What Is Current Measured In
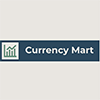
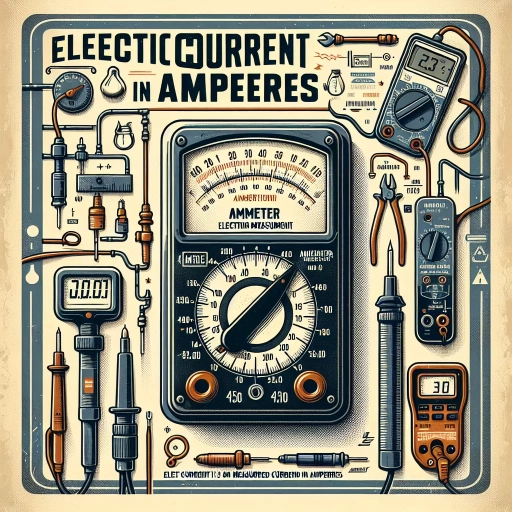
Electric current, a fundamental concept in physics and engineering, is the flow of electric charge through a conductor. Understanding what current is measured in and how it is quantified is crucial for both theoretical and practical applications. This article delves into the multifaceted nature of electric current, beginning with the basics of its measurement. We will explore **Understanding the Basics of Electric Current**, where we define key terms and principles that underpin our comprehension of current. Next, we will examine **Measurement Techniques and Tools**, highlighting the various methods and instruments used to quantify current accurately. Finally, we will discuss **Practical Applications and Importance**, illustrating how electric current impacts daily life and technological advancements. By grasping these aspects, readers will gain a comprehensive understanding of electric current and its significance. Let us start by laying the groundwork with **Understanding the Basics of Electric Current**.
Understanding the Basics of Electric Current
Electric current, a fundamental concept in physics and engineering, is the flow of electric charge through a conductor. Understanding its basics is crucial for grasping the principles behind many modern technologies. This article delves into the core aspects of electric current, starting with the **Definition and Units of Measurement**, where we explore how current is quantified and the standard units used to describe it. We then delve into the **Historical Development and Key Discoveries**, tracing the evolution of our knowledge from early experiments to groundbreaking findings that shaped our current understanding. Finally, we examine the **Basic Principles and Laws Governing Current**, including Ohm's Law and other essential theories that govern how electric current behaves. By understanding these foundational elements, readers will gain a comprehensive insight into the mechanisms and significance of electric current, ultimately enhancing their appreciation for the intricate science behind it. This journey will culminate in a deeper **Understanding of the Basics of Electric Current**.
Definition and Units of Measurement
Understanding the basics of electric current begins with a solid grasp of the fundamental concepts of measurement. At its core, electric current is the flow of electric charge, typically carried by electrons, through a conductor such as a wire. The definition of electric current is crucial: it is quantified as the rate at which electric charge flows past a given point in a circuit. This rate is measured in units that reflect the amount of charge transferred over time. The standard unit of measurement for electric current is the ampere (A), often abbreviated as amp. One ampere is defined as one coulomb per second, where a coulomb is the unit of electric charge. This relationship underscores that current is essentially a measure of how many charges are moving through a point in a circuit over a specified period. For instance, if one coulomb of charge passes through a point in one second, the current at that point is one ampere. To further illustrate this concept, consider smaller units of measurement. The milliampere (mA) and microampere (μA) are commonly used when dealing with smaller currents. One milliampere equals one-thousandth of an ampere, while one microampere is one-millionth of an ampere. These units are particularly useful in electronic circuits where currents are often in the range of milliamperes or microamperes. Understanding these units is essential for designing and analyzing electrical systems. For example, in household wiring, currents are typically measured in amperes to ensure that the wiring can safely handle the load without overheating or causing a fire. In contrast, medical devices like pacemakers operate on much smaller currents, often in the range of microamperes, to deliver precise and safe electrical impulses to the heart. In addition to the ampere, other related units help in comprehending various aspects of electric current. The watt (W), for instance, measures power and is calculated as the product of voltage and current (W = V x A). This highlights how current interacts with voltage to produce power, which is vital for understanding energy consumption and efficiency in electrical systems. In summary, grasping the definition and units of measurement for electric current is foundational to understanding its role in electrical circuits. The ampere, along with its subdivisions like milliampere and microampere, provides a precise way to quantify current flow. This precision is critical for both practical applications and theoretical analysis across diverse fields from household electronics to medical devices. By mastering these concepts, one can better appreciate the intricacies of electric current and its measurement, ultimately enhancing their ability to work with and understand electrical systems.
