially a device that vibrates or oscillates at a specific frequency, known as its resonant frequency. This property allows resonators to selectively enhance or filter out signals based on their frequency, making them indispensable in various fields. In telecommunications, resonators are used in filters to ensure that only the desired frequency bands are transmitted or received. For instance, in radio communication systems, resonant circuits help tune into specific radio frequencies, allowing clear reception of signals while rejecting noise and interference. Similarly, in mobile phones, resonators are integral to the design of antennas and filters, ensuring efficient transmission and reception of data. In medical technology, resonators find application in MRI (Magnetic Resonance Imaging) machines. Here, powerful magnetic fields and radio waves are used to create detailed images of the body's internal structures. The resonant properties of hydrogen nuclei in the body are exploited to generate these images, making MRI a non-invasive diagnostic tool with high resolution. In the field of energy and power systems, resonators are used in power transmission lines to improve efficiency. For example, series compensators in high-voltage transmission lines use resonant circuits to compensate for line inductance, thereby enhancing power transfer capabilities and reducing energy losses. In consumer electronics, such as audio equipment and musical instruments, resonators are used to enhance sound quality. Guitar pickups and speakers often incorporate resonant circuits to amplify specific frequency ranges, contributing to the rich tonal characteristics of musical instruments. Moreover, in automotive systems, resonators are employed in exhaust systems to reduce noise pollution. By creating a resonant cavity that cancels out certain frequencies of engine noise, these devices help in complying with noise regulations while improving overall vehicle performance. In aerospace engineering, resonators are critical components in navigation systems like GPS receivers. Here, they help filter out unwanted signals and noise, ensuring accurate positioning and timing information even in challenging environments. Lastly, in scientific research, resonators are used in particle accelerators and spectroscopy instruments. For instance, cavity resonators in particle accelerators help accelerate charged particles to high speeds by creating electromagnetic fields that resonate at specific frequencies. In summary, resonators are versatile components that leverage their ability to oscillate at specific frequencies to enhance signal quality, filter noise, and stabilize systems across diverse fields. Their applications span from everyday consumer electronics to advanced medical imaging and cutting-edge scientific research, underscoring their importance in modern electrical and electronic systems.
Acoustic and Musical Instruments
Acoustic and musical instruments are quintessential examples of the practical application of resonators, showcasing how these devices amplify and enhance sound quality. In the realm of music, resonators play a crucial role in the design and functionality of various instruments. For instance, stringed instruments like guitars and violins rely on their bodies to act as resonators. The hollow body of these instruments amplifies the vibrations produced by the strings, projecting a richer and fuller sound to the listener. Similarly, wind instruments such as flutes and clarinets utilize the principle of resonance within their chambers to produce distinct tones and timbres. The shape, size, and material of these chambers determine the resonant frequencies, allowing musicians to achieve a wide range of musical notes. In percussion instruments like drums, the drumhead acts as a resonator when struck, producing a resonant sound that is amplified by the drum's body. This principle is also evident in brass instruments where the length and shape of the tubing create specific resonant frequencies that correspond to different musical notes. The human voice itself can be considered a biological resonator; the vocal tract acts as a resonating chamber that modifies the sound produced by the vocal cords, enabling us to produce a variety of tones and pitches. The application of resonators in musical instruments extends beyond traditional acoustic instruments. Electronic instruments such as synthesizers and electric guitars also employ resonators to enhance their sound. For example, electric guitars use pickups that capture the vibrations of the strings and amplify them through an electronic circuit, which often includes resonant filters to shape the tone. Synthesizers, on the other hand, generate sound electronically but can mimic the resonant characteristics of acoustic instruments through digital signal processing. Moreover, modern music production heavily relies on resonators in the form of audio effects processors. Reverb units, for instance, simulate the natural resonance of different environments (like concert halls or small rooms) to add depth and ambiance to recorded sounds. Equalizers and filters are also used to adjust the resonant frequencies of recorded tracks, allowing producers to fine-tune the sound quality and balance. In addition to their role in music-making, acoustic and musical instruments serve as educational tools for understanding the principles of physics and acoustics. They illustrate how resonant frequencies can be manipulated to produce desired sounds, teaching musicians and engineers about the intricate relationship between vibration, frequency, and sound quality. Overall, the integration of resonators in acoustic and musical instruments highlights their critical function in enhancing sound production across various genres and types of music. Whether it's a traditional acoustic instrument or a modern electronic device, the underlying principle of resonance remains essential for creating rich, vibrant sounds that captivate audiences worldwide. This underscores the broader significance of resonators not just as technical components but as integral elements that shape our musical experiences.
