What Does Ppu Mean
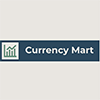
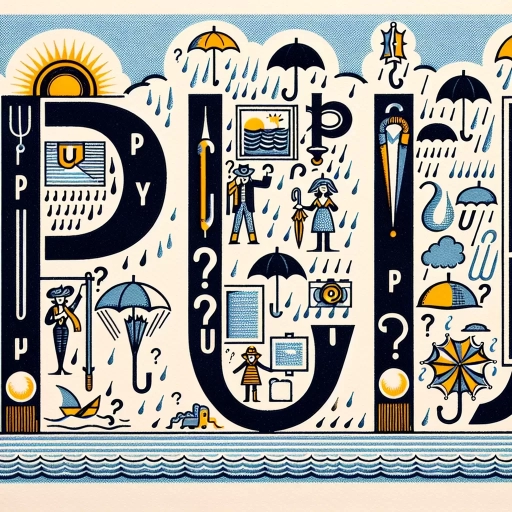
In the realm of technology and computing, acronyms often serve as gatekeepers to complex concepts. One such term that has garnered significant attention in recent years is "PPU," or Pixel Processing Unit. While it may seem like just another technical jargon, understanding what PPU means can unlock a wealth of knowledge about how modern computing systems handle graphical data. This article delves into the multifaceted world of PPU, starting with the foundational aspects that define its core functionality. We will explore the **Understanding the Basics of PPU**, where we dissect the fundamental principles behind this technology. Moving forward, we will delve into the **Technical Aspects of PPU**, examining the intricate mechanisms and architectures that make it tick. Finally, we will discuss the **Applications and Impact of PPU**, highlighting how this technology influences various fields from gaming to scientific simulations. By grasping these elements, readers will gain a comprehensive understanding of what PPU means and its pivotal role in contemporary computing. Let us begin by **Understanding the Basics of PPU**.
Understanding the Basics of PPU
In the realm of technology and engineering, acronyms often serve as gatekeepers to complex concepts, and PPU (Physics Processing Unit) is no exception. To fully grasp the significance and functionality of a PPU, it is essential to delve into its core components. This article aims to provide a comprehensive understanding by breaking down the basics of PPU through three key aspects. First, we will explore the **Definition and Acronym Expansion**, clarifying what PPU stands for and its fundamental role in processing physics-related data. Next, we will delve into the **Historical Context and Development**, tracing the evolution of PPUs from their inception to their current state. Finally, we will examine **Common Usage Across Industries**, highlighting how PPUs are integrated into various fields such as gaming, simulation, and scientific research. By understanding these elements, readers will gain a solid foundation in the principles of PPUs, enabling them to appreciate the intricacies and applications of these powerful processing units. This journey will ultimately lead to a deeper comprehension of **Understanding the Basics of PPU**.
Definition and Acronym Expansion
Understanding the basics of PPU (Power Processing Unit) begins with a solid grasp of definitions and acronym expansions, which are fundamental in deciphering technical jargon. In the context of computing and electronics, acronyms serve as shorthand for complex terms, enhancing communication efficiency among professionals. For instance, PPU itself stands for Power Processing Unit, a component designed to manage and optimize power consumption within electronic devices. This acronym encapsulates the unit's primary function: to process and regulate power efficiently. Expanding on this concept, other relevant acronyms include CPU (Central Processing Unit), which handles general computations; GPU (Graphics Processing Unit), specialized for graphics rendering; and MCU (Microcontroller Unit), a compact device integrating a CPU, memory, and input/output peripherals. Each of these components plays a distinct role in the overall functioning of electronic systems, and understanding their definitions is crucial for appreciating how they interact. Moreover, acronyms like SoC (System on Chip) and FPGA (Field-Programmable Gate Array) are also integral to modern electronics. SoC refers to an integrated circuit that combines multiple components into a single chip, enhancing performance and reducing size. FPGA, on the other hand, is a programmable logic device that can be configured post-manufacturing to perform specific tasks, offering flexibility in design. In the realm of power management, acronyms such as PMIC (Power Management Integrated Circuit) and DC-DC (Direct Current to Direct Current) converter are pivotal. PMICs are specialized ICs that manage power requirements for various components within a system, ensuring efficient energy use. DC-DC converters transform voltage levels from one DC source to another, which is essential for powering devices that require different voltage levels. The ability to expand and understand these acronyms not only clarifies technical documentation but also facilitates better communication among engineers, developers, and users. It allows for a deeper comprehension of how different components interact within a system, which is vital for designing, troubleshooting, and optimizing electronic devices. By mastering these definitions and expansions, one can gain a more nuanced understanding of the intricate mechanisms behind modern technology, ultimately enhancing their ability to work with and appreciate the complexity of systems like the PPU. This foundational knowledge serves as a stepping stone for more advanced topics in electronics and computing, making it an indispensable part of any technical education or professional development.
