What Are Specialized Cells
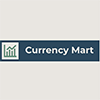
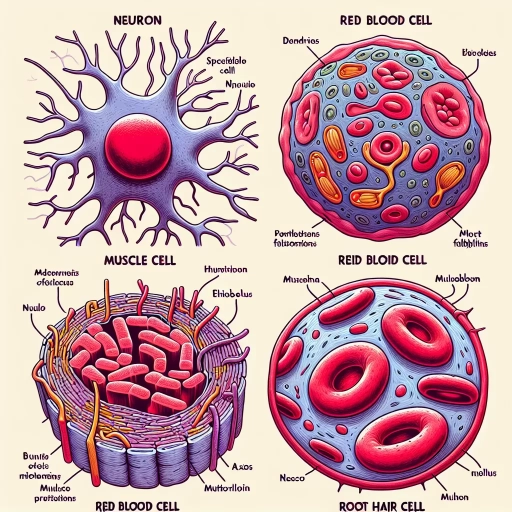
In the intricate tapestry of life, cells are the fundamental building blocks that underpin all biological processes. Among these cells, specialized cells stand out for their unique roles and adaptations that enable them to perform specific functions with remarkable efficiency. These cells are not just mere components; they are highly specialized entities that have evolved to meet the diverse needs of an organism. This article delves into the fascinating world of specialized cells, exploring their definition and characteristics, the various types found in the human body, and their critical importance and functions. By understanding what makes these cells so unique, we can appreciate the intricate mechanisms that sustain life. Let us begin by examining the definition and characteristics of specialized cells, which form the foundation of their extraordinary capabilities.
Definition and Characteristics of Specialized Cells
Specialized cells are the cornerstone of complex life forms, enabling organisms to perform a wide array of functions with precision and efficiency. These cells are distinguished by their unique structural features, specific functional roles, and the intricate cellular differentiation process that shapes them. Understanding these aspects is crucial for appreciating the sophistication of biological systems. Unique structural features, such as the presence of flagella in sperm cells or the extensive network of dendrites in neurons, allow specialized cells to execute their roles effectively. Specific functional roles, like the oxygen transport by red blood cells or the electrical signaling by neurons, are vital for maintaining homeostasis and facilitating communication within the body. The cellular differentiation process, which involves a series of genetic and environmental cues, ensures that these cells develop from stem cells into highly specialized entities. By examining these three key aspects—unique structural features, specific functional roles, and the cellular differentiation process—we can gain a deeper insight into how specialized cells contribute to the overall health and function of an organism. Let's begin by exploring the unique structural features that set these cells apart.
Unique Structural Features
Specialized cells, the cornerstone of complex life forms, exhibit unique structural features that enable them to perform specific functions with remarkable efficiency. These cells are tailored to their roles through distinct morphological and biochemical adaptations. For instance, nerve cells or neurons possess long, thin extensions called axons and dendrites, which facilitate the transmission and reception of electrical signals across vast distances, allowing for rapid communication within the nervous system. In contrast, muscle cells (skeletal, smooth, and cardiac) are characterized by their ability to contract, thanks to the presence of actin and myosin filaments that slide past each other to generate force. This structural specialization is crucial for movement, maintaining posture, and pumping blood throughout the body. Red blood cells, another example of highly specialized cells, lack a nucleus and most organelles to maximize their capacity for carrying oxygen. Their biconcave disk shape increases their surface area relative to volume, enhancing gas exchange efficiency. White blood cells, such as neutrophils and lymphocytes, have distinct shapes and surface receptors that aid in recognizing and combating pathogens. Neutrophils contain granules filled with enzymes and antimicrobial peptides, while lymphocytes have specific receptors on their surfaces that allow them to recognize and target specific antigens. Epithelial cells form tight junctions and desmosomes to create impermeable barriers in tissues like the skin and gut lining, protecting the body from external pathogens and toxins while regulating the passage of substances. In the gut, microvilli on the surface of epithelial cells increase the absorptive area for nutrients. Similarly, in the kidneys, podocytes have foot processes that form filtration slits crucial for filtering waste from the blood. Plant cells also display unique structural features tailored to their functions. For example, xylem cells are dead at maturity and form hollow tubes that transport water and minerals from the roots to the leaves through a process known