What Are Metalloids
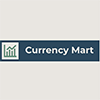
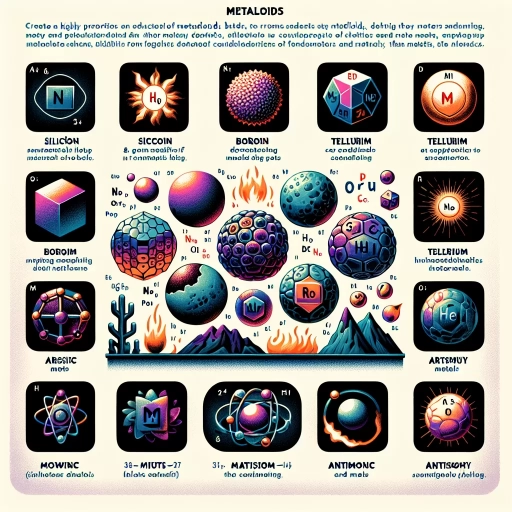
Metalloids, often referred to as semimetals, occupy a unique position in the periodic table, bridging the gap between metals and nonmetals. These elements exhibit a blend of characteristics from both groups, making them fascinating subjects for study and application. Understanding metalloids begins with their definition and classification, which sets the stage for exploring their distinct physical and chemical properties. These properties are crucial in determining their behavior and reactivity, which in turn influence their various applications across different fields. From electronics and solar panels to pharmaceuticals and environmental science, metalloids play pivotal roles due to their versatility and specific attributes. This article delves into the world of metalloids, starting with their definition and classification, to provide a comprehensive overview of these intriguing elements and their significance in modern science and technology. By examining their physical and chemical properties, as well as their diverse applications, we can appreciate the importance of metalloids in our daily lives and future innovations. Let us begin by defining and classifying these unique elements to lay the groundwork for a deeper exploration of their properties and uses.
Definition and Classification of Metalloids
Metalloids, often referred to as semimetals, occupy a unique position in the periodic table, exhibiting properties that bridge the gap between metals and nonmetals. Understanding these elements requires a multifaceted approach, delving into their historical background, chemical properties, and their placement within the periodic table. Historically, the term "metalloid" has evolved over time, reflecting changes in scientific understanding and classification methods. The chemical properties of metalloids are distinct, showcasing a mix of metallic and nonmetallic characteristics that set them apart from other elements. Furthermore, their position in the periodic table is crucial for understanding their behavior and interactions. By exploring these aspects, we can gain a comprehensive insight into the definition and classification of metalloids, which is essential for both theoretical and practical applications in chemistry and materials science. This article will delve into these key areas, providing a detailed examination of the historical background and evolution of the term, the chemical properties and characteristics of metalloids, and their strategic position in the periodic table, ultimately leading to a clear definition and classification of these intriguing elements.
Historical Background and Evolution of the Term
The term "metalloid" has a rich and evolving historical background that reflects the gradual refinement of chemical understanding over centuries. The concept of metalloids emerged as scientists began to distinguish between metals and nonmetals, recognizing a group of elements that exhibited properties intermediate between these two categories. In the early days of chemistry, the distinction between metals and nonmetals was not as clear-cut, with many elements being classified based on their physical properties such as malleability, ductility, and conductivity. The term "metalloid" itself was first introduced in the mid-19th century by the English chemist William Odling in 1850. Odling used this term to describe elements like arsenic, antimony, and tellurium, which did not fit neatly into the traditional metal or nonmetal classifications. These elements were found to possess some metallic characteristics, such as forming alloys with metals, but they also exhibited nonmetallic traits like forming anions in compounds. As the periodic table developed, particularly with the work of Dmitri Mendeleev in the late 19th century, the classification of metalloids became more systematic. Mendeleev's periodic table helped to organize elements based on their atomic weights and recurring chemical properties, highlighting the transitional nature of metalloids. The elements now recognized as metalloids—boron, silicon, germanium, arsenic, antimony, tellurium, and sometimes polonium and astatine—were placed along the "staircase" line that demarcates metals from nonmetals in the periodic table. The evolution of the term continued with advancements in atomic theory and the discovery of new elements. The development of quantum mechanics in the 20th century provided a deeper understanding of the electronic structures of atoms, further clarifying why certain elements exhibit intermediate properties. Modern definitions and classifications of metalloids are based on a combination of physical and chemical criteria, including their position in the periodic table, electron configuration, and typical chemical behavior. Today, the study of metalloids is crucial in various fields such as materials science and semiconductor technology. Metalloids like silicon and germanium are key components in electronic devices due to their unique ability to act as semiconductors—materials that can control the flow of electrical current. This practical significance underscores the importance of understanding the historical development and precise classification of metalloids, which has been refined over time through scientific inquiry and technological innovation. The ongoing research into these elements continues to expand our knowledge and applications of metalloids, solidifying their place as a distinct and important group within the periodic table.
