What Is A Pure Substance
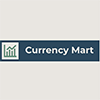
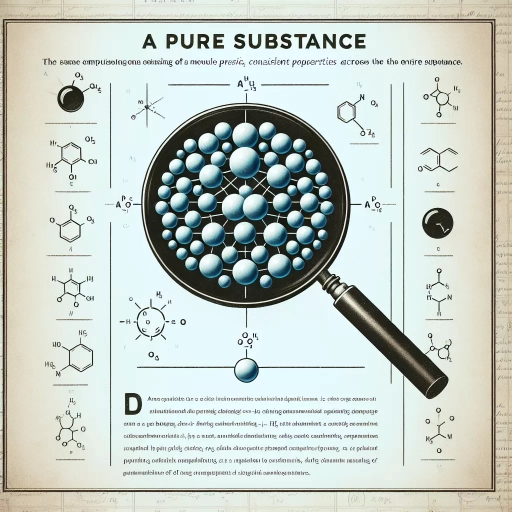
In the realm of chemistry, understanding the concept of pure substances is fundamental for both theoretical and practical applications. A pure substance is a material that consists of only one type of matter, whether it be an element or a compound, and it exhibits consistent properties throughout. This article delves into the intricacies of pure substances, exploring their definition and characteristics, the various types that exist, and the methods used for their identification and purification. By grasping these key aspects, one can better appreciate the significance of pure substances in scientific research, industrial production, and everyday life. We begin by examining the definition and characteristics of pure substances, which form the foundation for understanding their unique properties and behaviors. This foundational knowledge will then be expanded upon as we discuss the diverse types of pure substances and the sophisticated methods employed to identify and purify them. Let us start with the essential definition and characteristics that define what a pure substance truly is.
Definition and Characteristics of Pure Substances
In the realm of chemistry, understanding the nature of pure substances is fundamental to grasping the building blocks of matter. A pure substance, by definition, is a material that consists of only one type of matter, whether it be an element or a compound. This article delves into the definition and characteristics of pure substances, exploring three key aspects that define their identity and distinguish them from mixtures. First, we will examine the **Chemical Composition** of pure substances, highlighting how their molecular structure and atomic makeup set them apart. Next, we will discuss their **Physical Properties**, such as melting and boiling points, which are consistent and unique to each pure substance. Finally, we will explore how to **Distinguish Pure Substances from Mixtures**, emphasizing the importance of purity in scientific research and everyday applications. By understanding these facets, we can better appreciate the definition and characteristics of pure substances, which form the cornerstone of chemical science. This comprehensive overview will provide a clear and detailed insight into what makes a substance pure and how it is characterized.
Chemical Composition
Chemical composition is a fundamental concept in understanding the nature of pure substances. It refers to the specific proportion of elements that make up a substance, which remains constant regardless of the source or method of preparation. This consistency is a hallmark of pure substances, distinguishing them from mixtures where the composition can vary. The chemical composition of a pure substance is typically expressed through its chemical formula, which indicates the type and number of atoms of each element present in the molecule. For instance, water (H₂O) always consists of two hydrogen atoms and one oxygen atom, while carbon dioxide (CO₂) is composed of one carbon atom and two oxygen atoms. This precise ratio ensures that every sample of the substance will exhibit identical physical and chemical properties, such as melting point, boiling point, and reactivity. The significance of chemical composition extends beyond mere identification; it also influences the physical and chemical behavior of the substance. For example, the unique arrangement of atoms in a molecule determines its polarity, solubility, and reactivity with other substances. In organic chemistry, slight variations in chemical composition can lead to vastly different properties and functions, as seen in isomers—molecules with the same number and type of atoms but different structural arrangements. Understanding the chemical composition is crucial for predicting how a substance will interact with its environment and other substances, making it a cornerstone in fields such as pharmacology, materials science, and environmental chemistry. Moreover, the study of chemical composition has led to numerous advancements in science and technology. Analytical techniques like spectroscopy and chromatography allow scientists to determine the chemical composition of unknown substances with high precision. These methods have been instrumental in discovering new compounds and understanding complex biological systems. In industrial applications, knowing the exact chemical composition is essential for quality control and ensuring the reproducibility of products. For instance, in pharmaceutical manufacturing, precise control over the chemical composition of active ingredients is critical for ensuring efficacy and safety. In summary, the chemical composition of a pure substance is its defining characteristic, providing a blueprint for its properties and behavior. It is a concept that underpins much of modern chemistry and has far-reaching implications across various scientific disciplines and industrial applications. By understanding and analyzing the chemical composition, scientists can unlock the secrets of materials at the molecular level, leading to innovations that transform our world.