as transpiration. Phloem cells, on the other hand, are alive and transport sugars and other organic compounds produced by photosynthesis. The cell walls of plant cells are rigid due to cellulose, providing structural support and protection against mechanical stress. In addition to these morphological adaptations, specialized cells often have specific organelle compositions that support their functions. For instance, liver cells (hepatocytes) are rich in mitochondria and smooth endoplasmic reticulum, enabling them to metabolize drugs and detoxify harmful substances efficiently. Pancreatic beta cells contain numerous secretory vesicles filled with insulin, which they release in response to elevated blood glucose levels. These unique structural features underscore the remarkable diversity and adaptability of specialized cells, each designed to optimize their performance in their respective roles within an organism. This specialization is a key factor in the complexity and functionality of multicellular organisms, allowing them to thrive in a wide range of environments. By understanding these structural adaptations, we gain insights into how life has evolved to meet specific biological needs, highlighting the intricate beauty of cellular biology.
Specific Functional Roles
Specialized cells, the cornerstone of complex life forms, exhibit a wide array of specific functional roles that are crucial for the survival and efficiency of organisms. These cells, often referred to as differentiated cells, have evolved to perform unique tasks that contribute to the overall health and function of an organism. For instance, **neurons** in the nervous system are specialized for transmitting and processing information through electrical and chemical signals, enabling communication between different parts of the body. **Muscle cells**, including skeletal, smooth, and cardiac muscles, are designed for contraction and movement. Skeletal muscles facilitate voluntary movements such as walking and running, while smooth muscles control involuntary actions like digestion and blood pressure regulation. Cardiac muscles, found in the heart, ensure continuous pumping of blood throughout the body. **Epithelial cells** form the lining of various body surfaces and cavities, serving as barriers against external pathogens and aiding in absorption and secretion processes. For example, epithelial cells in the small intestine are specialized for nutrient absorption, while those in the skin protect against environmental stressors. **Connective tissue cells**, including osteocytes in bone, chondrocytes in cartilage, and adipocytes in fat tissue, provide structural support and facilitate the storage of energy. **Immune cells**, such as T lymphocytes and B lymphocytes, are pivotal in defending against infections by recognizing and eliminating foreign substances. **Endocrine cells**, found in glands like the pancreas and thyroid, produce hormones that regulate various bodily functions including metabolism, growth, and reproductive processes. **Red blood cells**, or erythrocytes, are optimized for oxygen transport from the lungs to tissues throughout the body. **Platelets**, or thrombocytes, play a critical role in blood clotting, preventing excessive bleeding from injuries. Each type of specialized cell has distinct morphological and biochemical characteristics tailored to its specific function, underscoring the intricate specialization that underpins the complexity of life. The specialization of these cells is not just about their structure but also about their gene expression profiles. Differentiated cells express specific sets of genes that encode proteins necessary for their unique functions. This precise regulation ensures that each cell type performs its role efficiently without unnecessary energy expenditure on non-essential functions. The interplay between these specialized cells forms the basis of tissue and organ function, highlighting the remarkable diversity and coordination within living organisms. In summary, the specific functional roles of specialized cells are fundamental to the proper functioning of an organism. Each cell type has evolved unique characteristics that enable it to contribute to the organism's overall health and survival. Understanding these roles provides insights into the intricate mechanisms that govern life and underscores the importance of cellular specialization in maintaining the delicate balance of physiological processes.