Historical Development and Key Discoveries
The historical development of our understanding of electric current is a rich tapestry woven from the contributions of numerous scientists and inventors over several centuries. The journey began with ancient civilizations, where observations of static electricity were first recorded. However, it wasn't until the 18th century that significant progress was made. Benjamin Franklin's groundbreaking work in the 1740s and 1750s introduced the concept of positive and negative charges, laying foundational principles for later research. The Italian physicist Alessandro Volta took a major leap forward in 1800 by inventing the first battery, known as the Voltaic pile, which provided a reliable source of electric current. In the early 19th century, André-Marie Ampère formulated Ampère's law, which relates the magnetic field around a conductor to the electric current flowing through it. This discovery was pivotal in understanding how electric currents interact with magnetic fields. Around the same time, Georg Ohm discovered Ohm's law, which describes the relationship between voltage, current, and resistance in an electric circuit. These laws collectively formed the basis for modern electrical engineering. The late 19th century saw further advancements with James Clerk Maxwell's formulation of Maxwell's equations, which unified the previously separate theories of electricity and magnetism into a single, coherent theory of electromagnetism. This work not only explained how electric currents generate magnetic fields but also predicted the existence of electromagnetic waves. In the 20th century, quantum mechanics provided deeper insights into the nature of electric current at the atomic level. The discovery of electrons by J.J. Thomson in 1897 revealed that electric current is fundamentally a flow of charged particles. Later, the development of semiconductor materials and devices by scientists like John Bardeen, Walter Brattain, and William Shockley revolutionized electronics and enabled the creation of modern electronic devices. Today, our understanding of electric current is precise and multifaceted, allowing us to harness it in myriad ways—from powering homes and industries to driving technological innovations in fields such as computing and telecommunications. The measurement of electric current itself is crucial; it is typically measured in amperes (A), with smaller units like milliamperes (mA) and microamperes (μA) used for more precise measurements. This ability to quantify and control electric current has been instrumental in transforming our world into the technologically advanced society we know today. Understanding these historical developments and key discoveries provides a solid foundation for grasping the basics of electric current and its measurement.
Basic Principles and Laws Governing Current
Understanding the basics of electric current is fundamentally rooted in several key principles and laws that govern its behavior. At the heart of these principles lies Ohm's Law, which states that the current flowing through a conductor is directly proportional to the voltage applied across it, provided the temperature remains constant. Mathematically, this is expressed as \(I = \frac{V}{R}\), where \(I\) is the current in amperes, \(V\) is the voltage in volts, and \(R\) is the resistance in ohms. This law underscores the interdependence of voltage, current, and resistance within an electrical circuit. Another crucial principle is Kirchhoff's Laws, which consist of two parts: the Current Law (KCL) and the Voltage Law (KVL). KCL states that at any node in an electrical circuit, the sum of currents entering the node is equal to the sum of currents leaving it, ensuring charge conservation. KVL, on the other hand, asserts that the sum of voltage changes around any closed loop in a circuit must be zero, reflecting the conservation of energy. The behavior of electric current is also influenced by the properties of materials, particularly their resistivity. Conductors like copper have low resistivity and allow current to flow easily, while insulators like glass or rubber have high resistivity and impede current flow. Semiconductors fall somewhere in between and are critical in modern electronics due to their ability to control current flow under different conditions. Furthermore, the direction and magnitude of electric current are governed by the concept of electromotive force (EMF). EMF is the energy per unit charge that drives electric current through a circuit. It can be generated by various sources such as batteries, generators, or solar cells. The polarity of EMF determines the direction of current flow according to the conventional flow model, where positive charges move from higher potential to lower potential. In addition to these fundamental laws and principles, understanding electric current involves recognizing its relationship with other physical quantities like power and energy. The power dissipated by a circuit element is given by Joule's Law, which states that power (\(P\)) is equal to the product of voltage (\(V\)) and current (\(I\)), or \(P = VI\). This highlights how efficiently energy is transferred within an electrical system. In summary, grasping the basics of electric current requires a solid understanding of Ohm's Law, Kirchhoff's Laws, material properties such as resistivity, electromotive force (EMF), and the relationship between current, voltage, power, and energy. These principles form the foundation upon which more complex electrical theories and applications are built, enabling us to design, analyze, and optimize electrical systems effectively. By mastering these fundamental concepts, one can delve deeper into the intricacies of electrical engineering and appreciate the underlying mechanics that govern our modern technological world.