Physical Properties
Physical properties are intrinsic characteristics of a substance that can be observed or measured without altering its chemical identity. These properties are fundamental in defining and distinguishing pure substances from one another. For instance, **melting and boiling points** are critical physical properties that are unique to each pure substance. A pure substance will always melt and boil at the same temperature under standard conditions, making these points reliable identifiers. **Density**, another key physical property, is the mass per unit volume of a substance and is constant for a given pure substance at a specified temperature and pressure. This consistency allows for precise identification and differentiation between substances. **Solubility**, which describes how well a substance dissolves in a solvent, is also a significant physical property. Pure substances exhibit consistent solubility patterns, which can be used to predict their behavior in various solutions. **Viscosity**, or the resistance to flow, and **surface tension**, the energy at the surface of a liquid, are additional physical properties that help characterize pure substances. These properties are influenced by the intermolecular forces within the substance and can vary significantly between different pure substances. The **color** and **odor** of a substance are also physical properties that can be used to identify pure substances, although they are more subjective. Some pure substances have distinct colors or odors that make them easily recognizable. For example, iodine is known for its deep purple color, while hydrogen sulfide has a characteristic "rotten egg" smell. In addition to these properties, **magnetic behavior** and **electrical conductivity** can also be used to characterize pure substances. Certain substances are ferromagnetic, meaning they are attracted to magnets, while others may be paramagnetic or diamagnetic. Similarly, some pure substances are good conductors of electricity, while others are insulators. Understanding these physical properties is crucial in various fields such as chemistry, physics, and engineering. They provide a basis for identifying and classifying substances, which is essential in scientific research and industrial applications. For example, knowing the melting point of a substance can help in determining its purity and in designing processes for its purification or synthesis. In summary, physical properties serve as a comprehensive set of tools for defining and characterizing pure substances. Each property offers unique insights into the nature of a substance, allowing scientists to distinguish between different pure substances with precision. These properties are not only fundamental in understanding the behavior of substances but also play a vital role in practical applications across various disciplines.
Distinguishing from Mixtures
Distinguishing mixtures from pure substances is a fundamental concept in chemistry, crucial for understanding the properties and behaviors of various materials. A pure substance, such as an element or a compound, has a fixed composition and consistent properties throughout its entirety. In contrast, a mixture can be composed of two or more substances that are not chemically combined and can vary in their proportions. To distinguish between these two, several key characteristics and methods are employed. Firstly, the composition of a pure substance is uniform and unchanging, whereas mixtures can have varying compositions depending on the ratio of their components. For instance, water (H₂O) is a pure substance because it always consists of two hydrogen atoms and one oxygen atom per molecule, regardless of its source or preparation method. On the other hand, air is a mixture primarily composed of nitrogen (about 78%) and oxygen (about 21%), with trace amounts of other gases; its composition can vary slightly depending on the location and conditions. Another way to distinguish between pure substances and mixtures is through their physical properties. Pure substances have fixed melting and boiling points, which are intrinsic properties that do not change regardless of the sample size or source. For example, pure water boils at 100°C at standard atmospheric pressure. Mixtures, however, exhibit a range of boiling points because their components boil at different temperatures. This phenomenon is known as fractional distillation, where each component of the mixture is separated based on its boiling point. Chemical properties also play a significant role in distinguishing between pure substances and mixtures. Pure substances undergo chemical reactions in predictable ways due to their uniform composition. For example, when hydrogen gas reacts with oxygen gas to form water, the reaction occurs consistently according to the stoichiometry of the reactants. In contrast, mixtures may exhibit complex and variable chemical behaviors because their components can react differently under various conditions. Lastly, analytical techniques such as chromatography and spectroscopy are often used to determine whether a sample is a pure substance or a mixture. These methods allow chemists to separate and identify the individual components within a sample based on their unique properties like molecular weight, polarity, or spectral signatures. In summary, distinguishing mixtures from pure substances involves examining their composition, physical properties such as melting and boiling points, chemical behaviors during reactions, and employing analytical techniques to identify individual components. Understanding these differences is essential for accurately characterizing materials in various scientific and industrial contexts.
