What Is Density In Science
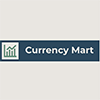
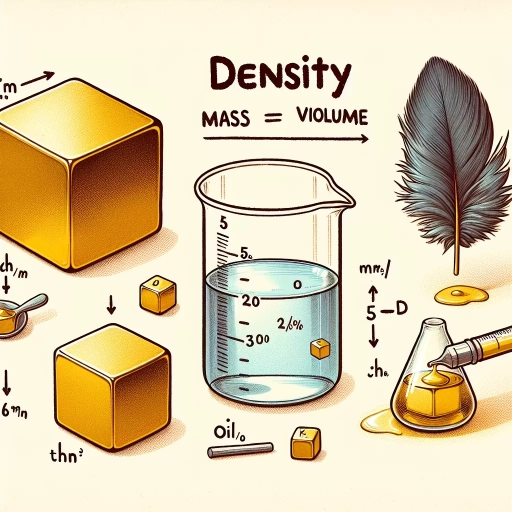
Density, a fundamental concept in science, plays a crucial role in understanding the physical properties of substances. It is a measure that quantifies the amount of mass contained in a given volume of a substance. This article delves into the multifaceted nature of density, starting with its definition and conceptual framework. We will explore how density is defined and the underlying principles that govern its calculation. Following this, we will discuss various methods for measuring density, highlighting both traditional and modern techniques. Finally, we will examine the applications and importance of density in scientific fields, illustrating its significance in everyday life and advanced research. By grasping these aspects, readers will gain a comprehensive understanding of density's role in science. Let us begin by delving into the definition and concept of density, which forms the foundation of our exploration.
Definition and Concept of Density
The concept of density is a fundamental principle in physics that describes the amount of mass contained in a given volume of a substance. Understanding density is crucial for various scientific and engineering applications, as it helps in predicting the behavior of materials under different conditions. This article delves into the definition and concept of density, exploring three key aspects: the relationship between mass and volume, the units of measurement for density, and how density varies across different states of matter. By examining the intricate relationship between mass and volume, we can grasp how these two quantities are inversely related to density. This foundational understanding sets the stage for discussing the specific units used to measure density, which are essential for precise calculations. Finally, we will explore how density changes as a substance transitions between solid, liquid, and gas states. To begin, let's first explore the critical relationship between mass and volume, as this forms the basis of density calculations.
Mass and Volume Relationship
The relationship between mass and volume is a fundamental concept in understanding density. Density, defined as mass per unit volume, is a measure of how much matter is packed into a given space. To grasp this relationship, it's essential to distinguish between mass and volume. Mass is a measure of the amount of matter in an object and is typically measured in units such as grams or kilograms. Volume, on the other hand, is the amount of space that the object occupies and is usually measured in cubic units like cubic meters or cubic centimeters. When considering the mass and volume relationship, it becomes clear that density is the connecting link. For instance, if two objects have the same volume but different masses, the one with the higher mass will have a higher density. Conversely, if two objects have the same mass but different volumes, the one with the smaller volume will have a higher density. This is because density (ρ) is calculated using the formula: ρ = mass / volume. Therefore, any change in either mass or volume directly affects the density of an object. Understanding this relationship is crucial in various scientific and practical applications. For example, in chemistry, knowing the density of substances helps in identifying them and predicting their behavior under different conditions. In engineering, density plays a critical role in designing structures and materials that need to meet specific weight and volume requirements. Even in everyday life, the mass and volume relationship influences how we perceive and interact with objects; for instance, a dense object like a metal brick feels heavier than a less dense object like a Styrofoam block of the same size. Moreover, the mass and volume relationship highlights the importance of units and dimensions. Ensuring that measurements are taken in consistent units is vital for accurate calculations of density. This precision is particularly important in fields such as physics and materials science where small variations can significantly impact outcomes. In summary, the interplay between mass and volume forms the backbone of understanding density. By recognizing how these two quantities interact, scientists and engineers can better comprehend and manipulate the properties of materials, leading to advancements in various fields. This foundational knowledge not only aids in theoretical understanding but also has practical implications that shape our daily experiences and technological innovations.
