What Happens In Anaphase
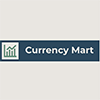
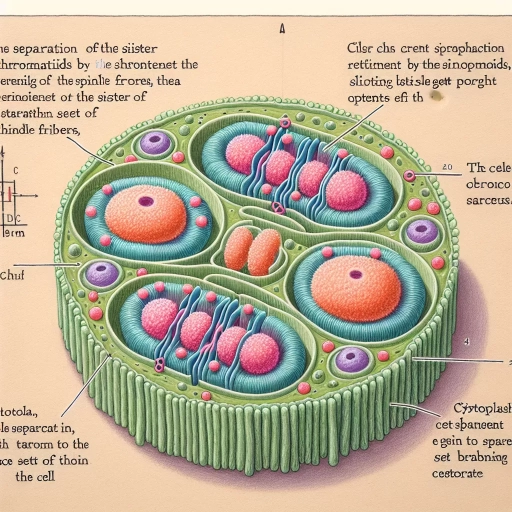
In the intricate dance of cell division, anaphase stands as a pivotal phase where the genetic material is meticulously separated to ensure the proper distribution of chromosomes to daughter cells. This critical stage is part of the broader process of mitosis, a fundamental mechanism in both plant and animal cells. Understanding anaphase is essential for grasping the overall dynamics of cell division, as it involves key events that are tightly regulated by complex mechanisms. This article delves into the intricacies of anaphase, beginning with an **Overview of Anaphase**, which sets the stage by explaining the context and significance of this phase. We will then explore the **Key Events During Anaphase**, detailing the precise steps involved in chromosome separation and movement. Finally, we will examine the **Regulation and Mechanisms of Anaphase**, uncovering the molecular controls and cellular machinery that ensure this process occurs with precision. By dissecting these aspects, we gain a comprehensive understanding of how anaphase contributes to the successful completion of cell division. Let us start by taking a closer look at the **Overview of Anaphase**.
Overview of Anaphase
In the intricate dance of cell division, one phase stands out for its critical role in ensuring the precise distribution of genetic material: Anaphase. This pivotal stage is a cornerstone of mitosis and meiosis, where chromosomes are separated and pulled to opposite poles of the cell. To fully understand Anaphase, it is essential to delve into its definition and context within the broader process of cell division. This involves exploring how Anaphase fits into the sequence of events that lead to the formation of new cells. Additionally, examining the role of Anaphase in cell division highlights its unique mechanisms and importance in maintaining genetic integrity. By comparing Anaphase with other phases of cell division, such as Prophase and Telophase, we can appreciate its distinct characteristics and functional significance. In this article, we will provide an overview of Anaphase, discussing its definition and context, its crucial role in cell division, and how it compares to other phases, offering a comprehensive understanding of this vital cellular process.
Definition and Context
In the intricate dance of cell division, **anaphase** stands as a pivotal phase where the replicated chromosomes are separated and pulled to opposite poles of the cell. To fully grasp the significance of anaphase, it is crucial to understand its definition and context within the broader process of mitosis or meiosis. **Definitionally**, anaphase is the third stage of mitosis and the second stage of meiosis II, following metaphase and preceding telophase. During this phase, the sister chromatids (in mitosis) or homologous chromosomes (in meiosis II) are separated due to the contraction of microtubules attached to the kinetochores, which are protein structures at the centromeres of chromosomes. This separation ensures that each daughter cell receives an identical set of chromosomes in mitosis or a unique combination in meiosis. **Contextually**, anaphase occurs after the chromosomes have aligned at the metaphase plate during metaphase. Here, the spindle fibers attached to the kinetochores contract, pulling the sister chromatids apart. This precise mechanism is essential for genetic continuity and diversity. In mitosis, it ensures that each daughter cell receives a complete and identical set of chromosomes, maintaining the genetic integrity of the organism. In meiosis II, it contributes to genetic diversity by ensuring that each gamete (sperm or egg cell) receives a unique combination of chromosomes, which is vital for sexual reproduction. The **mechanisms** underlying anaphase are highly regulated and involve complex interactions between microtubules, motor proteins, and kinetochores. The spindle checkpoint, a quality control mechanism, ensures that all chromosomes are properly attached to the spindle fibers before anaphase begins, preventing errors that could lead to chromosomal abnormalities. Once initiated, anaphase proceeds rapidly but precisely, with chromosomes moving towards opposite poles at speeds of up to several micrometers per minute. Understanding anaphase within its context highlights its critical role in cell division. It is a phase where the meticulous preparation of previous stages culminates in the physical separation of genetic material, setting the stage for the final stages of cell division—telophase and cytokinesis. This phase underscores the remarkable precision and coordination inherent in cellular processes, ensuring that genetic information is accurately distributed to daughter cells, thereby maintaining cellular and organismal health. By delving into the definition and context of anaphase, we gain a deeper appreciation for the intricate ballet of molecular interactions that govern cell division and the continuity of life.