Types of Pure Substances
In the realm of chemistry, pure substances are fundamental building blocks that underpin our understanding of matter. These substances, devoid of impurities, exhibit consistent properties and compositions, making them crucial for scientific inquiry and practical applications. This article delves into the diverse types of pure substances, exploring their definitions, characteristics, and distinctions. We will examine **Elements**, which are the simplest forms of pure substances, consisting of a single type of atom. Next, we will discuss **Compounds**, which are formed when different elements combine in fixed ratios. Additionally, we will explore **Isotopes and Allotropes**, variations within elements that arise from differences in atomic mass or physical form. By understanding these categories, we can better appreciate the intricate world of pure substances and their unique properties. This journey through the types of pure substances will ultimately lead us to a deeper comprehension of their **Definition and Characteristics**, highlighting why they are so vital in both theoretical and applied chemistry.
Elements
Elements are the fundamental building blocks of matter and constitute one of the primary types of pure substances. A pure substance is a material that consists of only one type of matter, and elements are the simplest form of this, as they are composed of atoms that all have the same number of protons in their atomic nuclei. This uniformity in atomic structure gives elements their unique chemical properties and distinguishes them from compounds, which are formed by the chemical bonding of different elements. There are currently 118 known elements, each identified by its atomic number and symbol. These elements range from the lightest, hydrogen (H), to the heaviest, tennessine (Ts). Elements can be broadly categorized into metals, nonmetals, and metalloids based on their physical and chemical characteristics. Metals, such as iron (Fe) and copper (Cu), are typically malleable, ductile, and good conductors of electricity. Nonmetals, including carbon (C) and oxygen (O), are generally brittle, poor conductors, and often form anions when they react with metals. Metalloids, like silicon (Si) and germanium (Ge), exhibit intermediate properties between metals and nonmetals. The discovery and classification of elements have been pivotal in the development of chemistry. The periodic table, devised by Dmitri Mendeleev, organizes elements in a systematic way based on their atomic numbers and recurring chemical properties. This tool has been invaluable for predicting the behavior of elements and their compounds. Additionally, the study of elements has led to numerous technological advancements, from the use of silicon in semiconductors to the application of titanium in aerospace engineering. Understanding elements is also crucial for various scientific disciplines beyond chemistry. In biology, elements such as carbon, oxygen, and nitrogen are essential for life processes. In physics, the properties of elements are fundamental to understanding phenomena like superconductivity and magnetism. Moreover, environmental science relies on knowledge of elements to address issues such as pollution and resource management. In summary, elements are pure substances that consist of a single type of atom and are the foundational components of all matter. Their unique properties and behaviors make them indispensable in various fields of science and technology, underscoring their importance in our understanding of the natural world.
