What Is Dcpp
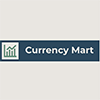
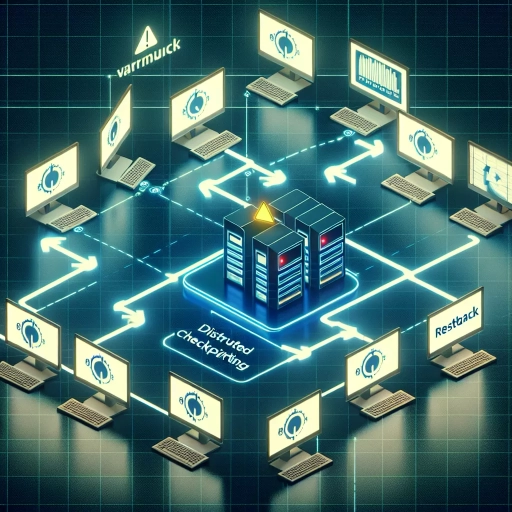
In the realm of modern technology, Distributed Checkpointing and Recovery Protocol (DCPP) stands out as a crucial component in ensuring the reliability and efficiency of distributed systems. This innovative protocol is designed to manage and recover from failures in complex network environments, making it an essential tool for maintaining system integrity. To fully appreciate the significance of DCPP, it is important to delve into its fundamental principles, key components, and real-world applications. Understanding the basics of DCPP provides a foundational knowledge of how this protocol operates, including its mechanisms for checkpointing and recovery. Key components and features of DCPP highlight its robust design and adaptability in various scenarios. Finally, exploring the applications and impact of DCPP reveals its practical value in enhancing system resilience and performance. By grasping these aspects, one can gain a comprehensive insight into the role of DCPP in contemporary computing. Let us begin by understanding the basics of DCPP, which forms the cornerstone of this protocol's functionality.
Understanding the Basics of DCPP
Understanding the basics of DCPP (Data Center Power and Cooling) is crucial for anyone involved in the design, operation, or management of data centers. This complex field encompasses a variety of critical components that ensure the efficient and reliable functioning of these facilities. To delve into the intricacies of DCPP, it is essential to explore three key areas: the definition and acronym expansion, historical context and development, and primary objectives and goals. Firstly, grasping the definition and expansion of the DCPP acronym sets the foundation for understanding its scope and significance. This involves breaking down what each term means within the context of data center operations. Secondly, examining the historical context and development of DCPP provides insight into how this field has evolved over time, driven by technological advancements and increasing demand for data storage and processing. Lastly, understanding the primary objectives and goals of DCPP highlights its importance in ensuring energy efficiency, reliability, and cost-effectiveness in data center operations. By exploring these aspects, one can gain a comprehensive understanding of DCPP's role in modern computing infrastructure. Let's begin by defining what DCPP stands for and what each component entails, setting the stage for a deeper dive into its historical development and core objectives.
Definition and Acronym Expansion
**Understanding the Basics of DCPP: Definition and Acronym Expansion** To delve into the intricacies of DCPP (Data Center Power and Cooling), it is essential to start with a clear understanding of its core components. DCPP stands for Data Center Power and Cooling, a critical aspect of data center operations that ensures the efficient and reliable functioning of IT infrastructure. The term "Data Center" refers to a facility used to house computer systems and associated components, such as telecommunications and storage systems. "Power" encompasses the electrical systems that supply energy to these components, including power distribution units (PDUs), uninterruptible power supplies (UPS), and generators. "Cooling" involves the mechanisms designed to maintain optimal operating temperatures within the data center, including air conditioning systems, liquid cooling solutions, and heat management technologies. The acronym DCPP highlights the interdependence of these two elements: power and cooling. Efficient power management is crucial because it directly affects the operational costs and reliability of the data center. Similarly, effective cooling is vital as it prevents overheating, which can lead to equipment failure and downtime. Together, they form a symbiotic relationship where optimized power consumption can reduce cooling needs, and efficient cooling can enhance the overall power efficiency. In practice, DCPP involves meticulous planning and implementation to ensure that both power and cooling systems are aligned with the specific needs of the data center. This includes selecting appropriate hardware, designing efficient layouts, and implementing advanced monitoring and management tools. For instance, data centers often employ advanced cooling techniques such as hot aisle containment or cold aisle containment to maximize cooling efficiency. Additionally, power management strategies like power usage effectiveness (PUE) metrics help in measuring and optimizing the energy efficiency of the data center. Understanding DCPP is fundamental for data center administrators, engineers, and stakeholders because it directly impacts the performance, reliability, and cost-effectiveness of their operations. By grasping the nuances of power and cooling systems, professionals can make informed decisions that enhance overall data center efficiency, reduce operational costs, and ensure continuous uptime. This knowledge also enables them to adopt best practices and leverage emerging technologies such as green data centers and edge computing, which are increasingly important in today's digital landscape. In summary, DCPP is a cornerstone of modern data center management, emphasizing the critical balance between power supply and cooling mechanisms. As technology continues to evolve and demand for data storage and processing grows, mastering the basics of DCPP becomes indispensable for maintaining high-performance, sustainable data centers that support the ever-expanding digital ecosystem.