Role in Cell Division
In the intricate process of cell division, particularly during the anaphase stage, several key roles come into play to ensure the precise and efficient separation of chromosomes. Anaphase is a critical phase where sister chromatids, now recognized as individual chromosomes, are pulled apart to opposite poles of the cell. This separation is facilitated by the spindle fibers, which are composed of microtubules that attach to the centromeres of the chromosomes. The role of these spindle fibers is multifaceted: they not only provide the mechanical force necessary to separate the chromosomes but also ensure that each pole receives an identical set of chromosomes, thus maintaining genetic integrity. Another crucial role in anaphase is played by the kinetochore, a protein structure located at the centromere of each chromosome. The kinetochore acts as an anchor point for the spindle fibers, allowing them to grasp and move the chromosomes. The interaction between kinetochores and spindle fibers is highly regulated, ensuring that all chromosomes are properly aligned and attached before the onset of anaphase. This alignment is checked by the spindle assembly checkpoint, which delays anaphase until all chromosomes are correctly attached to the spindle apparatus. Furthermore, motor proteins such as dynein and kinesin play vital roles in generating the forces required for chromosome movement. Dynein, located at the poles, pulls chromosomes towards the poles by sliding along the microtubules, while kinesin, associated with the kinetochores, helps in stabilizing and fine-tuning this movement. The coordinated action of these motor proteins ensures that chromosomes move in a synchronized manner, preventing any lag or missegregation. Additionally, the cytoskeleton undergoes significant reorganization during anaphase to support the structural changes within the cell. Actin filaments and intermediate filaments help maintain cell shape and provide additional mechanical support as the cell prepares for cytokinesis, the final stage of cell division where the cytoplasm divides and the cell splits into two daughter cells. In summary, the roles played by spindle fibers, kinetochores, motor proteins, and the cytoskeleton during anaphase are essential for accurate chromosome segregation. These components work in harmony to ensure that genetic material is distributed equally between daughter cells, a process that is fundamental to maintaining cellular and organismal health. Understanding these roles provides insights into the complex mechanisms underlying cell division and highlights the precision and coordination required for successful anaphase progression.
Comparison with Other Phases
In the context of cell division, particularly during mitosis, the phase of anaphase stands out for its unique and critical role. To fully appreciate the significance of anaphase, it is essential to compare it with other phases of the cell cycle. **Prophase**, the preceding phase, sets the stage for anaphase by preparing the chromosomes for separation. During prophase, chromosomes condense, the nuclear envelope breaks down, and the spindle fibers begin to form. In contrast, **anaphase** is characterized by the actual separation of sister chromatids to opposite poles of the cell. This phase ensures genetic material is evenly distributed between daughter cells, a process that is not initiated until the chromosomes are properly aligned at the metaphase plate. **Metaphase**, which immediately precedes anaphase, is a checkpoint phase where chromosomes align at the metaphase plate. This alignment is crucial because it ensures that each daughter cell will receive an identical set of chromosomes. Once this alignment is confirmed, the cell proceeds to anaphase, where the sister chromatids separate. This separation is a direct result of the shortening of spindle fibers and the movement of kinetochores towards opposite poles. **Telophase**, following anaphase, marks the beginning of the reformation of the nuclear envelope and the decondensation of chromosomes back into chromatin. While anaphase focuses on the physical separation of genetic material, telophase focuses on the reorganization and preparation for the next cell cycle or interphase. **Interphase**, which includes G1, S, and G2 phases, is the longest part of the cell cycle and occurs before mitosis begins. During interphase, the cell grows, replicates its DNA, and prepares for cell division. In contrast to these preparatory phases, anaphase is a highly dynamic and precise phase where the physical separation of chromosomes occurs. In summary, while each phase of the cell cycle has its distinct functions and importance, anaphase stands out as a critical juncture where genetic material is accurately distributed between daughter cells. The comparison with other phases highlights how anaphase builds upon the preparatory work done in prophase and metaphase and sets the stage for the reorganization seen in telophase. This precise coordination ensures that cell division proceeds accurately and efficiently, maintaining genetic integrity across generations.