Chemical Properties and Characteristics
Chemical properties and characteristics are pivotal in understanding the unique nature of metalloids, which occupy a distinct position between metals and nonmetals in the periodic table. Metalloids, also known as semimetals, exhibit a blend of metallic and nonmetallic traits that set them apart from their more extreme counterparts. One of the defining chemical properties of metalloids is their intermediate electrical conductivity. Unlike metals, which are excellent conductors, and nonmetals, which are generally poor conductors, metalloids display moderate conductivity. This characteristic is particularly evident in elements like silicon and germanium, which are widely used in semiconductor technology due to their ability to control the flow of electrical current. Another significant aspect of metalloids is their reactivity. While they do not readily form ions like metals or share electrons as freely as nonmetals, metalloids can engage in covalent bonding with other elements. For instance, boron and silicon form covalent bonds with oxygen to produce boron oxide and silicon dioxide, respectively. These compounds are crucial in various industrial applications, including ceramics and glass production. The ability of metalloids to form alloys with metals further underscores their versatility; for example, arsenic is often alloyed with lead to enhance its durability and resistance to corrosion. The physical characteristics of metalloids also reflect their intermediate nature. They typically exhibit a metallic luster but are brittle and only moderately malleable, unlike the highly malleable metals. Additionally, metalloids have relatively high melting points compared to nonmetals but lower than those of metals. This combination of physical and chemical properties makes metalloids invaluable in diverse technological fields. For example, germanium is used in fiber optic cables due to its transparency to infrared light, while antimony is employed in flame retardants because of its ability to inhibit combustion. In terms of classification, understanding the chemical properties and characteristics of metalloids helps in distinguishing them from metals and nonmetals. The most commonly recognized metalloids are boron, silicon, germanium, arsenic, antimony, tellurium, and sometimes polonium and astatine. These elements are typically found along the "staircase" line that demarcates metals from nonmetals on the periodic table. Their unique blend of properties makes them essential components in modern technology, from electronics and solar panels to pharmaceuticals and environmental protection. In summary, the chemical properties and characteristics of metalloids—such as intermediate conductivity, covalent bonding capabilities, and specific physical traits—highlight their unique position within the periodic table. These attributes not only define metalloids but also underscore their critical role in various technological and industrial applications, making them an indispensable part of modern science and engineering.