Units of Measurement for Density
When discussing the concept of density in science, it is crucial to understand the various units of measurement that are used to express this physical property. Density is defined as the mass per unit volume of a substance, and it is typically measured in units that reflect this relationship. The most common unit of density in the International System of Units (SI) is kilograms per cubic meter (kg/m³). This unit is widely used in scientific and engineering applications due to its precision and ease of calculation. In addition to kg/m³, other units are also employed depending on the context and the specific field of study. For instance, grams per cubic centimeter (g/cm³) is another frequently used unit, particularly in chemistry and biology. This unit is convenient for measuring the density of substances that are typically handled in smaller quantities. Another unit, pounds per cubic foot (lb/ft³), is commonly used in engineering and construction in the United States, reflecting the imperial system of measurement. The choice of unit often depends on the scale and nature of the measurement. For example, when dealing with very dense materials like metals, g/cm³ might be more practical, while for larger volumes such as those encountered in civil engineering projects, kg/m³ or lb/ft³ might be more appropriate. Understanding these different units is essential for accurate calculations and comparisons across various scientific disciplines. Moreover, converting between these units is straightforward with basic arithmetic operations. For instance, to convert from g/cm³ to kg/m³, one simply multiplies by 1000 since there are 1000 grams in a kilogram and 1000 cubic centimeters in a cubic meter. Similarly, converting from lb/ft³ to kg/m³ involves multiplying by 0.01601846 due to the conversion factors between pounds and kilograms and between cubic feet and cubic meters. In summary, the units of measurement for density—such as kg/m³, g/cm³, and lb/ft³—provide a standardized way to express this fundamental physical property. Each unit has its own set of applications and advantages, making it important for scientists and engineers to be familiar with them to ensure accurate and meaningful measurements. This understanding is pivotal in various fields where density plays a critical role, such as in materials science, fluid dynamics, and geology. By mastering these units, professionals can better analyze and interpret data related to density, leading to more precise and reliable conclusions.
Density in Different States of Matter
Density, a fundamental concept in physics, varies significantly across different states of matter—solid, liquid, and gas. In solids, the molecules are tightly packed and have a fixed position in space, resulting in a higher density compared to other states. This close packing is due to strong intermolecular forces that hold the molecules together, making solids generally denser than liquids and gases. For example, the density of iron in its solid state is approximately 7.9 grams per cubic centimeter (g/cm³), which is much higher than its liquid state. In liquids, the molecules are closer together but are free to move past one another. This mobility reduces the overall density compared to solids because the molecules are not as tightly packed. However, liquids still maintain a relatively high density due to the remaining intermolecular forces. Water, for instance, has a density of about 1 g/cm³ at room temperature, which is less than its solid form (ice) but significantly higher than its gaseous state. Gases exhibit the lowest density among the three states of matter. Here, the molecules are widely spaced and are free to move in any direction. The intermolecular forces are much weaker, leading to a much lower density. For example, the density of air at room temperature and atmospheric pressure is approximately 0.0012 g/cm³, which is several orders of magnitude lower than that of solids and liquids. Understanding these variations in density across different states of matter is crucial for various scientific and engineering applications. It helps in predicting the behavior of materials under different conditions, such as how they will expand or contract with temperature changes or how they will interact with other substances. Additionally, knowledge of density is essential in fields like chemistry, where it aids in identifying substances and determining their purity, and in physics, where it plays a key role in understanding phenomena like buoyancy and fluid dynamics. In summary, the density of a substance changes significantly as it transitions from one state of matter to another. Solids are generally the densest due to their tightly packed molecules, followed by liquids with their somewhat looser molecular arrangement, and finally gases with their widely spaced molecules. This understanding of density across different states is fundamental to a wide range of scientific inquiries and practical applications.
Methods for Measuring Density
Measuring density is a fundamental task in various scientific disciplines, including physics, chemistry, and engineering. There are several methods to determine the density of substances, each with its own set of advantages and applications. This article will delve into three primary methods: Archimedes' Principle and the Displacement Method, the Pycnometer Method for solids and liquids, and the use of Density Gradient Columns and Hydrometers. Archimedes' Principle, which involves measuring the buoyancy force exerted on an object when submerged in a fluid, is a classic and widely used technique. The Pycnometer Method, on the other hand, relies on the precise measurement of volume changes to calculate density. Density Gradient Columns and Hydrometers offer a more visual and straightforward approach, particularly useful for quick assessments. By understanding these methods, researchers and scientists can accurately determine the density of various materials, which is crucial for numerous applications. Let's begin by exploring Archimedes' Principle and the Displacement Method, which form the foundation of density measurement techniques.