Historical Context and Development
The concept of PPU, or Processing Power Unit, has its roots deeply embedded in the historical context of computing and technological advancements. To understand the basics of PPU, it is crucial to delve into its development trajectory. The journey begins in the early 20th century with the advent of electronic computers, where processing power was primarily measured by the speed and efficiency of central processing units (CPUs). As technology evolved, so did the need for more specialized and efficient processing capabilities. In the 1970s and 1980s, the introduction of graphics processing units (GPUs) marked a significant milestone. Initially designed to handle graphical computations, GPUs were later recognized for their potential in parallel processing, which led to their adoption in various fields beyond graphics rendering. This shift highlighted the importance of dedicated processing units tailored to specific tasks, setting the stage for the concept of PPUs. The term "PPU" itself gained prominence with the introduction of the Nintendo Entertainment System (NES) in the 1980s. The Picture Processing Unit (PPU) in the NES was responsible for rendering graphics on the screen, demonstrating how specialized hardware could enhance performance in specific areas. This early implementation showcased the benefits of offloading certain tasks from the main CPU to dedicated units, thereby improving overall system efficiency. As computing technology continued to advance, the idea of PPUs expanded beyond gaming consoles. In modern computing, PPUs are often associated with various forms of specialized processing units such as GPUs, TPUs (Tensor Processing Units), and even FPGAs (Field-Programmable Gate Arrays). These units are designed to handle complex computations that traditional CPUs struggle with, such as machine learning algorithms, data encryption, and high-performance scientific simulations. The development of PPUs has been driven by the increasing demand for faster and more efficient processing in diverse fields. For instance, the rise of artificial intelligence and deep learning has necessitated the creation of TPUs by companies like Google, which are optimized for matrix operations essential in neural networks. Similarly, advancements in cryptocurrency mining have led to the development of Application-Specific Integrated Circuits (ASICs), another form of PPU tailored for specific computational tasks. In summary, the historical context and development of PPUs reflect a continuous pursuit of optimizing computational efficiency through specialization. From early graphics rendering in gaming consoles to current-day applications in AI and cryptocurrency, the evolution of PPUs underscores the importance of tailored processing units in enhancing performance and driving technological innovation. Understanding this historical backdrop is essential for grasping the basics of PPU and its role in modern computing landscapes.