Cellular Differentiation Process
Cellular differentiation is the intricate process by which a cell changes from one cell type to another, often resulting in specialized cells that perform specific functions. This complex transformation is crucial for the development and maintenance of multicellular organisms, allowing tissues and organs to form and function optimally. The journey begins with stem cells, which are undifferentiated cells capable of dividing to produce more stem cells or differentiating into specialized cells. The differentiation process involves a series of tightly regulated genetic and epigenetic changes that guide the cell towards its final phenotype. At the molecular level, cellular differentiation is orchestrated by a cascade of signaling pathways and transcriptional regulation. Key transcription factors bind to specific DNA sequences, activating or repressing gene expression to shape the cell's fate. For instance, during the differentiation of a stem cell into a muscle cell (myocyte), specific transcription factors such as MyoD and Myf5 are activated, leading to the expression of muscle-specific genes. Simultaneously, genes not required for muscle function are silenced, ensuring the cell adopts the correct morphology and function. The environment also plays a pivotal role in cellular differentiation. External signals from neighboring cells, growth factors, and the extracellular matrix provide critical cues that influence the differentiation pathway. For example, during embryonic development, gradients of signaling molecules like morphogens guide cells to differentiate into various tissue types. Additionally, mechanical forces and physical interactions with the extracellular matrix can trigger specific signaling pathways that direct cell fate decisions. Once differentiated, specialized cells exhibit distinct characteristics that enable them to perform their unique roles. For instance, neurons have extended axons and dendrites for transmitting and receiving signals, while red blood cells lack a nucleus to maximize their oxygen-carrying capacity. These specialized features are maintained through ongoing gene expression and cellular homeostasis mechanisms. In adult organisms, cellular differentiation continues to be essential for tissue repair and regeneration. Adult stem cells, such as those found in bone marrow or skin, can differentiate into various cell types to replace damaged or aged cells. This capacity for differentiation is vital for maintaining tissue integrity and function over an organism's lifetime. Understanding cellular differentiation has significant implications for medical research and therapy. Insights into the mechanisms of differentiation can inform strategies for regenerative medicine, where the goal is to use stem cells to repair or replace damaged tissues. Moreover, dysregulation of cellular differentiation processes is associated with various diseases, including cancer, where cells fail to differentiate properly and instead proliferate uncontrollably. In summary, cellular differentiation is a highly regulated and dynamic process that transforms undifferentiated cells into specialized cells with unique functions. This process is fundamental to the development and maintenance of complex life forms and continues to be an area of active research with profound implications for human health and disease.
Types of Specialized Cells in the Human Body
The human body is a complex and highly specialized organism, comprising a vast array of cell types that each play unique roles in maintaining overall health and function. Among these, three types of specialized cells stand out for their critical contributions: Nerve Cells (Neurons), Muscle Cells (Myocytes), and Epithelial Cells. Nerve Cells, or neurons, are the building blocks of the nervous system, enabling communication and coordination throughout the body. Muscle Cells, including skeletal, smooth, and cardiac myocytes, are responsible for movement, contraction, and maintaining the structural integrity of various tissues. Epithelial Cells form the lining of organs, glands, and other bodily surfaces, facilitating functions such as absorption, secretion, and protection. Understanding these specialized cells is essential for grasping how the human body operates at its most fundamental level. By delving into the specific roles and characteristics of each, we can appreciate the intricate mechanisms that underpin our very existence. Let's begin by exploring the fascinating world of Nerve Cells (Neurons), the primary components of our nervous system.