Historical Context and Development
Understanding the historical context and development of DCPP (Distributed Checksum Clearinghouse Protocol) is crucial for grasping its current role and significance in email spam filtering. DCPP, a protocol designed to combat spam, has its roots in the early 2000s when email spam became a pervasive issue. During this period, traditional spam filtering methods such as keyword-based filters and Bayesian algorithms were increasingly ineffective due to the sophisticated tactics employed by spammers. The concept of collaborative spam filtering emerged as a response to this challenge. One of the pioneering efforts in this area was the development of Vipul's Razor, a distributed spam-tracking system introduced in 2000. However, it was the creation of the Distributed Checksum Clearinghouse (DCC) by Vernon Schryver in 2004 that laid the groundwork for DCPP. The DCC used a distributed database to track checksums of email messages, allowing participating servers to quickly identify and reject spam emails based on collective data. DCPP built upon this foundation by enhancing the efficiency and security of the DCC system. It introduced improvements such as better handling of false positives, enhanced scalability, and more robust mechanisms for updating checksum databases. This evolution was driven by the need for more accurate and adaptive spam detection as spammers continued to innovate their tactics. The development of DCPP also reflects broader trends in cybersecurity and network collaboration. As the internet grew, so did the complexity of threats, necessitating collaborative solutions that leveraged collective intelligence. DCPP exemplifies this shift by enabling multiple email servers to share information about spam emails, thereby creating a robust defense against spam that is greater than the sum of its parts. Moreover, DCPP's development has been influenced by open-source principles and community-driven initiatives. The protocol's design allows for widespread adoption and customization, making it a versatile tool for various email systems. This openness has fostered a community of developers and users who continuously contribute to its improvement, ensuring that DCPP remains effective against evolving spam threats. In summary, the historical context and development of DCPP are marked by a series of innovations aimed at combating the increasingly sophisticated problem of email spam. From its roots in early collaborative filtering systems to its current form as a robust and adaptive protocol, DCPP represents a significant advancement in email security. Understanding this history provides essential context for appreciating the role of DCPP in modern email systems and its continued relevance in the fight against spam.
Primary Objectives and Goals
**Understanding the Basics of DCPP: Primary Objectives and Goals** At the heart of the Data-Centric Protection Protocol (DCPP) lies a robust framework designed to safeguard sensitive information in an increasingly interconnected world. The primary objectives and goals of DCPP are multifaceted, yet they converge on a singular mission: to ensure the integrity, confidentiality, and availability of data across various domains. First and foremost, **data integrity** is a cornerstone of DCPP. This objective involves ensuring that data remains accurate and unaltered throughout its lifecycle, from creation to storage and transmission. By implementing robust validation mechanisms and cryptographic techniques, DCPP prevents unauthorized modifications or tampering, thereby maintaining the trustworthiness of the data. Another critical goal is **confidentiality**, which focuses on protecting sensitive information from unauthorized access. DCPP achieves this through advanced encryption methods that render data unintelligible to anyone without the appropriate decryption keys. This ensures that even if data is intercepted or compromised, it remains secure and inaccessible to malicious actors. **Availability** is also a key objective, ensuring that authorized users can access the data when needed. DCPP addresses this by implementing resilient storage solutions and redundant systems that minimize downtime and data loss. This is particularly crucial in environments where continuous access to data is vital for operational efficiency and decision-making. In addition to these core objectives, DCPP aims to enhance **compliance** with regulatory standards and industry best practices. By aligning with stringent data protection regulations such as GDPR, HIPAA, and others, organizations can mitigate legal risks and maintain public trust. DCPP provides a structured approach to compliance, ensuring that all data handling processes adhere to the highest standards. Furthermore, DCPP emphasizes **scalability** and **flexibility** to accommodate diverse organizational needs. Whether it's a small enterprise or a large corporation, DCPP can be tailored to fit specific requirements without compromising on security or performance. This adaptability allows organizations to grow and evolve while maintaining robust data protection measures. Lastly, **user experience** plays a significant role in the design of DCPP. The protocol is engineered to be user-friendly, ensuring that security measures do not hinder productivity or workflow efficiency. By integrating seamlessly into existing systems and providing intuitive interfaces, DCPP fosters a culture of security awareness among users without imposing unnecessary burdens. In summary, the primary objectives and goals of DCPP are centered around ensuring data integrity, confidentiality, availability, compliance, scalability, flexibility, and a positive user experience. By achieving these goals, DCPP provides a comprehensive framework for safeguarding data in today's complex digital landscape, thereby protecting both the organization and its stakeholders from potential threats and breaches. This holistic approach makes DCPP an indispensable tool for any entity serious about data security in the modern era.