Common Usage Across Industries
**Common Usage Across Industries** The term "PPU" (Pixels Per Unit) is a versatile metric that finds application across various industries, each leveraging its unique benefits to enhance performance, efficiency, and quality. In the **graphics and gaming industry**, PPU is crucial for measuring the resolution and detail of textures and models. Higher PPU values indicate more detailed graphics, which is essential for creating immersive gaming experiences and realistic visual effects. This metric helps developers optimize their games for different hardware configurations, ensuring that the visual quality remains consistent across various platforms. In **manufacturing and engineering**, PPU is used in the context of 3D printing and CNC machining. Here, it refers to the resolution or precision of the printing or machining process. A higher PPU means finer details can be achieved, which is vital for producing complex parts with high accuracy. This application is particularly important in industries like aerospace and automotive, where precision is paramount. **Medical imaging** also relies heavily on PPU, particularly in modalities such as MRI and CT scans. The resolution of these images, measured in pixels per unit, directly affects the diagnostic accuracy. Higher resolution images provide clearer details of anatomical structures, enabling healthcare professionals to make more accurate diagnoses and develop effective treatment plans. In **geospatial analysis and mapping**, PPU is used to measure the resolution of satellite or aerial imagery. This metric is critical for applications such as land use planning, environmental monitoring, and disaster response. High-resolution imagery (higher PPU) allows for more precise identification of features on the ground, which is essential for making informed decisions in these fields. **Digital signage and display technology** also benefit from understanding PPU. The pixel density of displays, measured in PPU, determines the clarity and readability of content from various distances. This is particularly important for outdoor displays or large indoor screens where viewers may be far from the screen. Across these diverse industries, the common thread is the importance of precision and detail. Whether it's enhancing visual fidelity in gaming, ensuring precision in manufacturing, improving diagnostic accuracy in medicine, or providing clear imagery in geospatial analysis, PPU serves as a critical metric that helps professionals achieve their goals by quantifying and optimizing the level of detail in their work. Understanding PPU is thus fundamental to leveraging its full potential and driving innovation across multiple sectors.
Technical Aspects of PPU
In the realm of modern computing, the Processing Power Unit (PPU) stands as a critical component, driving the efficiency and performance of various technological systems. Understanding the technical aspects of PPU is essential for optimizing system performance, ensuring reliability, and staying ahead in the competitive landscape of technology. This article delves into three key areas that form the backbone of PPU technology: **Architecture and Design Principles**, which explore how the structural layout and fundamental design choices impact functionality; **Performance Metrics and Benchmarks**, which discuss how to measure and evaluate the effectiveness of a PPU; and **Comparison with Other Processing Units**, which provides insights into how PPUs stack up against other processing technologies. By examining these aspects, readers will gain a comprehensive understanding of what makes a PPU tick and how it contributes to overall system performance. This foundational knowledge is crucial for anyone looking to grasp the intricacies of PPU technology, setting the stage for a deeper dive into **Understanding the Basics of PPU**.
Architecture and Design Principles
In the realm of technical aspects, particularly when discussing the **Technical Aspects of PPU (Programmable Processing Unit)**, it is crucial to delve into the foundational principles that govern its design and architecture. Architecture and design principles are the bedrock upon which any sophisticated system, including PPUs, is built. These principles ensure that the system is not only functional but also efficient, scalable, and maintainable. At the heart of PPU architecture lies a deep understanding of **modularity** and **hierarchy**. Modularity allows for the division of complex tasks into simpler, more manageable components. This approach facilitates easier development, testing, and maintenance by enabling developers to focus on individual modules without affecting the entire system. Hierarchy, on the other hand, organizes these modules in a structured manner, ensuring clear communication pathways and reducing complexity. **Scalability** is another critical principle in PPU design. As technology evolves and demands increase, a scalable architecture allows for seamless integration of new components or upgrades without compromising performance. This is particularly important for PPUs, which are often tasked with handling diverse and dynamic workloads. **Symmetry** and **redundancy** are also key considerations. Symmetry in design ensures that resources are utilized efficiently and that the system can handle various types of data or tasks uniformly. Redundancy provides a safety net against failures by duplicating critical components or pathways, thereby ensuring continuous operation even in the event of component failure. Furthermore, **energy efficiency** and **thermal management** are vital design principles for PPUs. Given their high computational intensity, PPUs can generate significant heat and consume substantial power. Effective thermal management through innovative cooling solutions and energy-efficient design techniques are essential to maintain performance while minimizing environmental impact. Lastly, **flexibility** and **adaptability** are paramount in PPU architecture. These units must be capable of adapting to different programming models and workloads, making them versatile tools in various applications. This adaptability ensures that the PPU remains relevant as technological landscapes shift. Incorporating these architectural and design principles into the development of PPUs not only enhances their technical capabilities but also ensures they are robust, reliable, and future-proof. By adhering to these principles, engineers can create PPUs that are not just powerful processing units but also integral components of sophisticated systems that drive innovation across multiple industries.