Nerve Cells (Neurons)
Nerve cells, or neurons, are among the most fascinating and critical specialized cells in the human body. These intricate cells form the backbone of the nervous system, enabling communication and coordination between different parts of the body. Structurally, neurons consist of three main components: the dendrites, cell body (soma), and axon. Dendrites are branching extensions that receive signals from other neurons, while the cell body contains the nucleus and is responsible for protein synthesis and metabolic activities. The axon, often long and thin, carries electrical signals away from the cell body to other neurons, muscles, or glands. Functionally, neurons are specialized to transmit and process information through electrical and chemical signals. When a neuron is stimulated, it generates an action potential—a rapid change in electrical charge that travels down the length of the axon. This electrical signal is then converted into a chemical signal at the synapse, the gap between two neurons, where neurotransmitters are released to bind with receptors on adjacent neurons, facilitating the continuation of the signal. There are several types of neurons, each with distinct roles. Sensory neurons transmit information from sensory receptors to the central nervous system, allowing us to perceive the world around us. Motor neurons carry signals from the central nervous system to muscles and glands, enabling movement and other bodily functions. Interneurons, found within the central nervous system, process and integrate information between sensory and motor neurons. The complexity and diversity of neurons are underscored by their unique properties and behaviors. For instance, some neurons can fire repeatedly without resting, while others may have a refractory period during which they cannot generate another action potential. Additionally, neurons exhibit synaptic plasticity—the ability to strengthen or weaken connections with other neurons based on activity patterns—a key mechanism underlying learning and memory. In terms of development, neurons are formed during embryogenesis and early childhood through a process called neurogenesis. Once formed, most neurons do not divide again; instead, they maintain their structure and function throughout life. However, recent research has shown that certain parts of the brain continue to produce new neurons even in adulthood, a phenomenon known as adult neurogenesis. Dysfunction or damage to neurons can lead to various neurological disorders. For example, Alzheimer's disease is characterized by the degeneration of neurons in the brain, leading to cognitive decline. Parkinson's disease involves the loss of dopamine-producing neurons in the substantia nigra, resulting in motor symptoms such as tremors and rigidity. Understanding how neurons work and how they can be protected or repaired is crucial for developing treatments for these conditions. In conclusion, nerve cells are highly specialized and essential for the proper functioning of the human body. Their unique structure and function enable complex processes like perception, movement, and cognition. The study of neurons continues to be a vibrant area of research, offering insights into both normal physiological processes and pathological conditions, ultimately contributing to our understanding of human health and disease.
Muscle Cells (Myocytes)
Muscle cells, or myocytes, are a crucial type of specialized cell in the human body, playing a pivotal role in movement, posture, and overall physical function. These cells are highly differentiated to perform their specific roles, which include contraction to generate force and movement. There are three primary types of muscle cells: skeletal, smooth, and cardiac myocytes. **Skeletal Myocytes** are the most abundant type and are found in skeletal muscles. These cells are multinucleated, meaning they contain multiple nuclei, and are characterized by their striated appearance under a microscope due to the regular arrangement of actin and myosin filaments. Skeletal myocytes are responsible for voluntary movements such as walking, running, and lifting. They are attached to bones via tendons and work in conjunction with the nervous system to execute precise movements. **Smooth Myocytes** are non-striated and lack the multinucleation seen in skeletal myocytes. They are found in the walls of hollow organs like the digestive tract, blood vessels, and airways. Smooth muscle cells are involved in involuntary movements such as peristalsis (the movement of food through the digestive tract) and vasoconstriction (the narrowing of blood vessels). Unlike skeletal muscles, smooth muscles operate autonomously without direct nervous control. **Cardiac Myocytes**, or cardiomyocytes, are specialized for the heart muscle. These cells are also striated but have a unique structure adapted for the heart's continuous pumping action. Cardiac myocytes are interconnected by gap junctions that allow electrical signals to pass through, enabling synchronized contractions necessary for efficient heart function. The heart's ability to pump blood throughout the body is entirely dependent on the coordinated contraction of these cells. The structure and function of muscle cells are tailored to their specific roles. For instance, all muscle cells contain sarcomeres—the functional units of muscle contraction—but the arrangement and size of these sarcomeres vary between skeletal, smooth, and cardiac muscles. Additionally, muscle cells have a high demand for energy, which is met by an extensive network of mitochondria that produce ATP through cellular respiration. In terms of development, muscle cells undergo a process called myogenesis during embryonic development. This involves the differentiation of precursor cells into mature myocytes through a series of complex cellular and molecular interactions. Once formed, muscle cells can undergo hypertrophy (increase in size) in response to increased demand or atrophy (decrease in size) due to disuse or disease. Dysfunction or damage to muscle cells can lead to various diseases and conditions. For example, muscular dystrophy results from genetic mutations affecting proteins essential for muscle integrity, leading to progressive muscle weakness and degeneration. Similarly, heart failure can occur when cardiac myocytes are damaged due to conditions like myocardial infarction or cardiomyopathy. In conclusion, muscle cells are vital components of the human body's musculoskeletal and cardiovascular systems. Their specialized structures and functions enable a wide range of movements and physiological processes essential for life. Understanding these cells is crucial not only for appreciating human physiology but also for diagnosing and treating muscle-related disorders.