Key Events During Anaphase
Anaphase, a critical phase of cell division, is characterized by a series of intricate and highly coordinated events that ensure the accurate separation of genetic material. During this phase, several key processes unfold, each playing a vital role in the successful completion of mitosis or meiosis. One of the most significant events is **Sister Chromatid Separation**, where the sister chromatids, previously joined at the centromere, are pulled apart to opposite poles of the cell. This separation is facilitated by **Chromatid Movement to Poles**, a process driven by the dynamic contraction of spindle fibers. The **Spindle Fiber Dynamics** are crucial in this context, as they provide the mechanical force necessary for chromatid movement and ensure that each daughter cell receives a complete and identical set of chromosomes. Understanding these events is essential for grasping the overall mechanics of anaphase. In this article, we will delve into each of these processes in detail, providing a comprehensive overview of anaphase and its critical components. Transitioning seamlessly into an **Overview of Anaphase**, we will explore how these elements work together to achieve precise chromosome segregation, a cornerstone of cellular reproduction.
Sister Chromatid Separation
During anaphase, one of the most critical events is the separation of sister chromatids, a process that ensures genetic material is accurately distributed to daughter cells. This phase begins once the spindle fibers attached to the kinetochores of sister chromatids are under tension, signaling that chromosomes are properly aligned at the metaphase plate. As anaphase commences, the cohesin proteins that hold sister chromatids together are cleaved by the enzyme separase, allowing these identical DNA molecules to separate. This separation is facilitated by the contraction of spindle fibers, which pull the sister chromatids towards opposite poles of the cell. The precise timing and execution of this event are crucial; any errors can lead to chromosomal abnormalities such as aneuploidy, where cells end up with an incorrect number of chromosomes. The dynamic interplay between microtubules and motor proteins further ensures that each pole receives a complete set of chromosomes, maintaining genomic integrity. Additionally, this process is tightly regulated by checkpoint mechanisms that verify chromosome alignment and attachment before allowing progression to the next stage of cell division. The successful separation of sister chromatids during anaphase is a cornerstone of mitosis and meiosis, ensuring that daughter cells inherit a complete and accurate set of genetic information necessary for their survival and function. This intricate ballet of molecular interactions underscores the complexity and precision inherent in cellular reproduction processes.
Chromatid Movement to Poles
During anaphase, one of the key events is the chromatid movement to the poles, a process that is both precise and crucial for the proper segregation of genetic material. This phase begins once the sister chromatids are separated at the centromere, marking the end of metaphase. The separated chromatids, now referred to as daughter chromosomes, are pulled towards opposite poles of the cell by the spindle fibers. These fibers, composed of microtubules, contract and shorten, generating the force necessary for chromosome movement. The kinetochore, a protein structure attached to the centromere of each chromosome, plays a pivotal role in this process by binding to the spindle fibers and facilitating their attachment and subsequent movement. The movement itself is highly coordinated and involves several mechanisms. The plus ends of the microtubules, which are embedded in the kinetochores, depolymerize as they move towards the poles, while the minus ends remain anchored at the centrosomes. This depolymerization process releases energy that propels the chromosomes poleward. Additionally, motor proteins such as dynein and kinesin contribute to this movement by sliding along the microtubules and generating additional force. The precision of chromatid movement is ensured by several checkpoints and regulatory mechanisms. For instance, the spindle assembly checkpoint (SAC) ensures that all chromosomes are properly attached to the spindle fibers before anaphase can proceed. If any chromosome is not correctly aligned or attached, the SAC delays the onset of anaphase until all chromosomes are in place. As the daughter chromosomes reach the poles, they begin to decondense and form distinct nuclei. This marks the beginning of telophase, where nuclear envelopes start to reform around each set of chromosomes. The accurate and timely movement of chromatids to the poles is essential for maintaining genomic integrity and ensuring that each daughter cell receives a complete and identical set of chromosomes. In summary, the movement of chromatids to the poles during anaphase is a complex yet highly regulated process that relies on the coordinated action of spindle fibers, kinetochores, and motor proteins. This precise mechanism ensures that genetic material is evenly distributed between daughter cells, a critical step in the cell division process.