Performance Metrics and Benchmarks
**Performance Metrics and Benchmarks** In the context of Technical Aspects of PPU (Peripheral Processing Unit), understanding performance metrics and benchmarks is crucial for evaluating and optimizing the efficiency of these specialized processing units. Performance metrics serve as quantifiable measures that help in assessing the effectiveness and efficiency of a PPU in performing its designated tasks. Key metrics include throughput, latency, power consumption, and computational intensity. Throughput, for instance, measures the rate at which a PPU can process data or complete tasks, while latency focuses on the time it takes for the PPU to respond to input or complete a task. Power consumption is vital for ensuring that the PPU operates within energy constraints, especially in embedded systems or mobile devices. Benchmarks, on the other hand, provide standardized tests that allow for comparative analysis across different PPUs. These benchmarks simulate real-world scenarios and stress various aspects of the PPU's performance. For example, benchmarks like SPEC (Standard Performance Evaluation Corporation) and MLPerf (Machine Learning Performance) are widely used to evaluate the performance of PPUs in specific domains such as machine learning and general-purpose computing. By running these benchmarks, developers can identify bottlenecks, optimize code, and make informed decisions about hardware upgrades or design improvements. Moreover, performance metrics and benchmarks help in setting realistic expectations and goals for PPU development. They enable engineers to fine-tune architectural designs, optimize software frameworks, and ensure that the PPU meets the required specifications for its intended application. For instance, in automotive systems where real-time processing is critical, benchmarks can help validate whether a PPU can handle complex tasks like object detection and tracking within stringent time constraints. In addition to these technical aspects, performance metrics and benchmarks also play a significant role in market positioning and customer satisfaction. By publishing benchmark results, manufacturers can demonstrate the superiority of their PPUs over competitors, thereby influencing purchasing decisions. This transparency also fosters trust among users who rely on these metrics to make informed choices about which hardware to adopt for their specific needs. In summary, performance metrics and benchmarks are indispensable tools in the development and evaluation of PPUs. They provide a structured approach to measuring performance, identifying areas for improvement, and ensuring that these specialized processing units meet the demanding requirements of modern applications. By leveraging these metrics and benchmarks effectively, developers can create highly efficient and reliable PPUs that drive innovation across various industries.
Comparison with Other Processing Units
When comparing the Processing Power Unit (PPU) to other processing units, several key differences and similarities emerge that highlight its unique strengths and applications. Unlike Central Processing Units (CPUs), which are general-purpose processors designed to handle a wide range of tasks, the PPU is specialized for specific types of computations. For instance, Graphics Processing Units (GPUs) are optimized for parallel processing and are particularly adept at handling the complex matrix operations required in graphics rendering and machine learning. In contrast, the PPU is often tailored for tasks that require high-speed data processing in real-time, such as those found in embedded systems or specialized computing environments. In terms of performance, the PPU typically outperforms CPUs in tasks that require rapid data throughput and low latency, making it an ideal choice for applications like signal processing, scientific simulations, and real-time analytics. However, CPUs remain superior in tasks that demand high single-thread performance and complex instruction sets. Another comparison can be drawn with Field-Programmable Gate Arrays (FPGAs), which offer reconfigurable hardware that can be optimized for specific algorithms. While FPGAs provide flexibility and energy efficiency, PPUs are generally easier to program and integrate into existing systems due to their software-friendly architecture. The PPU also stands out from Application-Specific Integrated Circuits (ASICs) in its balance between performance and programmability. ASICs are highly optimized for specific tasks but lack the flexibility of PPUs, which can be reprogrammed to adapt to changing requirements without the need for hardware redesign. This adaptability makes PPUs particularly valuable in environments where the computational workload is dynamic or evolving. Furthermore, the power consumption of PPUs is often lower compared to other specialized processors like GPUs, especially when considering the performance-per-watt metric. This efficiency is crucial in battery-powered devices or data centers where energy costs are significant. Additionally, the thermal design of PPUs allows them to operate within tighter thermal envelopes, making them suitable for compact form factors and harsh environmental conditions. In summary, while other processing units excel in their respective domains—CPUs in general-purpose computing, GPUs in parallel processing, FPGAs in reconfigurability, and ASICs in absolute performance—the PPU distinguishes itself through its unique blend of high-speed data processing capabilities, low latency, ease of programming, and energy efficiency. These attributes make the PPU an indispensable component in various technical applications requiring real-time data handling and specialized computational tasks.