Key Components and Features of DCPP
When delving into the intricacies of Data Center Power Protection (DCPP), it is crucial to understand the key components and features that ensure reliable and efficient power distribution. This comprehensive overview will explore three pivotal aspects: Core Principles and Methodologies, Technical Specifications and Standards, and Tools and Software Utilized. At the heart of DCPP lies a robust framework of core principles and methodologies that guide the design, implementation, and maintenance of power protection systems. These principles are grounded in best practices that prioritize redundancy, scalability, and fault tolerance. Understanding these core principles is essential for ensuring that data centers operate with minimal downtime and optimal performance. Technical specifications and standards play a critical role in DCPP, as they define the benchmarks for equipment compatibility, safety, and performance. Adherence to these standards ensures that all components work seamlessly together, reducing the risk of system failures and enhancing overall reliability. Finally, the tools and software utilized in DCPP are vital for monitoring, managing, and optimizing power distribution. Advanced software solutions provide real-time analytics, predictive maintenance capabilities, and automated controls that help data center operators make informed decisions. By grasping these three critical elements—core principles and methodologies, technical specifications and standards, and tools and software utilized—data center professionals can build and maintain resilient power protection systems. Let us begin by examining the core principles and methodologies that form the foundation of effective DCPP.
Core Principles and Methodologies
The core principles and methodologies of the Data-Centric Parallel Processing (DCPP) framework are pivotal in ensuring its effectiveness and efficiency. At its core, DCPP is built around the principle of data locality, where data is processed as close to its source as possible to minimize latency and maximize throughput. This principle is supported by a distributed architecture that leverages a network of nodes, each capable of processing and storing data independently. One of the key methodologies employed by DCPP is the use of parallel processing algorithms that can be scaled horizontally across multiple nodes. This allows for the handling of large datasets with high performance, making it particularly suitable for big data analytics and machine learning applications. Another critical methodology is data partitioning, where large datasets are divided into smaller, manageable chunks that can be processed concurrently by different nodes. This not only enhances processing speed but also improves fault tolerance by ensuring that the failure of one node does not halt the entire process. DCPP also adheres to the principle of data immutability, which ensures that once data is written, it cannot be altered. This approach enhances data integrity and simplifies the management of concurrent updates. Additionally, DCPP incorporates robust data replication mechanisms to ensure high availability and redundancy, safeguarding against data loss due to node failures. The framework's design emphasizes flexibility and adaptability through the use of modular components. This modularity allows developers to easily integrate new functionalities and algorithms without disrupting the existing system. Furthermore, DCPP supports a variety of programming models and APIs, making it accessible to a wide range of developers with different skill sets and preferences. In terms of operational efficiency, DCPP employs advanced resource management techniques such as dynamic resource allocation and load balancing. These mechanisms ensure that resources are utilized optimally, preventing bottlenecks and ensuring consistent performance even under varying workloads. Security is another fundamental principle in DCPP, with robust encryption and access control mechanisms in place to protect sensitive data. The framework also supports auditing and logging features to provide transparency and accountability in data processing operations. Overall, the core principles and methodologies of DCPP are designed to provide a scalable, efficient, and reliable platform for data-intensive applications. By focusing on data locality, parallel processing, data partitioning, immutability, replication, modularity, operational efficiency, and security, DCPP offers a comprehensive solution for organizations seeking to leverage their data assets effectively. These principles and methodologies underpin the key components and features of DCPP, making it an indispensable tool in today's data-driven world.