Spindle Fiber Dynamics
During anaphase, one of the most critical and dynamic processes is the behavior of spindle fibers. These fibers, composed of microtubules, are essential for the precise separation of sister chromatids to opposite poles of the cell. At the onset of anaphase, the sister chromatid cohesion is released, allowing the spindle fibers to pull the chromosomes apart. This separation is facilitated by two types of spindle fibers: kinetochore microtubules and interpolar microtubules. Kinetochore microtubules attach directly to the kinetochores on the chromosomes, while interpolar microtubules overlap and interact with each other at the spindle equator. The dynamics of these spindle fibers are highly regulated and involve continuous assembly and disassembly. As kinetochore microtubules shorten, they pull the chromosomes towards the poles, a process known as anaphase A. Simultaneously, interpolar microtubules slide past each other, pushing the poles apart and elongating the spindle, a phenomenon referred to as anaphase B. This coordinated action ensures that each daughter cell receives a complete and identical set of chromosomes. The regulation of spindle fiber dynamics is complex and involves various proteins and molecular motors. Motor proteins such as dynein and kinesin play crucial roles in generating the forces necessary for microtubule movement. Dynein, for example, is involved in the transport of chromosomes along kinetochore microtubules towards the poles, while kinesin helps in the sliding of interpolar microtubules. Additionally, microtubule-associated proteins (MAPs) and kinases like Aurora B kinase fine-tune the stability and dynamics of microtubules, ensuring accurate chromosome segregation. The precision and speed of spindle fiber dynamics during anaphase are critical for maintaining genomic integrity. Any errors in this process can lead to chromosomal missegregation, resulting in aneuploidy or genetic instability, which are hallmarks of many cancers and developmental disorders. Therefore, understanding the intricate mechanisms governing spindle fiber dynamics is not only fascinating from a biological perspective but also crucial for developing therapeutic strategies to combat diseases associated with mitotic errors. In summary, the dynamic interplay of spindle fibers during anaphase is a highly orchestrated process that ensures the accurate distribution of genetic material, underscoring its importance in cellular reproduction and overall organismal health.
Regulation and Mechanisms of Anaphase
Anaphase, a critical phase of mitosis, is the process by which sister chromatids are separated and moved to opposite poles of the cell, ensuring accurate chromosome distribution to daughter cells. This intricate process is governed by a complex interplay of regulatory mechanisms and molecular interactions. To delve into the intricacies of anaphase, it is essential to understand the checkpoints and controls that ensure its precision, the molecular mechanisms involved in chromatid separation, and the pivotal role of motor proteins in orchestrating this movement. Checkpoints and controls serve as quality assurance measures, halting the process if errors are detected, while molecular mechanisms involve the dynamic interactions of various proteins and structures such as kinetochores and microtubules. Motor proteins, including dynein and kinesin, play a crucial role in generating the forces necessary for chromatid separation. By examining these components, we can gain a comprehensive understanding of the regulation and mechanisms of anaphase, setting the stage for an in-depth overview of this vital cellular process.
Checkpoints and Controls
During anaphase, the precise separation of sister chromatids is crucial for maintaining genomic integrity. To ensure this process occurs accurately, cells employ checkpoints and controls that monitor and regulate the progression of mitosis. These mechanisms are essential for preventing errors that could lead to chromosomal abnormalities, such as aneuploidy or chromosomal breakage. One key checkpoint is the spindle assembly checkpoint (SAC), which operates during metaphase but has a significant impact on anaphase. The SAC ensures that all chromosomes are properly aligned at the metaphase plate and attached to the spindle fibers before the onset of anaphase. This checkpoint involves proteins like Mad2, Bub1, and BubR1, which bind to unattached kinetochores, inhibiting the anaphase-promoting complex (APC) until all chromosomes are correctly aligned. Once all chromosomes are properly attached and aligned, the SAC is satisfied, allowing the APC to activate the separation of sister chromatids. Another critical control mechanism involves the kinetochore, a protein structure attached to the centromere of each chromosome. Kinetochores play a dual role in anaphase: they are the sites where spindle fibers attach to chromosomes and also serve as sensors for the SAC. Proper kinetochore function ensures that each chromosome is attached to microtubules emanating from opposite poles of the cell, facilitating their precise separation during anaphase. Additionally, the Aurora B kinase pathway acts as a quality control mechanism during anaphase. This pathway corrects improper kinetochore attachments by phosphorylating and destabilizing incorrect attachments, allowing for their reattachment in a correct manner. This ensures that sister chromatids separate to opposite poles without any lagging chromosomes. In summary, checkpoints and controls during anaphase are vital for ensuring the accurate separation of sister chromatids. The SAC and kinetochore mechanisms work in tandem to guarantee that chromosomes are correctly aligned and attached before anaphase begins, while the Aurora B kinase pathway corrects any errors in kinetochore attachments. These regulatory mechanisms collectively contribute to the fidelity of chromosome segregation, safeguarding against genetic errors that could arise from missegregation events. By understanding these intricate controls, we gain insight into the complex processes that underpin cell division and the maintenance of genomic stability.