Position in the Periodic Table
Position in the Periodic Table is a crucial determinant for understanding the properties and classification of metalloids. Metalloids, also known as semimetals, are elements that exhibit some properties of metals and some properties of nonmetals. They are located on the periodic table along a diagonal line that separates metals from nonmetals, often referred to as the "staircase" or "borderline" between these two groups. This strategic positioning reflects their intermediate nature in terms of electron configuration and chemical behavior. Starting from the upper left corner of the periodic table, moving diagonally down to the lower right, we find the metalloids: Boron (B), Silicon (Si), Germanium (Ge), Arsenic (As), Antimony (Sb), Tellurium (Te), and sometimes Polonium (Po) and Astatine (At) are also included. This diagonal trend is significant because it aligns with changes in atomic radius, electronegativity, and electron affinity that distinguish metalloids from their neighboring elements. For instance, as you move from left to right across a period, elements generally become less metallic and more nonmetallic due to increased electronegativity and electron affinity. The position of metalloids in the periodic table also influences their physical and chemical properties. Unlike metals, which are typically good conductors of electricity and have high malleability and ductility, metalloids are generally poor conductors or semiconductors. They often exhibit intermediate values for physical properties such as melting points and densities. Chemically, metalloids can form ions with charges that are intermediate between those of metals and nonmetals, and they can participate in both ionic and covalent bonding. Understanding the position of metalloids in the periodic table is essential for predicting their behavior in various chemical reactions and applications. For example, Silicon, a key metalloid, is pivotal in semiconductor technology due to its ability to conduct electricity under certain conditions but not others. Similarly, Germanium and Arsenic are used in electronic devices because of their unique electrical properties. In summary, the position of metalloids on the periodic table is a defining characteristic that underpins their unique blend of metallic and nonmetallic traits. This positioning not only aids in their identification but also explains their distinctive physical and chemical behaviors, making them invaluable in various technological and industrial applications.
Physical and Chemical Properties of Metalloids
Metalloids, a unique group of elements situated on the border between metals and nonmetals in the periodic table, exhibit a fascinating blend of physical and chemical properties. These elements, which include boron, silicon, germanium, arsenic, antimony, tellurium, and sometimes polonium and astatine, are characterized by their intermediate characteristics. This article delves into the intricate details of metalloids, focusing on three key aspects: their electrical and thermal conductivity, their reactivity with other elements, and their mechanical properties along with their practical uses. Understanding these properties is crucial for appreciating the versatility and importance of metalloids in various technological and industrial applications. By exploring these facets, we gain insight into why metalloids are so valuable in fields such as electronics, semiconductors, and advanced materials. This comprehensive overview will transition seamlessly into a detailed discussion on the definition and classification of metalloids, providing a solid foundation for understanding their unique place within the periodic table.
Electrical and Thermal Conductivity
Metalloids, situated at the border between metals and nonmetals on the periodic table, exhibit unique physical and chemical properties that set them apart from their more traditional counterparts. One of the most intriguing aspects of metalloids is their electrical and thermal conductivity. Unlike metals, which are typically excellent conductors due to their free-flowing electrons, metalloids display intermediate conductivity characteristics. For instance, silicon and germanium, two well-known metalloids, have electrical conductivities that are significantly lower than those of metals but higher than those of insulators. This intermediate behavior makes them crucial in semiconductor technology, where they are used to control the flow of electrical current in devices such as transistors and solar cells. Thermally, metalloids also show a balance between the high thermal conductivity of metals and the low thermal conductivity of nonmetals. Boron, for example, has a relatively low thermal conductivity compared to metals like copper or aluminum but is still more conductive than many nonmetallic materials. This property is advantageous in applications where moderate heat transfer is required without the need for high electrical conductivity. The intermediate thermal conductivity of metalloids can also be beneficial in composite materials, where they can help distribute heat evenly without compromising electrical insulation. The unique electrical and thermal properties of metalloids are largely due to their electronic structure. Metalloids have a partially filled valence band, which allows them to conduct electricity under certain conditions but not as freely as metals. This partial filling also influences their thermal behavior, as the electrons in the valence band can participate in heat transfer processes to a limited extent. Additionally, the ability of metalloids to form alloys and compounds with other elements further enhances their conductivity properties, making them versatile materials in various technological applications. In summary, the electrical and thermal conductivity of metalloids are key factors that distinguish them from metals and nonmetals. Their intermediate conductivity makes them indispensable in semiconductor technology and other fields where controlled electrical and thermal properties are essential. Understanding these properties is crucial for harnessing the full potential of metalloids in modern technology and engineering.
