What Is Particle Theory Of Matter
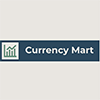
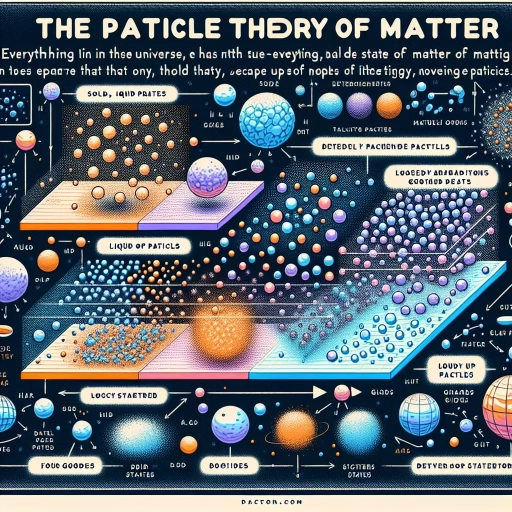
Introduction to Particle Theory of Matter
Particle theory, also known as the kinetic theory of matter, is a fundamental scientific principle that underscores the concept that all matter is made up of minute particles. This introductory exposition delves into the engrossing world of the particle theory, elucidating its historical development, key principles and assumptions, and objective prominence in modern science. Commencing with its historical progression, this article will trace the evolutionary arc of this fascinating theory, demystifying the evolution from philosophical ideas to data-backed scientific tenets over time. Moving forward, the article will then delve into the intricate details of the key principles and assumptions of particle theory, articulating how tiny, invisible, and incessantly moving particles constitute the whole universe. Finally, we will investigate the pivotal role it plays in advancing modern science by laying the foundation for various fields such as quantum mechanics, thermodynamics, and environmental science. As we embark on this explorative journey, let's first transport ourselves to the historic juncture where the rudimentary form of particle theory was born.
Historical Development of Particle Theory
In the grand scheme of scientific knowledge, the historical development of particle theory is truly an exhilarating journey that echoes the ceaseless curiosity and relentless pursuit of truth by generations of scientists. The underlying concept of particle theory, or the idea that matter is composed of minuscule, discrete particles, has ancient roots; the philosophy dates back to the 5th century BC, where Greek philosophers Leucippus and his pupil Democritus first proposed ideas on atomism. They suggested that the world is composed of empty space and an infinite number of tiny particles, 'atomos,' which are indivisible, thus laying the groundwork for modern particle theory. Throughout late antiquity to the middle ages, these pioneering ideas fell into obscurity until the 17th-century resurgence through the works of significant scientists like Gassendi and Newton, who drew upon the Greek's original concept and provided a physical interpretation of atomism in their inquiries into gases and light. However, it wasn't until the nineteenth century that the concept of atoms as the building blocks of matter decisively entered mainstream scientific thought, thanks primarily to John Dalton's atomic theory. Dalton's work forged a pivotal link between chemical reactions and the rearrangement of these particles, further solidifying the validity of particle theory. The dawn of the 20th century saw a dramatic intensification of research, leading to various breakthroughs that have since reshaped our understanding of matter fundamentally. JJ Thomson's discovery of the electron in 1897 led to the "plum pudding" atomic model, representing atoms as a mix of positive and negative particles. Subsequently, Rutherford's gold foil experiments heralded a major shift in perspective, replacing Thomson's model with one that focused on a tiny nucleus surrounded by orbiting electrons. Niels Bohr soon improved this by incorporating quantum theory and elucidating the specific energy levels in which the electrons reside. Later in the mid-20th century, the introduction of quantum mechanics and the Standard Model, a theoretical framework to explain the workings of the subatomic world, further propelled the particle theory. The discovery of quarks, leptons, and bosons has not only unraveled the bewildering complexity of atomic structure but also explained why matter exists in the first place. Presently, the CERN's Large Hadron Collider's experiments are leading us into a new era of particle physics where even more exciting discoveries await. From the ancient Greeks' basic musings to the intricate world of quarks and leptons, the historical development of particle theory draws a fascinating roadmap of human curiosity and our quest to unfold the mysteries of the universe.