Compounds
Compounds are a fundamental type of pure substance, characterized by their composition of two or more different elements chemically bonded together in a fixed ratio. Unlike mixtures, where the proportions of the components can vary, compounds have a definite and consistent chemical formula. This uniformity is due to the specific chemical bonds that form between the atoms of the constituent elements, resulting in a new substance with properties distinct from those of its individual components. For instance, water (H₂O) is a compound composed of hydrogen and oxygen atoms in a 2:1 ratio. The chemical bonding between these atoms creates a molecule that exhibits unique properties such as a high boiling point and surface tension, which are not present in either hydrogen or oxygen alone. Similarly, sodium chloride (NaCl), commonly known as table salt, is a compound formed from sodium and chlorine atoms in a 1:1 ratio. The ionic bond between these elements gives sodium chloride its characteristic taste and solubility in water. The formation of compounds can occur through various types of chemical bonds, including ionic, covalent, and metallic bonds. Ionic compounds, like sodium chloride, are formed when electrons are transferred between atoms, leading to the formation of ions that are electrostatically attracted to each other. Covalent compounds, such as water and carbon dioxide (CO₂), result from the sharing of electron pairs between atoms. Metallic compounds involve the delocalization of electrons among metal atoms, creating a "sea" of electrons that holds the metal lattice together. The properties of compounds are often significantly different from those of their constituent elements. For example, carbon in its elemental form can exist as graphite or diamond, both of which are very hard but non-reactive. However, when carbon forms compounds like carbon dioxide or methane (CH₄), these substances exhibit entirely different physical and chemical properties. Carbon dioxide is a gas at room temperature and plays a crucial role in the Earth's climate system, while methane is a potent greenhouse gas and a primary component of natural gas. Understanding compounds is essential in various fields such as chemistry, biology, and materials science. In chemistry, the study of compounds helps in understanding chemical reactions and the synthesis of new materials. In biology, many biological molecules such as proteins, carbohydrates, and nucleic acids are complex compounds that play vital roles in living organisms. In materials science, the properties of compounds are exploited to develop new materials with specific characteristics for technological applications. In summary, compounds are pure substances that consist of two or more elements chemically bonded together in fixed ratios. Their unique properties arise from the specific types of chemical bonds that form between their constituent atoms. The study of compounds is fundamental to understanding many natural phenomena and technological advancements across various scientific disciplines.
Isotopes and Allotropes
Within the realm of pure substances, two intriguing concepts stand out: isotopes and allotropes. These terms describe different forms of elements that share certain characteristics but also exhibit distinct properties. **Isotopes** are atoms of the same chemical element that have the same number of protons in their atomic nuclei (atomic number) but differ in the number of neutrons. This variation in neutron count leads to differences in atomic mass. For instance, carbon-12, carbon-13, and carbon-14 are isotopes of carbon because they all have six protons but vary in their neutron count. Isotopes behave chemically in a nearly identical manner due to their identical electron configurations, but they can be distinguished through physical properties such as mass and radioactivity. The study of isotopes is crucial in fields like nuclear physics, geology, and medicine, where they are used for dating materials, tracing chemical pathways, and diagnosing diseases. **Allotropes**, on the other hand, refer to different physical forms of the same element. Unlike isotopes, allotropes do not differ in their atomic composition but rather in their molecular or crystal structure. A classic example is carbon, which exists as diamond and graphite. In diamond, carbon atoms are arranged in a tetrahedral structure, resulting in a hard, transparent solid. In contrast, graphite consists of layered sheets of carbon atoms arranged in a hexagonal lattice, making it soft and slippery. Another example is sulfur, which can exist as orthorhombic, monoclinic, and rhombic allotropes under different conditions. Allotropes often exhibit vastly different physical properties despite being composed of the same element, making them valuable for various applications across materials science and technology. Understanding isotopes and allotropes is essential for comprehending the diversity within pure substances. These concepts highlight the complexity and versatility of elemental forms, demonstrating how subtle changes at the atomic or molecular level can lead to significant variations in properties and uses. This knowledge is pivotal in advancing fields such as chemistry, physics, and engineering, where precise control over material properties is critical for innovation and discovery. By recognizing and leveraging these differences, scientists can develop new materials with tailored characteristics, driving progress in numerous scientific and industrial endeavors.