Archimedes' Principle and Displacement Method
Archimedes' Principle and the displacement method are fundamental concepts in measuring density, a crucial property in various scientific disciplines. Archimedes' Principle states that the buoyancy force on an object submerged in a fluid is equal to the weight of the fluid displaced by the object. This principle was famously discovered by Archimedes while he was taking a bath, leading to his celebrated "Eureka!" moment. The principle is mathematically expressed as \( F_b = \rho V g \), where \( F_b \) is the buoyant force, \( \rho \) is the density of the fluid, \( V \) is the volume of the fluid displaced, and \( g \) is the acceleration due to gravity. The displacement method leverages Archimedes' Principle to measure the density of an object. Here’s how it works: an object is submerged in a fluid (usually water) in a container, causing the fluid level to rise. The volume of the fluid displaced is directly proportional to the volume of the object. By measuring this displaced volume and knowing the mass of the object, one can calculate its density using the formula \( \text{Density} = \frac{\text{Mass}}{\text{Volume}} \). This method is particularly useful for irregularly shaped objects or those whose volume cannot be easily measured directly. For instance, if you want to determine the density of a rock, you would first measure its mass using a balance. Then, you would submerge it in water and measure the volume of water displaced. The difference in water levels before and after submersion gives you the volume of the rock. With these two pieces of information—mass and volume—you can calculate the density of the rock accurately. This technique is not only limited to solids but can also be applied to liquids and gases under specific conditions. For example, in hydrostatic weighing, an object is weighed both in air and when submerged in water. The difference in weights gives the buoyant force, which can then be used to calculate the volume and hence the density of the object. In summary, Archimedes' Principle and the displacement method provide a reliable and straightforward approach to measuring density. By understanding how much fluid an object displaces when submerged, scientists can accurately determine its volume and subsequently its density, making these concepts indispensable tools in various fields such as physics, chemistry, and engineering.
Pycnometer Method for Solids and Liquids
The Pycnometer Method is a precise and widely used technique for measuring the density of both solids and liquids. This method involves using a pycnometer, which is essentially a container with a precisely known volume. For solids, the process begins by filling the pycnometer with a known volume of water or another liquid of known density. The weight of the pycnometer filled with this liquid is then measured. Next, a sample of the solid is added to the pycnometer, displacing some of the liquid. The weight of the pycnometer with the solid and remaining liquid is measured again. By calculating the difference in weights and knowing the volume of the displaced liquid, one can determine the volume of the solid. The density of the solid is then calculated by dividing its mass by its volume. For liquids, the procedure is slightly different but equally straightforward. The pycnometer is first filled with the liquid to be measured, and its weight is recorded. Then, a known mass of a solid (often a metal sphere or pellet) is added to the pycnometer, causing some of the liquid to spill over. The weight of the pycnometer with the liquid and solid is measured again. By subtracting the weight of the solid from this total weight and knowing the volume of the pycnometer, one can calculate the density of the liquid. The Pycnometer Method offers several advantages, including high accuracy and the ability to measure densities over a wide range of temperatures and pressures. It is particularly useful for substances that are difficult to measure using other methods due to their texture or reactivity. However, it requires careful calibration and handling to ensure precise results. Overall, the Pycnometer Method is an essential tool in various scientific fields, such as chemistry, physics, and engineering, where accurate density measurements are crucial for understanding material properties and behaviors.