Measurement Techniques and Tools
In the realm of electrical engineering and physics, accurate measurement of electric current is paramount for ensuring the safe and efficient operation of electrical systems. Measurement techniques and tools play a crucial role in this process, enabling professionals to monitor, analyze, and optimize current flow. This article delves into the essential aspects of current measurement, starting with an exploration of **Types of Ammeters and Their Applications**, which highlights the diverse range of instruments used to measure current and their specific uses in various fields. It then delves into **Calibration and Accuracy in Current Measurement**, emphasizing the importance of precise calibration to ensure reliable data. Finally, it discusses **Modern Technologies for Current Measurement**, showcasing the latest advancements that have revolutionized the field. By understanding these key concepts, readers will gain a comprehensive insight into the tools and methods available for measuring electric current, ultimately leading to a deeper **Understanding the Basics of Electric Current**.
Types of Ammeters and Their Applications
Ammeters, essential tools in electrical measurement, are designed to quantify the flow of electric current in various circuits. There are several types of ammeters, each tailored to specific applications and offering unique advantages. **1. Analog Ammeters:** Analog ammeters use a needle and dial to display current readings. They are simple, cost-effective, and widely used for basic measurements. These ammeters rely on the magnetic field generated by the current to deflect a pointer, providing a visual indication of the current level. Analog ammeters are commonly found in educational settings and for routine maintenance tasks due to their ease of use and straightforward operation. **2. Digital Ammeters:** Digital ammeters offer higher precision and readability compared to their analog counterparts. These devices convert the analog signal into a digital format, displaying the current reading on an LCD screen. Digital ammeters are more accurate and can handle a wider range of current measurements, making them ideal for industrial and scientific applications where precise data is crucial. **3. Clamp Ammeters:** Clamp ammeters, also known as current clamps, are versatile tools that allow for non-invasive current measurement. By clamping around a conductor without needing to break the circuit, these ammeters provide a safe and convenient way to measure current in live circuits. This feature makes them particularly useful for troubleshooting electrical issues in residential, commercial, and industrial settings. **4. Hall Effect Ammeters:** Hall effect ammeters utilize the Hall effect principle to measure current. They consist of a Hall effect sensor that detects the magnetic field produced by the current flow and converts it into an electrical signal. These ammeters are known for their high accuracy, low power consumption, and ability to measure both DC and AC currents. They are often used in automotive and aerospace applications where reliability and precision are paramount. **5. Shunt Ammeters:** Shunt ammeters involve placing a low-resistance shunt in parallel with the circuit to measure current. The voltage drop across the shunt is proportional to the current flowing through it, allowing for precise measurement. Shunt ammeters are typically used in high-current applications such as power distribution systems and heavy machinery due to their ability to handle large currents without significant voltage drop. In summary, the choice of ammeter depends on the specific requirements of the measurement task. Analog ammeters provide simplicity and cost-effectiveness for basic measurements, while digital ammeters offer precision and versatility for more complex applications. Clamp ammeters ensure safety and convenience in live circuits, Hall effect ammeters deliver high accuracy in various environments, and shunt ammeters are suited for high-current scenarios. Understanding these types of ammeters and their applications is essential for selecting the right tool for accurate current measurement in various fields.