Reactivity with Other Elements
Metalloids, situated at the border between metals and nonmetals on the periodic table, exhibit unique reactivity patterns that distinguish them from their more metallic or nonmetallic counterparts. This reactivity is a critical aspect of their physical and chemical properties, influencing how they interact with other elements and compounds. When metalloids react with metals, they often form alloys or intermetallic compounds. For instance, silicon (Si) can form silicides with various metals like iron (Fe) and titanium (Ti), which are used in high-temperature applications due to their enhanced strength and resistance to corrosion. Similarly, germanium (Ge) forms alloys with metals such as aluminum (Al) and copper (Cu), which are crucial in semiconductor technology. In contrast, reactions between metalloids and nonmetals typically result in covalent compounds. Boron (B), for example, reacts with nitrogen (N) to form boron nitride (BN), a material known for its hardness and thermal stability. Silicon reacts with oxygen (O) to form silicon dioxide (SiO2), commonly known as quartz or sand, which is a fundamental component of many minerals and glasses. These covalent compounds highlight the ability of metalloids to share electrons effectively, leading to the formation of stable molecular structures. The reactivity of metalloids with other elements is also influenced by their ability to act as semiconductors. This property allows them to control the flow of electrical current, making them indispensable in electronic devices. For instance, arsenic (As) and antimony (Sb) are used in semiconductor materials due to their intermediate electrical conductivity between metals and insulators. Furthermore, metalloids can exhibit amphoteric behavior, meaning they can react with both acids and bases. Germanium, for example, reacts with hydrochloric acid (HCl) to form germanium tetrachloride (GeCl4), but it also reacts with sodium hydroxide (NaOH) to form sodium germanate (Na2GeO3). This dual reactivity underscores the versatile nature of metalloids in various chemical environments. In summary, the reactivity of metalloids with other elements is characterized by their ability to form a wide range of compounds, from alloys with metals to covalent molecules with nonmetals. Their semiconductor properties and amphoteric behavior further enhance their utility across diverse applications, from electronics and materials science to chemistry and engineering. Understanding these reactivity patterns is essential for harnessing the unique properties of metalloids in technological advancements and scientific research.
Mechanical Properties and Uses
Metalloids, situated at the border between metals and nonmetals on the periodic table, exhibit a unique set of mechanical properties that distinguish them from their metallic and nonmetallic counterparts. These properties are crucial for understanding their diverse applications across various industries. One of the key mechanical properties of metalloids is their intermediate hardness. Unlike metals, which are generally soft and malleable, metalloids are harder and more brittle. For instance, silicon, a common metalloid, has a hardness similar to that of glass, making it resistant to wear but also prone to cracking under stress. This property makes silicon ideal for use in semiconductor devices where high purity and stability are required. Germanium, another metalloid, is used in fiber optic systems due to its ability to maintain structural integrity under thermal stress. The electrical conductivity of metalloids is another significant mechanical property. Unlike metals, which are excellent conductors, metalloids are semiconductors, meaning their electrical conductivity lies between that of metals and insulators. This characteristic allows them to control the flow of electrical current, making them indispensable in electronic devices such as transistors, diodes, and solar cells. For example, silicon-based semiconductors are the backbone of modern electronics, enabling the creation of complex integrated circuits that power everything from smartphones to supercomputers. In addition to their electrical properties, metalloids also exhibit unique thermal characteristics. Boron, for instance, has a high melting point and is used in high-temperature applications such as in the production of fiberglass and ceramics. Arsenic, on the other hand, has a relatively low melting point but is highly toxic, limiting its use primarily to specialized applications like pesticides and certain types of glass. The mechanical strength and durability of metalloids make them valuable in various industrial contexts. For example, silicon carbide (carborundum), a compound formed from silicon and carbon, is one of the hardest substances known and is used as an abrasive in cutting tools and grinding wheels. Similarly, germanium's high strength-to-weight ratio makes it suitable for aerospace applications where lightweight yet robust materials are essential. In summary, the mechanical properties of metalloids—such as intermediate hardness, semiconductor behavior, and unique thermal characteristics—make them versatile materials with a wide range of applications. From electronics and semiconductors to high-temperature materials and abrasives, metalloids play a critical role in modern technology and industry, underscoring their importance in the physical and chemical properties that define them.