Epithelial Cells
Epithelial cells are a fundamental component of the human body, forming the lining of various organs, glands, and other bodily surfaces. These specialized cells play a crucial role in maintaining the integrity and function of these surfaces, acting as barriers against external factors such as pathogens, toxins, and physical damage. There are several types of epithelial cells, each with distinct functions tailored to their specific locations. For instance, squamous epithelial cells are flat and plate-like, often found in areas where filtration and diffusion are essential, such as the walls of blood vessels and the alveoli of the lungs. Cuboidal epithelial cells are cube-shaped and typically line glands and ducts, facilitating secretion and absorption processes. Columnar epithelial cells are tall and column-like, commonly found in the lining of the digestive tract where they aid in absorption and secretion of digestive enzymes. In addition to their structural roles, epithelial cells are involved in various physiological processes. They form tight junctions that prevent the passage of substances through the spaces between them, ensuring selective permeability. This is particularly important in the gut epithelium, where it helps to regulate nutrient absorption while preventing harmful substances from entering the bloodstream. Epithelial cells also participate in sensory functions; for example, taste buds on the tongue are composed of specialized epithelial cells that detect different flavors. Moreover, epithelial cells have a remarkable ability to regenerate and repair damaged tissues. This capacity is vital for maintaining tissue homeostasis and is particularly evident in the skin and gut lining, where continuous cell turnover occurs to replace worn-out or damaged cells. The regenerative potential of epithelial cells is supported by stem cells located within epithelial tissues, which can differentiate into various types of epithelial cells as needed. The importance of epithelial cells extends beyond their individual functions; they also interact with other cell types to form complex tissues and organs. For example, in the skin, epithelial cells work in conjunction with dermal cells to provide protection against environmental stressors. Similarly, in the respiratory tract, epithelial cells collaborate with immune cells to defend against airborne pathogens. In summary, epithelial cells are highly specialized and versatile components of the human body. Their diverse forms and functions enable them to perform a wide range of critical roles, from barrier formation and secretion to sensory perception and tissue regeneration. Understanding the biology of epithelial cells is essential for appreciating the intricate mechanisms that maintain health and prevent disease. As one of the primary types of specialized cells in the body, epithelial cells underscore the complexity and sophistication of human physiology.
Importance and Functions of Specialized Cells
The importance and functions of specialized cells are pivotal in the intricate machinery of the human body. Specialized cells, each with unique structures and roles, contribute significantly to various biological processes. This article delves into three key aspects that highlight their importance: Tissue and Organ Formation, Specialized Cellular Activities, and Overall Health and Disease Prevention. Specialized cells are the building blocks of tissues and organs, which are essential for maintaining the body's homeostasis. These cells work in harmony to form complex structures that perform specific functions, such as muscle cells enabling movement and nerve cells facilitating communication. The precise organization and specialization of these cells are crucial for the development and maintenance of tissues and organs. Moreover, specialized cells engage in a wide range of activities that are vital for the body's overall functioning. For instance, red blood cells transport oxygen, while white blood cells defend against pathogens. The efficiency and coordination of these cellular activities ensure that the body operates optimally. Finally, the health and prevention of diseases are directly linked to the proper functioning of specialized cells. When these cells malfunction or are compromised, it can lead to various health issues. Understanding the roles of specialized cells is therefore essential for developing treatments and preventive measures. Transitioning to the first supporting idea, let us explore how specialized cells contribute to **Tissue and Organ Formation**, a fundamental process that underpins the very existence of complex life forms.