Calibration and Accuracy in Current Measurement
**Calibration and Accuracy in Current Measurement** In the realm of electrical engineering and measurement techniques, calibration and accuracy are paramount when it comes to current measurement. Calibration is the process of configuring a measuring device to ensure its readings are as close to the true value as possible. This is crucial because even slight deviations can lead to significant errors in applications where precision is vital, such as in power distribution systems, industrial control systems, and scientific research. To achieve high accuracy in current measurement, several steps are involved. First, the measuring device—whether it's a current transformer, shunt resistor, or Hall effect sensor—must be calibrated against a standard reference. This reference is typically a highly accurate device that has been certified by a national or international standards organization. The calibration process involves comparing the readings of the device under test with those of the reference device over a range of current values. Any discrepancies are then adjusted for, ensuring that the device's output matches the true current within specified limits. Accuracy in current measurement is also influenced by environmental factors such as temperature, humidity, and electromagnetic interference (EMI). For instance, temperature changes can affect the resistance of shunt resistors or the magnetic properties of current transformers, leading to measurement errors. Therefore, it is essential to use devices that are designed to operate within specific environmental conditions and to implement shielding or other protective measures to mitigate EMI. Furthermore, the selection of appropriate measurement tools is critical. Different applications require different types of current sensors. For example, in high-frequency applications like power electronics, Hall effect sensors may be preferred due to their ability to measure DC and AC currents without physical contact. In contrast, current transformers are often used in power systems for their high accuracy and ability to measure large currents safely. Regular maintenance and periodic recalibration are also essential to maintain accuracy over time. Devices can drift out of calibration due to wear and tear or exposure to harsh conditions, so regular checks against a reference standard help ensure that measurements remain reliable. In summary, achieving high accuracy in current measurement requires meticulous calibration against certified standards, careful selection of appropriate measurement tools, consideration of environmental factors, and regular maintenance. By adhering to these best practices, engineers and technicians can ensure that their current measurements are reliable and accurate, which is critical for optimal performance and safety in a wide range of applications.
Modern Technologies for Current Measurement
Modern technologies for current measurement have revolutionized the field of electrical engineering, offering unprecedented accuracy, reliability, and versatility. At the forefront of these advancements are Hall Effect sensors, which leverage the principle of the Hall Effect to measure current without physical contact. These sensors are widely used in automotive, industrial, and consumer electronics due to their non-invasive nature, high precision, and robustness against environmental factors. Another significant technology is the Rogowski coil, a toroidal coil that encircles the conductor to measure current through the magnetic field induced by the current flow. This method is particularly advantageous in high-frequency applications and for measuring large currents with minimal insertion loss. Shunt resistors also play a crucial role in current measurement, especially in power electronics and battery management systems. These resistors provide a precise voltage drop proportional to the current flowing through them, allowing for accurate measurement with minimal impact on the circuit. The advent of high-precision shunt resistors with low temperature coefficients of resistance (TCR) has further enhanced their reliability and accuracy. Current transformers (CTs) are another essential tool, transforming high currents into lower, more manageable levels for measurement and monitoring. CTs are commonly used in power distribution systems to monitor and control current flow, ensuring grid stability and efficiency. Recent advancements in CT design have led to the development of compact, high-accuracy models suitable for a wide range of applications. In addition, modern digital multimeters and clamp meters have become indispensable tools for electricians and engineers. These devices integrate multiple measurement functions, including current measurement, into a single handheld unit. Advanced models often feature true RMS measurement capabilities, ensuring accurate readings even with distorted waveforms. The integration of Bluetooth and Wi-Fi connectivity in some models allows for real-time data logging and remote monitoring, enhancing both safety and efficiency. Furthermore, the integration of IoT (Internet of Things) technologies has enabled real-time monitoring and data analytics in current measurement. Smart sensors equipped with IoT capabilities can transmit data wirelessly to cloud platforms, where advanced algorithms can analyze trends, detect anomalies, and predict potential issues before they occur. This proactive approach significantly improves system reliability and reduces downtime. In conclusion, modern technologies for current measurement offer a diverse array of solutions tailored to various applications. From non-invasive Hall Effect sensors and Rogowski coils to precise shunt resistors and versatile current transformers, these tools collectively enhance the accuracy, reliability, and efficiency of electrical systems. As technology continues to evolve, we can expect even more sophisticated methods for measuring current, driving innovation across multiple industries.