Applications and Importance of Metalloids
Metalloids, a unique group of elements that exhibit properties intermediate between metals and nonmetals, play a pivotal role in various technological, industrial, and biological contexts. Their versatility and distinct characteristics make them indispensable in several key areas. In the realm of electronics and semiconductors, metalloids such as silicon and germanium are crucial for the fabrication of microchips and other electronic devices, enabling the modern digital age. Additionally, metalloids are integral components in the formation of alloys and compounds, enhancing the strength, durability, and conductivity of materials used in diverse applications. Furthermore, their environmental and biological significance cannot be overstated; metalloids like arsenic and antimony have critical roles in ecosystems and human health, albeit with potential toxic implications. Understanding the applications and importance of metalloids is essential for advancing technology, ensuring environmental sustainability, and promoting public health. To delve deeper into these aspects, it is first necessary to explore the definition and classification of metalloids, which will provide a foundational understanding of their unique properties and behaviors.
Role in Electronics and Semiconductors
Metalloids play a pivotal role in the realm of electronics and semiconductors, underpinning the technological advancements that drive modern society. These elements, situated at the boundary between metals and nonmetals on the periodic table, exhibit unique properties that make them indispensable in various electronic applications. Silicon, one of the most well-known metalloids, is the backbone of the semiconductor industry. Its ability to act as a semiconductor when doped with other elements allows it to control the flow of electrical current, making it crucial for the production of microchips, transistors, and solar cells. These components are integral to everything from smartphones and computers to medical devices and renewable energy systems. Germanium, another metalloid, is also significant in semiconductor technology due to its high carrier mobility and low thermal noise. It is often used in high-speed transistors and diodes, particularly in applications requiring high-frequency operation such as satellite communications and radar systems. Additionally, germanium's optical properties make it valuable in fiber optic systems, enabling the efficient transmission of data over long distances. Boron, a metalloid with a wide range of applications, is used in the doping process for silicon semiconductors. By introducing boron into silicon crystals, manufacturers can create p-type semiconductors that are essential for the fabrication of integrated circuits. This process enhances the conductivity of silicon, allowing for the creation of complex electronic devices with precise control over electrical current. Arsenic and antimony are also critical in semiconductor manufacturing. Arsenic is used to create n-type semiconductors when doped into silicon, complementing boron-doped p-type semiconductors to form the basis of modern electronic devices. Antimony, with its high thermal stability, is employed in lead-free soldering alloys and as a dopant in semiconductor materials, ensuring reliable performance under various environmental conditions. The importance of metalloids in electronics extends beyond their role in semiconductors. They are integral to the development of advanced materials and technologies such as photovoltaic cells, thermoelectric devices, and nanotechnology. For instance, tellurium, when combined with other elements like cadmium or selenium, forms compounds used in solar panels and other photovoltaic applications, contributing to the global shift towards renewable energy sources. In summary, metalloids are the linchpin of modern electronics and semiconductor technology. Their unique electrical and thermal properties make them irreplaceable in the fabrication of electronic devices that power our daily lives. As technology continues to evolve, the role of metalloids will only become more pronounced, driving innovation and efficiency in fields ranging from computing and communication to energy and healthcare.