Technical Specifications and Standards
Technical specifications and standards are crucial components in the development and implementation of Distributed Control and Protection Systems (DCPP), ensuring that these systems operate efficiently, reliably, and safely. These specifications outline the precise requirements for hardware, software, communication protocols, and performance metrics, thereby guaranteeing interoperability and consistency across different components and vendors. For instance, in DCPP systems, technical specifications might include details on the types of sensors and actuators to be used, the communication protocols such as IEC 61850 or Modbus, and the redundancy levels required for critical control functions. Standards such as those set by the International Electrotechnical Commission (IEC) or the Institute of Electrical and Electronics Engineers (IEEE) provide a framework that ensures compliance with industry best practices. These standards cover aspects like system architecture, data exchange formats, cybersecurity measures, and testing procedures. Adherence to these standards not only enhances system reliability but also facilitates maintenance, upgrades, and integration with other systems. Additionally, technical specifications often include environmental and operational requirements such as temperature ranges, humidity levels, and electromagnetic compatibility (EMC) standards to ensure that the system can function under various conditions. By adhering to these technical specifications and standards, DCPP systems can achieve high levels of performance, scalability, and fault tolerance, making them indispensable in modern industrial control environments. This meticulous attention to detail in technical specifications and standards is a key factor in the robustness and effectiveness of DCPP systems, enabling them to meet the demanding needs of contemporary industrial processes.
Tools and Software Utilized
In the realm of Distributed Computing Projects (DCPP), the tools and software utilized are pivotal in ensuring the efficiency, scalability, and reliability of these complex systems. At the heart of DCPP lies a robust suite of technologies that facilitate distributed computing, data management, and user interaction. **Distributed Computing Platforms** such as BOINC (Berkeley Open Infrastructure for Network Computing) and Condor are cornerstone tools, enabling the coordination of vast networks of volunteer computers to tackle computationally intensive tasks. These platforms provide the infrastructure necessary for project administrators to manage workloads, distribute tasks, and aggregate results from thousands of participating machines. **Data Management Systems** like relational databases (e.g., MySQL) and NoSQL databases (e.g., MongoDB) play a crucial role in storing and retrieving large datasets efficiently. These systems ensure data integrity, support high throughput, and offer flexible querying capabilities essential for analyzing the vast amounts of data generated by DCPPs. Additionally, **Data Processing Frameworks** such as Apache Hadoop and Spark are employed to handle big data processing tasks. These frameworks leverage distributed computing paradigms to process massive datasets in parallel, significantly reducing processing times and enhancing overall system performance. **Client-Server Software** is another key component, facilitating communication between the central server and client machines. Tools like GridMP and XtremWeb enable seamless interaction between these entities, ensuring that tasks are distributed efficiently and results are collected accurately. **Monitoring and Logging Tools** such as Nagios and ELK Stack (Elasticsearch, Logstash, Kibana) are vital for maintaining system health and diagnosing issues promptly. These tools provide real-time monitoring capabilities, alerting administrators to potential problems before they escalate into critical failures. **User Interface Tools** such as web portals and mobile apps enhance user engagement by providing intuitive interfaces for participants to monitor their contributions, view project statistics, and interact with the community. Platforms like WordPress or custom-built web applications are often used to create user-friendly websites that serve as the public face of DCPPs. Furthermore, **Security Software** such as SSL/TLS encryption and firewalls protect sensitive data from unauthorized access, ensuring the integrity of the project. **Scientific Software Libraries** like NumPy, SciPy, and OpenCL are integral for performing complex scientific computations efficiently. These libraries optimize computational tasks by leveraging multi-core processors and GPU acceleration, thereby speeding up simulations and data analysis. **Version Control Systems** such as Git help manage code repositories effectively, allowing developers to collaborate on software development while maintaining a clear history of changes. In summary, the tools and software utilized in DCPPs form a sophisticated ecosystem that supports every aspect of distributed computing projects—from task distribution and data management to user interaction and security. By leveraging these technologies, DCPPs can achieve unprecedented scales of computation while maintaining reliability and efficiency. This comprehensive toolkit is essential for the success of initiatives that rely on collective computational power to advance scientific knowledge and solve complex problems.