Tissue and Organ Formation
Tissue and organ formation are intricate processes that underscore the importance and functions of specialized cells. Specialized cells, each with unique structures and functions, come together to form tissues, which in turn aggregate to create organs. This hierarchical organization is crucial for the proper functioning of an organism. At the cellular level, specialized cells such as epithelial, connective, muscle, and nervous cells contribute to the formation of four primary types of tissues: epithelial tissue, which forms the lining of organs and glands; connective tissue, which provides support and binds other tissues together; muscle tissue, responsible for movement; and nervous tissue, which facilitates communication through electrical and chemical signals. The process of tissue formation begins with cell differentiation, where stem cells or progenitor cells acquire specific characteristics and functions. For instance, during embryonic development, mesenchymal stem cells differentiate into various types of connective tissue cells like osteoblasts (bone cells), chondrocytes (cartilage cells), and adipocytes (fat cells). Similarly, neural stem cells differentiate into neurons and glial cells to form nervous tissue. These differentiated cells then organize into specific patterns and structures through a series of complex cellular interactions involving adhesion molecules, growth factors, and extracellular matrix components. As tissues form, they begin to function in a coordinated manner. For example, epithelial tissues form tight junctions to create barriers that regulate the passage of substances, while muscle tissues contract and relax to facilitate movement. The integration of these functional tissues leads to the development of organs such as the skin, liver, heart, and brain. Each organ is composed of multiple types of tissues working together to perform specific functions essential for maintaining homeostasis and overall health. The importance of specialized cells in tissue and organ formation cannot be overstated. Without the precise differentiation and organization of these cells, tissues would lack the structural integrity and functional capabilities necessary for organ development. For instance, in the absence of properly differentiated cardiac muscle cells, the heart would be unable to pump blood efficiently, leading to severe cardiovascular issues. Similarly, the lack of functional neurons would impair cognitive functions and motor control. Moreover, the specialized nature of cells allows for remarkable adaptability and resilience. In response to injury or disease, certain specialized cells have the capacity to proliferate and differentiate into replacement cells, a process known as tissue repair. This ability is particularly evident in tissues like the skin and liver, which can regenerate lost or damaged cells to restore normal function. In conclusion, the formation of tissues and organs is a testament to the critical role of specialized cells. These cells, through their unique structures and functions, enable the creation of complex biological systems that underpin life itself. Understanding the importance and functions of specialized cells in tissue and organ formation provides valuable insights into human health and disease, highlighting the potential for regenerative medicine and tissue engineering to address various medical conditions. This knowledge also underscores the intricate beauty and complexity of biological systems, where each cell plays a vital role in maintaining the integrity and function of the organism as a whole.