Identification and Purification Methods
In the realm of chemistry, the identification and purification of substances are crucial steps that underpin various scientific and industrial processes. These methods are essential for ensuring the quality and integrity of materials, whether in research laboratories, pharmaceutical manufacturing, or environmental monitoring. This article delves into three key methodologies that form the backbone of substance identification and purification: Chemical Analysis Techniques, Physical Separation Methods, and Chromatography and Distillation. Chemical Analysis Techniques involve the use of spectroscopic and chromatographic methods to determine the chemical composition of substances. Physical Separation Methods, such as filtration and centrifugation, rely on physical properties to isolate components. Chromatography and Distillation are sophisticated techniques that exploit differences in solubility, boiling points, and affinity for stationary phases to separate and purify substances. Understanding these methods is pivotal for defining and characterizing pure substances, which is the ultimate goal in many chemical endeavors. By exploring these techniques in depth, we can better appreciate the definition and characteristics of pure substances, ensuring accuracy and reliability in our scientific pursuits.
Chemical Analysis Techniques
Chemical analysis techniques are indispensable tools in the identification and purification of substances, ensuring the accuracy and reliability of scientific research and industrial processes. These methods enable scientists to determine the composition, structure, and properties of substances, which is crucial for understanding their behavior and potential applications. One of the foundational techniques is **Spectroscopy**, which involves the interaction between matter and electromagnetic radiation. Techniques such as Infrared (IR) spectroscopy, Nuclear Magnetic Resonance (NMR) spectroscopy, and Mass Spectrometry (MS) provide detailed information about molecular structure and composition. For instance, IR spectroscopy helps identify functional groups within a molecule by analyzing the absorption of infrared radiation, while NMR spectroscopy offers insights into the molecular environment of atoms through the measurement of nuclear spin states. Another pivotal technique is **Chromatography**, which separates, identifies, and quantifies each component in a mixture. High-Performance Liquid Chromatography (HPLC) and Gas Chromatography (GC) are widely used for this purpose. HPLC separates compounds based on their interaction with a stationary phase and a mobile phase, allowing for precise identification and quantification of components in complex mixtures. GC, on the other hand, separates volatile compounds based on their boiling points and affinity for the stationary phase, making it ideal for analyzing gases and volatile liquids. **Titration** is another essential method that involves the quantitative reaction between a known amount of a reagent (titrant) and the substance being analyzed (analyte). This technique is particularly useful for determining the concentration of acids, bases, and other reactive substances. Conductometric titration, for example, measures changes in electrical conductivity during the reaction to determine the endpoint. **X-ray Crystallography** is a powerful tool for determining the three-dimensional structure of molecules. By analyzing the diffraction patterns produced when X-rays interact with crystalline samples, researchers can elucidate the precise arrangement of atoms within a molecule. This information is vital for understanding the physical and chemical properties of substances. In addition to these techniques, **Thermal Analysis** methods such as Differential Scanning Calorimetry (DSC) and Thermogravimetric Analysis (TGA) provide valuable data on thermal stability and phase transitions. DSC measures heat flow associated with phase transitions or chemical reactions, while TGA quantifies mass changes due to thermal decomposition or desorption. These chemical analysis techniques collectively form a robust arsenal for identifying and purifying substances. By combining data from multiple methods, scientists can achieve a comprehensive understanding of a substance's properties and ensure its purity, which is paramount in fields ranging from pharmaceuticals to materials science. The integration of these techniques not only enhances the accuracy of scientific findings but also drives innovation by enabling the discovery and development of new materials and compounds.