Applications and Impact of DCPP
The applications and impact of Distributed Control and Protection Systems (DCPP) are multifaceted and far-reaching, transforming various industries with their advanced capabilities. This technology has revolutionized the way control and protection are implemented, offering a high degree of reliability, efficiency, and scalability. In this article, we will delve into three key aspects that highlight the significance of DCPP: Industry-Specific Use Cases, Benefits and Advantages in Practice, and Future Trends and Potential Developments. Firstly, we will explore how DCPP is tailored to meet the unique needs of different industries such as power generation, oil and gas, and manufacturing. These industry-specific use cases demonstrate how DCPP enhances operational safety, reduces downtime, and improves overall performance. Secondly, we will discuss the tangible benefits and advantages that DCPP brings to real-world applications, including enhanced reliability, improved maintenance strategies, and cost savings. Finally, we will examine the future trends and potential developments in DCPP technology, including advancements in IoT integration, AI-driven analytics, and cybersecurity enhancements. By understanding these facets, readers will gain a comprehensive insight into the transformative power of DCPP. Let us begin by examining the diverse industry-specific use cases that illustrate the versatility and effectiveness of DCPP in various sectors.
Industry-Specific Use Cases
**Industry-Specific Use Cases** Distributed Cloud Processing Platforms (DCPP) are revolutionizing various industries by offering scalable, efficient, and secure solutions tailored to specific needs. In the **healthcare sector**, DCPP enables the rapid processing of large medical datasets, facilitating advanced analytics and AI-driven diagnostics. For instance, hospitals can leverage DCPP to analyze patient data in real-time, improving treatment outcomes and personalized care. This technology also enhances telemedicine by ensuring seamless video conferencing and data exchange between healthcare providers. In **finance**, DCPP plays a crucial role in risk management and compliance. Financial institutions can use DCPP to process vast amounts of transactional data quickly, detecting anomalies and potential fraud in real-time. This not only enhances security but also aids in regulatory compliance by providing detailed audit trails and reports. Additionally, DCPP supports high-frequency trading by executing trades at lightning-fast speeds, giving financial firms a competitive edge. The **manufacturing industry** benefits significantly from DCPP through enhanced IoT integration. By connecting and analyzing data from various sensors and machines on the factory floor, manufacturers can optimize production processes, predict maintenance needs, and reduce downtime. This predictive maintenance capability extends the lifespan of equipment and improves overall operational efficiency. In **retail**, DCPP is transforming customer experience through personalized marketing and inventory management. Retailers can analyze consumer behavior patterns in real-time, offering targeted promotions and recommendations that drive sales. Inventory management becomes more accurate with DCPP, ensuring that stock levels are optimized to meet demand without overstocking or understocking. The **energy sector** also sees substantial benefits from DCPP. Utility companies can use this technology to manage smart grids more effectively by analyzing real-time data from various sources such as weather stations, sensors, and consumer usage patterns. This helps in predicting energy demand and optimizing supply, reducing waste and improving grid reliability. Furthermore, in **transportation**, DCPP supports the development of smart cities by managing traffic flow efficiently. Real-time data from traffic sensors and cameras can be processed to optimize traffic light timings, reducing congestion and improving travel times. This technology also aids in autonomous vehicle development by providing the necessary computational power for real-time decision-making. In **education**, DCPP enhances learning experiences by enabling personalized learning paths based on individual student performance data. Educational institutions can analyze student interactions with digital resources to identify knowledge gaps and tailor educational content accordingly. This not only improves student outcomes but also makes educational resources more accessible. Across these industries, DCPP's ability to handle large volumes of data securely and efficiently makes it an indispensable tool for driving innovation and operational excellence. By leveraging DCPP, businesses can gain valuable insights, improve decision-making processes, and stay ahead of the competition in their respective markets.