Practical Applications and Importance
Electric current is a fundamental concept in the realm of electrical engineering, underpinning a wide array of practical applications that are crucial for modern society. This article delves into the multifaceted importance and practical uses of electric current, highlighting its pivotal role in various domains. First, we explore the **Role in Electrical Engineering and Design**, where understanding electric current is essential for the development and optimization of electrical systems. Next, we examine the **Impact on Safety and Efficiency in Electrical Systems**, emphasizing how accurate current measurement and management can prevent hazards and enhance system performance. Additionally, we discuss **Current Measurement in Various Industries**, illustrating its significance across different sectors such as manufacturing, healthcare, and energy production. By grasping these critical aspects, readers will gain a comprehensive understanding of the significance of electric current, setting the stage for a deeper exploration into **Understanding the Basics of Electric Current**. This foundational knowledge is indispensable for anyone seeking to appreciate the intricacies and applications of electrical engineering in today's world.
Role in Electrical Engineering and Design
In the realm of electrical engineering, design plays a pivotal role in ensuring the efficient, safe, and reliable operation of electrical systems. Electrical engineers are tasked with the critical responsibility of designing circuits, systems, and devices that meet specific performance criteria while adhering to stringent safety standards. This involves a deep understanding of electrical principles, including Ohm's Law, Kirchhoff's Laws, and the behavior of various components such as resistors, capacitors, and inductors. The design process begins with a thorough analysis of the problem or need, followed by the development of detailed specifications and schematics. Engineers must consider factors such as power requirements, voltage levels, current flow, and potential hazards like overcurrent or short circuits. Advanced software tools like SPICE (Simulation Program with Integrated Circuit Emphasis) and CAD (Computer-Aided Design) are often employed to simulate and optimize designs before physical prototypes are built. Effective design in electrical engineering also involves selecting appropriate materials and components that can withstand operational stresses and environmental conditions. For instance, in high-voltage applications, engineers must choose insulators and conductors that can handle extreme voltages without failing. Similarly, in consumer electronics, the design must balance performance with cost and user safety. Moreover, the role of electrical engineering design extends beyond individual components to entire systems. This includes power distribution networks, communication systems, and control systems. In power distribution, engineers design grids that efficiently transmit electricity from power plants to consumers while minimizing energy loss. In communication systems, they develop circuits that enable reliable data transmission over various media such as wires, fiber optics, or wireless channels. The importance of meticulous design cannot be overstated. Poorly designed electrical systems can lead to inefficiencies, malfunctions, or even catastrophic failures. For example, a faulty circuit design could result in overheating or electrical fires, posing significant risks to human life and property. Conversely, well-designed systems enhance reliability, reduce maintenance costs, and improve overall system performance. In practical applications, the impact of electrical engineering design is evident across various industries. In healthcare, medical devices such as defibrillators and MRI machines rely on precise electrical designs to function accurately and safely. In transportation, electric vehicles depend on sophisticated electrical systems for propulsion and control. Even in everyday life, the reliability of household appliances and lighting systems is a direct result of sound electrical engineering design. In conclusion, the role of design in electrical engineering is multifaceted and indispensable. It requires a blend of theoretical knowledge, practical skills, and meticulous attention to detail to ensure that electrical systems operate efficiently, safely, and reliably. The importance of this role underscores the critical contribution that electrical engineers make to modern society by enabling the development of complex technologies that drive innovation and improve quality of life.