Use in Alloys and Compounds
Metalloids play a crucial role in the creation of various alloys and compounds, significantly enhancing their properties and applications. One of the most notable uses of metalloids is in semiconductor technology, where silicon, a key metalloid, is the backbone of modern electronics. Silicon's ability to conduct electricity under certain conditions makes it ideal for manufacturing microchips, solar panels, and other electronic devices. Another metalloid, germanium, is often used in combination with silicon to improve the performance of semiconductors, particularly in high-speed applications. In the realm of alloys, metalloids like boron and silicon are essential components. Boron, for instance, is used in steel production to enhance hardness and resistance to wear and tear. It forms boron carbide, one of the hardest substances known, which is utilized in body armor and vehicle armor due to its exceptional strength-to-weight ratio. Silicon, on the other hand, is a critical element in the production of ferrosilicon, an alloy used in the manufacture of steel and cast iron to improve their strength and durability. Arsenic, another metalloid, has historical significance in the production of alloys. Although its toxicity has limited its modern use, arsenic was once used to harden copper and bronze, making these metals more suitable for tools and weapons. Additionally, antimony is used in lead-acid batteries to improve their performance and lifespan by enhancing the durability of the battery plates. The versatility of metalloids extends beyond industrial applications; they also find use in various chemical compounds. Tellurium, for example, is combined with cadmium to form cadmium telluride (CdTe), a semiconductor material used in photovoltaic cells due to its high efficiency in converting sunlight into electricity. Similarly, selenium is used in photocopiers and laser printers as a key component in the xerographic process. In summary, metalloids are indispensable in creating alloys and compounds that drive technological advancements and industrial processes. Their unique properties allow them to enhance the performance of materials across diverse fields, from electronics and energy storage to construction and manufacturing. The importance of metalloids in these applications underscores their critical role in modern technology and industry, highlighting their significance beyond their intrinsic chemical characteristics.
Environmental and Biological Significance
Metalloids, situated at the boundary between metals and nonmetals on the periodic table, hold significant environmental and biological importance. These elements, including boron, silicon, germanium, arsenic, antimony, tellurium, and polonium, play crucial roles in various ecological processes and biological systems. In the environment, metalloids can act as both essential nutrients and toxic pollutants. For instance, silicon is a key component of silicates, which form the backbone of many minerals and rocks, influencing soil structure and plant growth. Boron, another metalloid, is essential for plant development, particularly in cell wall formation and nutrient transport. However, when present in excessive amounts, these elements can become harmful; arsenic, for example, is a well-known contaminant that can accumulate in groundwater and pose serious health risks to humans and wildlife. In biological systems, metalloids are involved in various biochemical processes. Arsenic, despite its toxicity, has been found to be necessary in trace amounts for certain organisms. It participates in the metabolism of some bacteria and archaea, highlighting its complex role in microbial ecosystems. Antimony, another metalloid, is used in some medical treatments due to its antimicrobial properties, particularly against leishmaniasis. The biological significance of metalloids extends to their role in enzyme function and cellular regulation. For example, boron is a cofactor for certain enzymes involved in carbohydrate metabolism and hormone regulation in plants. The environmental impact of metalloids is also noteworthy. Human activities such as mining, industrial processes, and agricultural practices can lead to the release of these elements into the environment, causing pollution. Arsenic contamination in groundwater is a major public health concern in many regions, leading to chronic exposure and associated health issues like skin lesions, cardiovascular diseases, and various types of cancer. Conversely, the controlled use of metalloids in technology and medicine underscores their importance. Silicon, for instance, is a cornerstone of modern electronics due to its semiconductor properties, while germanium is used in fiber optics and solar panels. Understanding the dual nature of metalloids—both beneficial and harmful—is crucial for managing their applications effectively. This knowledge informs strategies for mitigating environmental pollution while harnessing their unique properties for technological advancements and medical treatments. By recognizing the intricate balance between the beneficial and detrimental effects of metalloids, we can better navigate their role in sustaining ecosystems and supporting human health. This nuanced understanding underscores the importance of continued research into the environmental and biological significance of these elements, ensuring that their applications are both sustainable and safe.