Density Gradient Columns and Hydrometers
Density Gradient Columns and hydrometers are two distinct yet complementary tools used in the measurement of density, a fundamental property in various scientific disciplines. **Density Gradient Columns**: A density gradient column is a laboratory tool that consists of a vertical column filled with layers of liquids of different densities. These layers are typically created by mixing a solvent with a dense substance, such as sucrose or cesium chloride, to achieve a range of densities. When an object is placed in the column, it will float or sink until it reaches the layer that matches its own density. This method is particularly useful for determining the density of small objects or samples where other methods might be impractical. For instance, in biology, density gradient centrifugation is used to separate cellular components based on their densities. **Hydrometers**: A hydrometer is a simpler, more straightforward device designed to measure the density of liquids. It consists of a weighted float attached to a graduated stem. When the hydrometer is placed in a liquid, it floats to a depth that corresponds to the liquid's density, which can then be read off the stem. Hydrometers are calibrated for specific temperature ranges and are commonly used in industries such as brewing, winemaking, and chemistry labs. They offer quick and accurate measurements but are limited to liquids and require careful handling to avoid errors. **Comparison and Applications**: While both tools measure density, they serve different purposes. Density gradient columns are versatile and can be used for both liquids and solids, making them invaluable in research settings where precise separation and analysis are required. Hydrometers, on the other hand, are specialized for liquid density measurements and are preferred for their ease of use and rapid results. In scientific research, density gradient columns are crucial in fields like biochemistry and materials science, whereas hydrometers find extensive use in quality control and process monitoring in various industries. In summary, density gradient columns and hydrometers are essential tools for measuring density, each with its unique advantages and applications. Understanding how these tools work and when to use them is critical for accurate and efficient density measurements across various scientific and industrial contexts.
Applications and Importance of Density in Science
Density, a fundamental physical property, plays a crucial role across various scientific disciplines, making it an indispensable concept in understanding and applying scientific principles. In physics and engineering, density is essential for designing structures, predicting the behavior of materials under different conditions, and optimizing performance. For instance, engineers rely on density to calculate the weight and stability of buildings, bridges, and other infrastructure. In chemistry and materials science, density helps in identifying substances, determining their purity, and understanding their chemical properties. This is particularly important in the development of new materials with specific characteristics. Additionally, in environmental and biological sciences, density is used to study the properties of water and soil, which are vital for understanding ecosystems and managing natural resources effectively. By grasping the concept of density in these diverse fields, scientists can make accurate predictions, develop innovative solutions, and advance our understanding of the world around us. This article will delve into the applications and importance of density, starting with its critical role in physics and engineering.
Density in Physics and Engineering
Density, a fundamental concept in physics and engineering, plays a crucial role in various scientific and practical applications. It is defined as the mass per unit volume of a substance, typically expressed in units such as kilograms per cubic meter (kg/m³) or grams per cubic centimeter (g/cm³). Understanding density is essential for engineers and scientists because it influences the behavior and properties of materials under different conditions. In engineering, density is critical for designing structures and systems. For instance, architects must consider the density of building materials to ensure structural integrity and stability. High-density materials like steel and concrete are often used in construction due to their strength and durability. Conversely, low-density materials such as foam and wood are used where weight reduction is necessary, such as in aircraft and automotive design. The buoyancy principle, which relies on density differences between objects and fluids, is also pivotal in naval engineering for designing ships and submarines. In physics, density is a key factor in understanding fluid dynamics and thermodynamics. The concept of density helps explain phenomena like buoyancy and flotation. According to Archimedes' Principle, an object will float if it is less dense than the fluid it is immersed in, which is why ships made of relatively low-density materials can stay afloat despite their massive size. Additionally, density variations with temperature are crucial in meteorology and oceanography, influencing weather patterns and ocean currents. Density also has significant implications in materials science. Researchers use density measurements to identify and characterize different materials, as each substance has a unique density. This property is particularly useful in forensic science for analyzing evidence and in geology for identifying minerals and rocks. Furthermore, understanding the density of materials is vital in the development of new technologies, such as advanced composites and nanomaterials, which often require precise control over material properties. In medical science, density measurements are used in diagnostic imaging techniques like computed tomography (CT) scans. These scans rely on the differences in density between various tissues to produce detailed images of the body's internal structures. Similarly, in nuclear medicine, the density of radioactive isotopes is crucial for therapeutic applications and diagnostic procedures. The importance of density extends to environmental science as well. For example, oceanographers study changes in seawater density to understand ocean circulation patterns and climate dynamics. These changes can have profound effects on global weather patterns and marine ecosystems. In summary, density is a fundamental property that underpins numerous scientific and engineering applications. Its importance spans from structural design and fluid dynamics to materials science, medical diagnostics, and environmental studies. Understanding density allows scientists and engineers to predict and manipulate the behavior of materials, leading to innovations and advancements across various fields.