Impact on Safety and Efficiency in Electrical Systems
The impact of current measurement on safety and efficiency in electrical systems is multifaceted and critical. Accurate current measurement ensures that electrical systems operate within safe parameters, preventing overheating, short circuits, and potential fires. For instance, in industrial settings, monitoring current levels helps in detecting anomalies such as overloads or malfunctions in machinery, allowing for prompt intervention to avoid catastrophic failures. This proactive approach not only enhances safety but also reduces downtime and maintenance costs, thereby improving overall efficiency. In residential contexts, smart home devices and energy management systems rely on precise current measurements to optimize energy consumption. By tracking real-time current usage, homeowners can identify areas of inefficiency and make informed decisions about energy-saving measures. This not only reduces their utility bills but also contributes to a more sustainable environment by minimizing energy waste. Furthermore, in the realm of renewable energy, accurate current measurement is essential for the efficient operation of solar and wind power systems. Inverters and charge controllers in these systems depend on precise current data to optimize power conversion and storage. This ensures that the maximum amount of renewable energy is harnessed and utilized effectively, enhancing the reliability and efficiency of these systems. From a regulatory standpoint, current measurement plays a crucial role in compliance with safety standards and regulations. Electrical inspectors and engineers use current measurements to verify that installations meet safety codes, such as those set by the National Electric Code (NEC) in the United States. Non-compliance can lead to severe penalties and risks to public safety, making accurate current measurement a legal necessity. In addition, advancements in technology have made current measurement more accessible and sophisticated. Modern digital multimeters and current clamps offer high precision and ease of use, enabling both professionals and DIY enthusiasts to measure current safely and accurately. These tools are indispensable for troubleshooting electrical issues, diagnosing faults, and performing routine maintenance tasks efficiently. In summary, the impact of current measurement on safety and efficiency in electrical systems is profound. It underpins the safe operation of industrial machinery, optimizes energy consumption in residential settings, enhances the performance of renewable energy systems, ensures regulatory compliance, and facilitates efficient troubleshooting and maintenance. As technology continues to evolve, the importance of accurate current measurement will only grow, making it an indispensable aspect of modern electrical engineering and management.
Current Measurement in Various Industries
Current measurement is a critical component across various industries, where precise and reliable data are essential for operational efficiency, safety, and innovation. In the **power generation and distribution sector**, accurate current measurements are vital for monitoring grid performance, detecting anomalies, and ensuring the reliable supply of electricity. Advanced current transformers and sensors enable real-time monitoring, helping utilities manage load balancing and predict potential outages. This data also supports the integration of renewable energy sources into the grid, facilitating a smoother transition to sustainable power. In **industrial automation**, current measurement plays a pivotal role in controlling and optimizing machinery performance. By monitoring the electrical current drawn by motors and other equipment, manufacturers can identify inefficiencies, predict maintenance needs, and prevent costly downtime. This proactive approach enhances overall plant productivity and reduces energy consumption. Additionally, current measurement is integral to the development of smart factories, where data-driven insights drive continuous improvement. The **healthcare industry** relies on precise current measurements in medical devices such as MRI machines, defibrillators, and patient monitoring systems. These devices require stable and controlled electrical currents to ensure accurate diagnoses and safe treatments. For instance, in MRI technology, precise current control is necessary to generate the strong magnetic fields that produce detailed images of the body. In **aerospace and defense**, current measurement is crucial for the operation of sophisticated electronic systems onboard aircraft and spacecraft. These systems demand high reliability and accuracy to ensure safe flight operations and mission success. Current sensors help in monitoring power distribution, detecting faults, and optimizing system performance under extreme conditions. The **automotive sector** also benefits significantly from current measurement. Modern vehicles rely on complex electrical systems, including those for engine control, safety features, and infotainment. Accurate current measurements help in diagnosing issues, optimizing battery performance, and enhancing overall vehicle efficiency. This is particularly important in electric vehicles (EVs), where precise current control is essential for battery health and range optimization. Furthermore, **research and development** in fields like physics and materials science often involve precise current measurements. Scientists use sensitive current detectors to study phenomena at the quantum level or to analyze the properties of new materials. These measurements are fundamental to advancing our understanding of the physical world and driving technological innovation. In summary, current measurement is a cornerstone of various industries, enabling better performance, safety, and innovation. Its importance spans from ensuring reliable power supply to driving advancements in healthcare, aerospace, automotive technologies, and scientific research. As technology continues to evolve, the role of accurate current measurement will only become more critical.