Medical and Scientific Research
In the realm of medical and scientific research, resonators play a pivotal role in advancing our understanding and treatment of various diseases. These devices, which amplify specific frequencies, are integral to several cutting-edge technologies. For instance, in medical imaging, resonators are crucial components of Magnetic Resonance Imaging (MRI) machines. Here, they help generate the precise magnetic fields necessary for aligning hydrogen nuclei in the body, allowing for detailed images that aid in diagnosing conditions ranging from neurological disorders to cancer. Additionally, resonators are used in spectroscopy techniques such as Nuclear Magnetic Resonance (NMR) spectroscopy, which is essential for analyzing the structure and properties of molecules. This is particularly valuable in drug discovery and development, where understanding the molecular interactions is key to creating effective treatments. In scientific research, resonators are employed in particle accelerators to accelerate charged particles to incredibly high speeds, facilitating experiments that uncover the fundamental nature of matter and energy. The Large Hadron Collider, for example, relies on sophisticated resonator systems to achieve the precise conditions necessary for collisions that reveal insights into subatomic particles and forces. Furthermore, optical resonators are used in laser technology, enabling the production of high-intensity beams that are vital for applications such as material processing and spectroscopic analysis. These lasers also find applications in medical procedures like eye surgery and skin treatments, where precision and control are paramount. Moreover, resonators are critical in the field of quantum computing and quantum communication. Quantum resonators, such as superconducting qubits, form the basis of quantum processors that promise to revolutionize computing by solving complex problems exponentially faster than classical computers. In quantum communication, optical resonators are used to enhance the sensitivity of detectors in quantum key distribution systems, ensuring secure data transmission over long distances. The versatility of resonators extends to environmental monitoring as well. Acoustic resonators can detect subtle changes in ocean currents and temperature, providing valuable data for climate studies and marine conservation efforts. Similarly, mechanical resonators are used in seismology to measure seismic waves, helping scientists understand Earth's internal structure and predict seismic activity more accurately. In summary, resonators are indispensable tools across various fields of medical and scientific research. Their ability to amplify specific frequencies makes them crucial for advanced imaging techniques, spectroscopic analysis, particle acceleration, laser technology, quantum computing, and environmental monitoring. As technology continues to evolve, the role of resonators will only become more significant, driving innovation and discovery in these critical areas of study.
How Resonators Work: Technical Insights
Resonators are fundamental components in various technological and scientific applications, from electronic circuits to musical instruments. Understanding how resonators work involves delving into three key aspects: the principle of resonance, the components and design of resonators, and the mechanisms of energy transfer and amplification. At the heart of any resonator lies the principle of resonance, which dictates how these devices respond to specific frequencies. This principle is crucial because it explains why resonators can amplify or filter signals with such precision. However, to fully appreciate this phenomenon, one must also consider the components and design of resonators, which include materials, geometries, and structural elements that influence their resonant properties. Additionally, grasping how energy is transferred and amplified within these systems is essential for optimizing their performance. By exploring these interconnected concepts, we can gain a comprehensive understanding of how resonators operate and why they are so vital in modern technology. Let's begin by examining the foundational principle of resonance, which underpins all other aspects of resonator functionality.
Principle of Resonance
The Principle of Resonance is a fundamental concept in physics that underpins the operation of resonators, which are crucial components in various technological and natural systems. Resonance occurs when a system vibrates or oscillates at a specific frequency, known as its resonant frequency, in response to an external force. This phenomenon is characterized by the system's ability to absorb and store energy efficiently at this particular frequency, leading to amplified oscillations. To understand how resonance works, consider a simple pendulum. When pushed gently at its natural frequency, the pendulum swings with increasing amplitude over time due to the efficient transfer of energy. Similarly, in electrical circuits, resonant frequencies are achieved when the inductive and capacitive reactances are equal, resulting in maximum current flow and minimal impedance. This principle is exploited in LC circuits (inductor-capacitor circuits) where the combination of inductors and capacitors creates a resonant circuit that can filter or amplify signals at specific frequencies. In mechanical systems, resonance can be observed in structures like bridges or buildings. For instance, if soldiers marching across a bridge synchronize their footsteps with the bridge's natural frequency, it can lead to catastrophic consequences due to the amplified vibrations. However, when harnessed correctly, mechanical resonance is used in devices such as tuning forks and guitar strings to produce clear tones. The principle of resonance also plays a critical role in quantum mechanics and particle physics. Quantum resonators, such as those found in atomic and molecular systems, exhibit resonant behavior when they absorb or emit energy at specific frequencies corresponding to their energy levels. This concept is essential for understanding phenomena like nuclear magnetic resonance (NMR) and electron spin resonance (ESR), which are pivotal in fields ranging from medical imaging to materials science. In practical applications, resonators are designed to operate at precise frequencies to achieve desired outcomes. For example, radio antennas are tuned to resonate at the frequency of the radio waves they are intended to receive or transmit. Similarly, quartz crystal oscillators in electronic devices use the resonant properties of quartz crystals to generate stable clock signals. Understanding the principle of resonance is crucial for designing efficient and reliable systems across various domains. It allows engineers to optimize performance by matching the operating frequency of a system with its natural resonant frequency, thereby maximizing energy transfer and minimizing losses. This technical insight into resonance not only enhances our comprehension of how resonators work but also underscores the importance of this fundamental principle in modern technology and scientific inquiry. By leveraging resonance effectively, we can create more sophisticated and efficient systems that drive innovation and advancement in multiple fields.