Physical Separation Methods
Physical separation methods are crucial in the identification and purification of substances, allowing scientists to isolate and analyze components based on their physical properties. These methods leverage differences in characteristics such as size, density, boiling point, and solubility to separate mixtures into their constituent parts. One of the most common techniques is filtration, which involves passing a mixture through a porous material to separate solid particles from liquids or gases. Another method is sedimentation, where particles settle due to gravity, often aided by centrifugation to accelerate the process. Chromatography is a versatile technique that separates substances based on their distribution between two phases: a stationary phase and a mobile phase. This can be further divided into various types like paper chromatography, thin-layer chromatography, and column chromatography, each suited for different types of mixtures. Distillation is another key method that separates substances based on differences in boiling points. This can be applied in various forms such as simple distillation for separating two components with significantly different boiling points, fractional distillation for separating mixtures of several components with close boiling points, and steam distillation for separating substances that are immiscible with water but have high boiling points. Crystallization is another powerful technique where a solution is cooled slowly to allow the formation of crystals of the desired substance, which can then be filtered out. Magnetic separation is used for substances that are ferromagnetic or paramagnetic, allowing them to be attracted by a magnetic field while non-magnetic substances remain unaffected. Similarly, electrostatic separation exploits differences in electrical charges among particles to separate them using an electric field. These physical separation methods are not only essential in laboratory settings but also play critical roles in industrial processes such as mineral processing and pharmaceutical manufacturing. In addition to these traditional methods, modern technologies have introduced advanced techniques like ultracentrifugation and gel electrophoresis. Ultracentrifugation uses high-speed centrifuges to separate particles based on density and size at the molecular level, while gel electrophoresis separates DNA, RNA, or proteins based on their size and charge as they move through a gel matrix under an electric field. The choice of physical separation method depends on the specific properties of the substances involved and the desired level of purity. By combining multiple techniques, scientists can achieve high levels of purity necessary for detailed analysis and application in various fields. These methods are foundational in ensuring that substances are isolated in their pure form, which is essential for accurate identification and further study or use in scientific research and industrial applications.
Chromatography and Distillation
Chromatography and distillation are two fundamental techniques in the arsenal of analytical chemistry, playing pivotal roles in the identification and purification of substances. **Chromatography**, derived from the Greek words "chroma" (color) and "graphein" (to write), is a method that separates mixtures based on the differential distribution of components between two phases: a stationary phase and a mobile phase. This versatile technique can be applied in various forms, such as gas chromatography (GC), liquid chromatography (LC), and paper chromatography, each tailored to specific types of samples. For instance, GC is particularly effective for separating volatile compounds, while LC is more suited for non-volatile substances. Chromatography's ability to resolve complex mixtures into their individual components makes it an indispensable tool for both qualitative and quantitative analysis, enabling scientists to identify and quantify the constituents of a sample with high precision. **Distillation**, on the other hand, leverages the differences in boiling points of substances to separate mixtures. This ancient technique has been refined over centuries and remains a cornerstone in chemical purification. Distillation can be categorized into several types, including simple distillation, fractional distillation, and vacuum distillation. Simple distillation is used for separating substances with significantly different boiling points, while fractional distillation is employed for mixtures where the boiling points are closer together. Vacuum distillation extends the utility of this method to substances that decompose at their normal boiling points by reducing the pressure. The process involves heating the mixture to vaporize the components, which are then condensed and collected separately based on their boiling points. This method is crucial in industries such as petroleum refining and pharmaceutical manufacturing, where high purity is paramount. Both chromatography and distillation are essential for ensuring the purity of substances, which is critical in various scientific and industrial applications. In pharmaceuticals, for example, these methods help in isolating active ingredients from complex natural extracts or synthetic mixtures. In environmental science, they aid in detecting and quantifying pollutants in water and air samples. The synergy between these techniques often leads to more effective purification processes; for instance, distillation can be used as a preliminary step to separate major components before further refinement using chromatography. By combining these powerful tools, researchers and manufacturers can achieve the high levels of purity required for accurate analysis and safe use of substances. Thus, chromatography and distillation stand as pillars of modern analytical chemistry, facilitating the identification and purification of substances with unparalleled precision and reliability.