Molecular Mechanisms Involved
During anaphase, the molecular mechanisms that govern chromosome segregation are intricately coordinated to ensure accurate and efficient separation of sister chromatids. At the heart of these mechanisms is the spindle apparatus, a dynamic structure composed of microtubules that emanate from the centrosomes at each pole of the cell. The kinetochore, a protein complex attached to the centromere of each chromosome, plays a crucial role by binding to microtubules and facilitating their attachment to the chromosomes. This interaction is mediated by kinetochore-associated proteins such as Ndc80 and Mis12 complexes, which stabilize microtubule attachments and allow for precise alignment of chromosomes at the metaphase plate. The onset of anaphase is triggered by the activation of the anaphase-promoting complex (APC), a ubiquitin ligase that targets key regulatory proteins for degradation. Specifically, APC-mediated degradation of securin leads to the activation of separase, an enzyme that cleaves cohesin complexes holding sister chromatids together. Cohesin is a ring-like structure composed of SMC1, SMC3, and kleisin subunits, which encircles the sister chromatids and maintains their cohesion until anaphase. The dissolution of cohesin allows sister chromatids to separate and move towards opposite poles of the cell. Microtubule dynamics also play a critical role during anaphase. The plus ends of microtubules attached to kinetochores undergo rapid depolymerization, generating force that pulls the chromosomes towards the poles. This process is regulated by proteins such as MCAK (mitotic centromere-associated kinesin) and Kif2A, which modulate microtubule stability and dynamics. Additionally, motor proteins like dynein and kinesin-5 contribute to spindle elongation and chromosome movement by generating forces along microtubules. The Aurora B kinase pathway is another essential component in ensuring accurate chromosome segregation during anaphase. Aurora B monitors kinetochore-microtubule attachments and corrects any improper attachments through a process known as the spindle assembly checkpoint (SAC). If chromosomes are not properly aligned or attached, the SAC delays anaphase onset until all chromosomes are correctly positioned. This surveillance mechanism prevents errors in chromosome segregation that could lead to aneuploidy or genetic instability. In summary, the molecular mechanisms involved in anaphase are highly regulated and involve complex interactions between kinetochores, microtubules, cohesin complexes, and various regulatory proteins. These mechanisms work in concert to ensure precise and efficient separation of sister chromatids, thereby maintaining genomic integrity during cell division.
Role of Motor Proteins
During anaphase, the precise and coordinated movement of chromosomes to opposite poles of the cell is crucial for ensuring genetic integrity. This intricate process is largely facilitated by motor proteins, which play a pivotal role in the regulation and mechanisms of anaphase. Motor proteins, such as dynein and kinesin, are molecular machines that convert chemical energy from ATP hydrolysis into mechanical work. In the context of anaphase, these proteins interact with microtubules of the mitotic spindle to drive chromosome segregation. Dynein, primarily associated with the minus end of microtubules, pulls chromosomes towards the spindle poles by walking along the microtubule tracks. Conversely, kinesin motors, often linked to the plus end of microtubules, push chromosomes away from the poles or stabilize microtubules to maintain spindle integrity. The balanced action of these motor proteins ensures that sister chromatids are separated and moved to opposite poles efficiently. Additionally, motor proteins like CENP-E (centromere protein E), a kinesin-related protein, specifically bind to kinetochores—the protein structures attached to centromeres—and facilitate the initial alignment of chromosomes at the metaphase plate before anaphase onset. The regulation of motor protein activity is tightly controlled to prevent errors in chromosome segregation. For instance, the Aurora B kinase complex monitors kinetochore-microtubule attachments and corrects any improper attachments by phosphorylating and thus inhibiting motor proteins until correct bipolar attachments are established. This checkpoint mechanism ensures that chromosomes are properly aligned before anaphase proceeds. Moreover, the dynamic instability of microtubules themselves is modulated by motor proteins to maintain spindle tension and stability. The interplay between motor proteins and microtubules not only drives chromosome movement but also contributes to the structural integrity of the mitotic spindle. In summary, motor proteins are indispensable for the precise regulation and execution of anaphase, ensuring that chromosomes are accurately segregated and genetic material is preserved for the next generation of cells. Their coordinated actions underscore the complexity and beauty of cellular machinery during mitosis.