Applications and Impact of PPU
In the rapidly evolving landscape of technology, the emergence of Processing-in-Memory (PIM) and its variant, Processing-in-Storage (PIS), has given rise to a new paradigm: Processing Power Units (PPU). These innovative units are revolutionizing data processing by integrating computation directly into memory and storage, significantly enhancing efficiency and reducing latency. The applications and impact of PPU are multifaceted and far-reaching, touching various fields such as artificial intelligence, big data analytics, and cloud computing. This article delves into the real-world use cases of PPU across diverse industries, exploring how they are transforming operational dynamics. It also examines future trends and innovations that are set to further amplify the capabilities of PPU. Additionally, we will discuss the economic and social implications of widespread PPU adoption, highlighting both the benefits and challenges associated with this technological shift. To fully appreciate these advancements, it is crucial to first understand the basics of PPU, which will be explored in detail following this introduction. By grasping the fundamental principles of PPU, readers will be better equipped to appreciate the profound impact these units are having and will continue to have in the future.
Real-World Use Cases in Various Fields
In the realm of modern technology, Processing Power Units (PPUs) have emerged as a cornerstone in various fields, revolutionizing how data is processed and analyzed. One of the most significant real-world use cases is in **Artificial Intelligence (AI) and Machine Learning (ML)**. PPUs are designed to handle the intensive computational demands of AI algorithms, enabling faster training times and more accurate model outputs. For instance, in healthcare, PPUs facilitate the rapid analysis of medical images, such as MRIs and CT scans, to diagnose diseases like cancer more accurately and earlier than traditional methods. This not only improves patient outcomes but also streamlines clinical workflows. In **Finance**, PPUs play a crucial role in high-frequency trading and risk analysis. They process vast amounts of financial data in real-time, allowing traders to make informed decisions quickly and mitigate potential risks. This capability is particularly valuable during market volatility, where milliseconds can make a significant difference in trading outcomes. Additionally, PPUs enhance fraud detection systems by analyzing transaction patterns at unprecedented speeds, protecting financial institutions and their customers from cyber threats. The **Automotive Industry** also benefits greatly from PPUs, especially in the development of autonomous vehicles. These units process sensor data from cameras, lidar, and radar in real-time, enabling vehicles to navigate safely and efficiently. This technology is pivotal for achieving Level 5 autonomy, where vehicles can operate without human intervention under all conditions. Moreover, PPUs improve vehicle-to-everything (V2X) communication, enhancing safety features like collision avoidance and traffic management. In **Scientific Research**, PPUs are instrumental in simulating complex phenomena such as climate models and molecular dynamics. They accelerate the processing of large datasets, allowing scientists to conduct more detailed and accurate simulations. This has led to breakthroughs in fields like climate science and materials engineering, where researchers can now predict weather patterns and material properties with greater precision. Furthermore, **Cybersecurity** relies heavily on PPUs to detect and respond to threats in real-time. Advanced threat detection systems powered by PPUs can analyze network traffic patterns to identify anomalies indicative of cyber attacks. This proactive approach helps protect sensitive data and prevents breaches before they occur. Lastly, in **Gaming**, PPUs enhance the gaming experience by providing faster rendering times and more realistic graphics. They handle complex game physics and simulations efficiently, allowing for smoother gameplay and more immersive experiences. In summary, PPUs are transforming various industries by providing the computational power needed to process vast amounts of data quickly and accurately. Their applications span from healthcare and finance to automotive and scientific research, each contributing significantly to innovation and efficiency in their respective fields. As technology continues to evolve, the impact of PPUs will only grow, driving further advancements across multiple sectors.