Specialized Cellular Activities
Specialized cellular activities are the cornerstone of complex biological processes, enabling organisms to perform a wide range of functions with precision and efficiency. These activities are carried out by specialized cells, which are highly differentiated to fulfill specific roles within an organism. For instance, in the human body, red blood cells are specialized for oxygen transport, with their biconcave shape and lack of a nucleus allowing them to maximize oxygen-carrying capacity. Similarly, nerve cells or neurons are specialized for rapid transmission of electrical signals, featuring long axons and dendrites that facilitate communication between different parts of the body. Muscle cells, another example, are designed for contraction and movement. Skeletal muscle cells contain numerous myofibrils that allow for powerful contractions, while smooth muscle cells in the digestive tract facilitate peristalsis to move food through the digestive system. Epithelial cells form the lining of various organs and glands, acting as barriers against external pathogens and aiding in absorption and secretion processes. The pancreas, for example, contains specialized epithelial cells known as beta cells that produce insulin to regulate blood sugar levels. The specialization of cells also extends to immune cells such as T lymphocytes and B lymphocytes, which play crucial roles in adaptive immunity. T cells recognize and destroy infected cells or produce chemical signals that activate other immune responses, while B cells produce antibodies to neutralize pathogens. In plants, specialized cells like guard cells control stomatal openings to regulate gas exchange and water loss, while xylem and phloem cells are responsible for the transport of water, nutrients, and sugars throughout the plant. These specialized cellular activities are not only essential for maintaining homeostasis but also for enabling organisms to adapt to changing environments. For example, in response to injury, specialized cells such as fibroblasts and macrophages are activated to initiate wound healing processes. Fibroblasts produce collagen to form new tissue, while macrophages clear debris and pathogens from the wound site. The importance of these specialized cellular activities cannot be overstated. They underpin the complex functions that allow organisms to survive, grow, and reproduce. Without these highly specialized cells performing their unique roles, life as we know it would be impossible. The intricate coordination and cooperation among different types of specialized cells ensure that all bodily functions operate harmoniously, highlighting the remarkable efficiency and adaptability of biological systems. Understanding these specialized cellular activities provides valuable insights into both normal physiological processes and the mechanisms underlying various diseases, thereby informing medical treatments and research endeavors aimed at improving human health.
Overall Health and Disease Prevention
Overall health and disease prevention are intricately linked to the functions and importance of specialized cells within the human body. Specialized cells, each with unique structures and roles, form the cornerstone of our physiological processes. For instance, immune cells such as T-cells and B-cells are crucial for recognizing and combating pathogens, thereby preventing infections and diseases. These cells undergo complex processes like antigen presentation and antibody production to ensure that the body remains protected against harmful invaders. Similarly, epithelial cells line the surfaces of organs and glands, acting as barriers to external pathogens while also facilitating the absorption of nutrients in the digestive system. The integrity of these epithelial layers is vital for maintaining overall health by preventing the entry of harmful substances into the body. In addition to immune and epithelial cells, other specialized cells play critical roles in disease prevention. For example, endothelial cells lining blood vessels regulate blood pressure and prevent atherosclerosis by maintaining vascular health. Smooth muscle cells in the walls of blood vessels and the digestive tract enable contraction and relaxation, which is essential for blood circulation and peristalsis. The dysfunction of these cells can lead to conditions such as hypertension and gastrointestinal disorders. Moreover, the health of specialized cells is directly tied to lifestyle choices and environmental factors. A diet rich in essential nutrients supports the optimal functioning of these cells, while a diet lacking in vital components can impair their ability to perform their roles effectively. Regular physical activity enhances cardiovascular health by improving the function of cardiac muscle cells and endothelial cells, reducing the risk of heart disease. Conversely, sedentary lifestyles and poor dietary habits can lead to cellular dysfunction, increasing the risk of chronic diseases like diabetes, obesity, and certain types of cancer. Furthermore, genetic factors also play a significant role in the health and function of specialized cells. Genetic mutations can lead to disorders where specialized cells fail to perform their intended functions. For example, sickle cell anemia results from a mutation in hemoglobin-producing red blood cells, leading to abnormal cell shapes that impede blood flow and oxygen delivery. Understanding these genetic underpinnings is crucial for developing targeted therapies and preventive measures. In conclusion, the importance and functions of specialized cells are paramount in maintaining overall health and preventing disease. These cells operate in a highly coordinated manner to protect the body from external threats, regulate internal processes, and ensure optimal physiological function. By understanding how these cells work and how they can be supported through lifestyle choices and medical interventions, we can significantly enhance our overall well-being and reduce the risk of various diseases. This underscores the critical importance of specialized cells in the broader context of human health and disease prevention.