Components and Design
In the intricate world of resonators, the components and design play a pivotal role in determining their performance and functionality. A resonator, fundamentally, is a device that vibrates or oscillates at a specific frequency, often used in various applications such as filters, oscillators, and sensors. The core components of a resonator include the resonant element, which can be mechanical (like a tuning fork), electrical (like an LC circuit), or even optical (such as a Fabry-Pérot cavity). For mechanical resonators, the design typically involves a mass-spring system where the mass represents the inertial component and the spring represents the elastic component. These elements are carefully tuned to achieve resonance at a desired frequency. In contrast, electrical resonators rely on inductive and capacitive elements. The LC circuit, for instance, consists of an inductor (L) and a capacitor (C) connected in series or parallel. The values of L and C are precisely calculated to ensure that the circuit resonates at the desired frequency, given by the formula \( f = \frac{1}{2\pi \sqrt{LC}} \). The design of resonators also involves careful consideration of damping factors to ensure that the oscillations are sustained without excessive energy loss. In some cases, active components like amplifiers may be integrated to compensate for energy losses and maintain stable oscillations. Additionally, the material selection for the resonant elements is crucial; materials with high Q-factors (quality factors) are preferred as they minimize energy dissipation and maximize the purity of the resonant frequency. Modern resonator designs often incorporate advanced materials and technologies. For example, quartz crystal resonators are widely used in electronic devices due to their high stability and low drift over temperature changes. Similarly, microelectromechanical systems (MEMS) technology has enabled the development of miniaturized resonators with improved precision and reliability. The geometric design of resonators is another critical aspect. For instance, in optical resonators like ring resonators or photonic crystals, the precise arrangement of the optical path and the refractive indices of the materials involved are essential for achieving the desired resonant behavior. These designs leverage principles from waveguide theory and optical physics to confine light within specific regions, enhancing the interaction between light and matter. In summary, the components and design of resonators are meticulously engineered to optimize their performance across various domains. Whether it's the precise tuning of mechanical or electrical elements, the selection of high-quality materials, or the innovative use of advanced technologies, each aspect contributes to the robust functionality and reliability of resonators in diverse applications. Understanding these intricacies is essential for appreciating how resonators work and their critical role in modern technology.
Energy Transfer and Amplification
Energy transfer and amplification are fundamental principles that underpin the operation of resonators, which are crucial components in various technological applications. At its core, a resonator is designed to efficiently transfer and amplify energy by exploiting the natural frequency of a system. This process begins with the concept of resonance, where the system vibrates or oscillates at a specific frequency, often referred to as its resonant frequency. When energy is introduced into the system at this frequency, it aligns perfectly with the system's natural oscillations, leading to a significant increase in amplitude. This amplification occurs because the energy input is synchronized with the system's inherent dynamics, allowing it to build up over time without being dissipated. The mechanism of energy transfer in resonators involves a delicate balance between the input energy and the system's damping characteristics. Damping refers to the loss of energy due to friction or other resistive forces within the system. In an ideal resonator, damping is minimized to ensure that the majority of the input energy is converted into useful oscillations rather than being lost as heat or other forms of dissipation. This optimization allows for maximum energy transfer efficiency, enabling the resonator to achieve higher amplitudes with minimal input. One of the key technical insights into how resonators work lies in their ability to store energy in a cyclic manner. For instance, in mechanical resonators like pendulums or springs, potential energy is converted into kinetic energy and vice versa during each cycle of oscillation. Similarly, in electrical resonators such as LC circuits (comprising inductors and capacitors), electrical energy is stored alternately in the magnetic field of the inductor and the electric field of the capacitor. This cyclic storage and release of energy enhance the overall efficiency of the resonator by ensuring that the input energy is utilized effectively. Furthermore, resonators can be tuned to specific frequencies by adjusting their physical parameters or components. For example, in an LC circuit, changing the values of the inductor or capacitor can alter the resonant frequency. This tunability makes resonators versatile tools across various fields such as telecommunications (where they are used in filters and antennas), medical imaging (like MRI machines), and even musical instruments (where they enhance sound quality). In addition to their role in amplifying signals, resonators also play a critical role in filtering out unwanted frequencies. By selectively amplifying signals at their resonant frequency while attenuating others, resonators act as narrowband filters that improve signal-to-noise ratios and overall system performance. This selective amplification is particularly important in applications where precise frequency discrimination is required. In conclusion, the principles of energy transfer and amplification are central to understanding how resonators function. By leveraging resonance to align with a system's natural frequency, resonators efficiently amplify input energy while minimizing losses due to damping. Their ability to store and release energy cyclically, combined with their tunability and filtering capabilities, makes them indispensable components in a wide range of technological applications. Understanding these technical insights into resonator operation provides a deeper appreciation for their role in enhancing performance across diverse fields.