Future Trends and Innovations
As we delve into the applications and impact of Processing Power Units (PPUs), it becomes evident that future trends and innovations will significantly shape their role in various industries. One of the most promising trends is the integration of PPUs with artificial intelligence (AI) and machine learning (ML) technologies. This synergy will enable more efficient data processing, enhanced predictive analytics, and real-time decision-making capabilities. For instance, in healthcare, AI-driven PPUs can analyze vast amounts of medical data to identify patterns and predict patient outcomes, leading to personalized and more effective treatment plans. Another trend is the adoption of edge computing, where PPUs will play a crucial role in processing data closer to where it is generated. This approach reduces latency, improves real-time processing, and enhances security by minimizing the amount of data transmitted over networks. In industrial settings, edge computing with PPUs can optimize manufacturing processes by analyzing sensor data in real-time, allowing for immediate adjustments and improving overall efficiency. The Internet of Things (IoT) is another area where PPUs will see significant innovation. As IoT devices proliferate, the need for robust and efficient processing units to handle the sheer volume of data generated becomes paramount. PPUs will be instrumental in managing this data deluge, ensuring seamless communication between devices and enabling smart cities, homes, and industries to operate more intelligently. Furthermore, advancements in quantum computing are set to revolutionize the capabilities of PPUs. Quantum PPUs will offer exponential increases in processing power, solving complex problems that are currently intractable with traditional computing. This will have profound implications for fields such as cryptography, materials science, and climate modeling, where complex simulations and data analyses are critical. In addition to these technological advancements, sustainability is becoming a key focus area for future PPU innovations. As energy consumption and environmental impact become increasingly important considerations, PPUs are being designed with energy efficiency in mind. This includes the development of low-power architectures and the use of renewable energy sources to power data centers and edge computing devices. Lastly, the integration of PPUs with emerging technologies like blockchain and 5G networks will further expand their applications. Blockchain-PPU combinations can enhance data security and transparency, while 5G networks will provide the high-speed connectivity necessary for real-time data processing across diverse applications. In summary, the future of PPUs is marked by exciting trends and innovations that promise to transform various sectors. From AI and edge computing to quantum processing and sustainable designs, these advancements will not only enhance the performance of PPUs but also drive new applications and impacts across multiple industries. As technology continues to evolve, the role of PPUs will become even more pivotal in shaping the digital landscape of tomorrow.
Economic and Social Implications
The economic and social implications of PPU (Personal Protective Equipment) are multifaceted and far-reaching, particularly in the context of public health crises and industrial safety. On the economic front, the demand for PPE has surged significantly during global health emergencies such as the COVID-19 pandemic, leading to substantial investments in manufacturing and distribution. This increased demand has created new job opportunities in the healthcare and manufacturing sectors, contributing to economic growth. However, it also poses challenges such as supply chain disruptions and price inflation, which can strain national budgets and impact consumer spending. From a social perspective, PPE plays a crucial role in safeguarding public health by reducing the transmission of infectious diseases. This not only protects healthcare workers but also ensures that essential services continue uninterrupted, maintaining societal stability. The widespread use of PPE has also led to changes in social norms and behaviors, with mask-wearing becoming a common practice in many countries. This shift underscores the importance of community adherence to health guidelines and fosters a sense of collective responsibility for public health. Moreover, the availability and accessibility of PPE can exacerbate existing social inequalities. In low-income communities, access to quality PPE may be limited due to financial constraints, placing these populations at higher risk of infection. This disparity highlights the need for equitable distribution policies and subsidies to ensure that all segments of society have adequate protection. In addition, the environmental impact of PPE cannot be overlooked. The increased production and disposal of single-use masks, gloves, and other protective gear contribute to plastic waste and pollution. This has prompted calls for sustainable alternatives and better waste management practices to mitigate these effects. Overall, the economic and social implications of PPE are intertwined with broader societal issues such as public health policy, economic resilience, social equity, and environmental sustainability. As technology continues to evolve and new applications emerge for PPE beyond traditional healthcare settings—such as in education and retail—the importance of addressing these implications will only grow. By understanding these dynamics, policymakers can develop more comprehensive strategies that balance economic needs with social welfare and environmental stewardship.