Density in Chemistry and Materials Science
Density, a fundamental property in chemistry and materials science, is defined as the mass per unit volume of a substance. It is a critical parameter that influences various physical and chemical behaviors of materials. In chemistry, density helps in identifying substances and determining their purity. For instance, the density of a solution can indicate the concentration of solutes, which is crucial in chemical reactions and synthesis processes. In materials science, density plays a pivotal role in understanding the structural properties of materials. It affects the mechanical strength, thermal conductivity, and electrical conductivity of materials, making it essential for material selection in engineering applications. For example, high-density materials like metals are often used in construction due to their strength and durability, while low-density materials such as foams are used for insulation. The measurement of density is also vital in quality control and research. Techniques such as pycnometry and hydrostatic weighing are commonly used to determine the density of solids and liquids accurately. In addition, density is a key factor in fluid dynamics and buoyancy calculations, which are important in fields like oceanography and aerospace engineering. The concept of density also extends to nanomaterials and composites, where it can significantly impact their performance and functionality. For instance, the density of nanoparticles can influence their interaction with biological systems, making it a critical consideration in biomedical applications. Moreover, understanding density is essential for environmental science. It helps in studying ocean currents and climate patterns by analyzing the density variations of seawater. In geology, density measurements are used to identify different rock types and understand Earth's internal structure. The importance of density is further highlighted in industrial processes such as separation techniques, where differences in density are exploited to separate components of mixtures. In summary, density is a multifaceted property that underpins numerous scientific and engineering disciplines. Its accurate measurement and understanding are indispensable for a wide range of applications, from chemical synthesis and material selection to environmental studies and industrial processes. The significance of density underscores its role as a fundamental descriptor of material properties, making it an essential concept in both chemistry and materials science.
Density in Environmental and Biological Sciences
Density plays a crucial role in both environmental and biological sciences, influencing various phenomena and processes that shape our understanding of the natural world. In environmental science, density is essential for understanding ocean currents and climate dynamics. For instance, the difference in density between warm and cold water drives global ocean circulation patterns, which in turn affect regional climates and weather systems. This thermohaline circulation is driven by changes in water density due to variations in temperature and salinity, impacting global heat distribution and marine ecosystems. Additionally, density affects the behavior of pollutants in water bodies; denser pollutants tend to sink, while less dense ones float, influencing their distribution and impact on aquatic life. In biological sciences, density is vital for understanding organismal physiology and ecology. For example, the density of bones in vertebrates is a key factor in determining their overall body density, which affects their buoyancy in water. This is particularly important for aquatic animals like fish and marine mammals, where body density must be carefully regulated to maintain efficient swimming and diving capabilities. Furthermore, the density of plant tissues influences their ability to float or sink in water, impacting seed dispersal mechanisms and plant distribution in aquatic environments. In human health, bone density is a critical indicator of osteoporosis risk, with lower bone density increasing the likelihood of fractures. Moreover, density is crucial in the study of ecosystems and biodiversity. The density of populations within an ecosystem can influence competition for resources, predator-prey dynamics, and overall ecosystem stability. For instance, high population densities can lead to increased competition for food and habitat, potentially driving evolutionary adaptations or even population declines. In conservation biology, understanding population density helps in managing wildlife populations and designing effective conservation strategies. In agricultural science, soil density affects water infiltration rates and root growth, impacting plant health and productivity. Compacted soils with higher densities can impede root penetration and reduce water availability to plants, while optimal soil densities support healthy root development and efficient water use. This understanding is essential for sustainable agriculture practices aimed at maximizing crop yields while minimizing environmental impact. Overall, density is a fundamental property that underpins many critical processes in environmental and biological sciences. Its applications range from understanding global climate patterns to managing ecosystems and ensuring human health, highlighting its importance as a key metric in scientific inquiry and practical application.