Benefits and Advantages in Practice
The benefits and advantages of Distributed Constraint Programming (DCPP) in practice are multifaceted and significant, contributing substantially to various domains where complex decision-making and coordination are essential. One of the primary advantages of DCPP is its ability to handle large-scale problems efficiently by distributing the computational load across multiple agents. This scalability allows for the solving of problems that would be intractable or extremely time-consuming with centralized approaches, making it particularly valuable in fields such as logistics, supply chain management, and resource allocation. Another key benefit is the flexibility and autonomy it offers. In DCPP, each agent can operate independently while still contributing to the overall solution, which is crucial in environments where agents may have different goals or constraints. For instance, in smart grid management, DCPP can help optimize energy distribution by allowing individual households or businesses to manage their energy consumption autonomously while ensuring the overall grid stability. Moreover, DCPP enhances robustness and fault tolerance. Since the computation is distributed, the failure of one agent does not necessarily halt the entire process; other agents can continue working towards a solution. This resilience is particularly important in critical systems such as air traffic control or financial networks where downtime can have severe consequences. From a practical standpoint, DCPP also facilitates privacy and security. By keeping sensitive information localized within each agent rather than centralizing it, DCPP reduces the risk of data breaches and unauthorized access. This aspect is particularly beneficial in applications involving personal data, such as healthcare or financial transactions. Additionally, DCPP promotes real-time decision-making by enabling agents to react quickly to changing conditions without needing to wait for centralized updates. This real-time capability is invaluable in dynamic environments like traffic management systems or emergency response coordination. In terms of collaboration and coordination, DCPP fosters more effective communication among agents by allowing them to negotiate and reach agreements through iterative processes. This collaborative framework is especially useful in multi-stakeholder scenarios such as international diplomacy or business partnerships. Lastly, the adaptability of DCPP makes it an attractive solution for evolving systems. As new agents join or existing ones leave the network, DCPP can adapt seamlessly without requiring significant reconfiguration. This adaptability ensures that the system remains functional and efficient over time. In summary, the benefits and advantages of DCPP in practice include enhanced scalability, flexibility, robustness, privacy, real-time decision-making capabilities, effective collaboration mechanisms, and adaptability. These advantages make DCPP a powerful tool for addressing complex problems across various domains and applications.
Future Trends and Potential Developments
As we delve into the future trends and potential developments of Distributed Control and Protection Systems (DCPP), it becomes evident that these technologies are poised to revolutionize various industries. One of the most significant trends is the integration of advanced artificial intelligence (AI) and machine learning (ML) algorithms. These technologies will enable DCPP systems to predict and prevent faults more accurately, optimize performance in real-time, and enhance overall system reliability. For instance, AI-driven predictive maintenance can identify potential issues before they occur, reducing downtime and improving operational efficiency. Another key trend is the adoption of Internet of Things (IoT) devices, which will expand the scope of DCPP by connecting a vast array of sensors and actuators. This IoT integration will provide a granular level of control and monitoring, allowing for more precise adjustments and better decision-making. Additionally, the increasing use of cloud computing and edge computing will facilitate data processing closer to where it is generated, reducing latency and enhancing real-time response capabilities. Cybersecurity is also a critical area of focus for future DCPP developments. As these systems become more interconnected, the risk of cyber threats increases. Therefore, advanced security protocols such as encryption, secure communication protocols, and intrusion detection systems will be integral components of future DCPP architectures. This will ensure that sensitive data remains protected and that the integrity of the control and protection functions is maintained. Furthermore, the trend towards greater decentralization and autonomy in DCPP systems is expected to continue. This involves the use of blockchain technology to create secure, decentralized networks where data can be shared and verified without a central authority. Decentralized systems can offer higher resilience against failures and cyber attacks, making them particularly appealing for critical infrastructure applications. In terms of specific applications, DCPP is likely to have a profound impact on sectors such as energy, transportation, and manufacturing. For example, in the energy sector, DCPP can optimize grid operations by managing distributed renewable energy sources more effectively. In transportation, it can enhance the safety and efficiency of autonomous vehicles by providing real-time control and protection mechanisms. In manufacturing, DCPP can improve production processes by enabling more precise control over machinery and equipment. The impact of these trends will be multifaceted. Economically, they will drive cost savings through improved efficiency and reduced maintenance needs. Environmentally, they will contribute to sustainability by optimizing resource usage and reducing waste. Societally, they will enhance safety by minimizing the risk of accidents and ensuring reliable operation of critical infrastructure. In conclusion, the future of DCPP is marked by significant technological advancements that promise to transform various industries. By leveraging AI, IoT, cloud computing, cybersecurity measures, decentralization technologies, and other innovations, DCPP systems will become even more robust, efficient, and secure. These developments will not only drive economic benefits but also contribute to a safer and more sustainable future. As these trends unfold, it is clear that DCPP will play an increasingly vital role in shaping the technological landscape of the 21st century.