Key Principles and Assumptions
Particle Theory of Matter draws its foundation from several key principles and assumptions that bolster our understanding of matter's fundamental nature. These principles are not mere conjectures but are grounded in empirical evidence and rigorous scientific exploration. Firstly, the theory posits that matter is composed of tiny, discrete particles called atoms and molecules. These particles are so small that they’re typically invisible to the naked eye, and they are in constant motion. This assumption helps explain various phenomena such as diffusion, where substances mix due to the unceasing motion of their particles. Secondly, Particle Theory assumes that all particles of a single substance are identical in mass, size, and chemical properties. For example, all water molecules possess the same molecular structure, regardless of their source, be it a glacier in the Arctic or a river in the Amazon. However, particles between different substances vary considerably. Additionally, there are intermolecular spaces between particles. The magnitude of these spaces can significantly influence a substance's state, either solid, liquid, or gas. For instance, in solids, particles are tightly packed with minimal spaces, resulting in definite volume and shape. In contrast, gases have particles more loosely arranged and far apart, leading to an indefinite shape and volume. The theory further propounds that particles are in ceaseless motion, and this movement depends substantially on the temperature of the substance. As temperature increases, particles move faster, and conversely, at lower temperatures, particle movements slow down. This principle explains why substances change their state upon heating or cooling. Lastly, the theory assumes that particles interact and attract each other, influencing many physical and chemical properties of substances. For example, the strength of these attractions can directly impact a substance's boiling or melting point. Understanding these principles is integral to the Particle Theory of Matter. These fundamental assumptions enable us to decipher the complexities of the physical world around us, from the phase changes we witness every day to more intricate chemical reactions. However, like all scientific theories, the Particle Theory of Matter is continually reviewed and adapted to incorporate new evidence and understandings, ensuring it remains a robust and relevant explanation of the physical universe's dynamics.
Importance in Modern Science
Understanding the Particle Theory of Matter is integral to Modern Science, especially in the fields of Physics and Chemistry. Deep comprehension of this fundamental theory forms the groundwork for further study into complex fields such as Quantum Mechanics, Cosmology and Molecular Biology. It has the ability to explain properties of solids, liquids and gases in detail. The importance of Particle Theory is magnified in Modern Science due to its potential to help address a multitude of esoteric scientific quandaries. Particle Theory is the cornerstone of Atomic Theory which postulates that matter is composed of discrete units referred to as atoms. This theory forms a solid basis for understanding chemical reactions where atoms recombine in different ways to form new substances. Furthermore, the Particle Theory extends into understanding how heat transfer occurs. This knowledge is vital in daily life applications such as design and operation of modern technology like refrigerators and air conditioners. Additionally, Particle Theory of Matter is absolutely essential in understanding the state-changing processes of matter. Understanding how particles behave and interact during these changes is pivotal to multiple industries. For instance, plastic manufacturers harness the knowledge of these behaviours to adequately manipulate polymers into new forms. In the grand scheme of Modern Science, Particle Theory acts as a gateway to understanding the nature of the universe itself. Understanding the properties of particles allows scientists to hypothesize and test theories about the universe's composition. This curiosity led to the discovery of the Higgs Boson, commonly known as the ‘God Particle’, a key component to our understanding of why physical matter has mass. In the medical field, understanding of the Particle Theory of Matter forms the basis for developing microscopic devices known as nanobots. These incredibly tiny devices are intended to navigate the human body, carrying out various tasks from targeted drug delivery to performing microsurgery. Moreover, the knowledge of Particle Theory contributes to the research and development of environmentally friendly and sustainable resources, such as recyclable materials and renewable energy. In conclusion, the importance of the Particle Theory of Matter in Modern Science is profound. It forms the foundation for various scientific studies, technological applications, and industrial processes. It helps us examine and explain the world around us, from the natural phenomena to the intricacy of the humankind’s most advanced technological creations. As modern science continues to unravel and explain the mysteries of our universe, the Particle Theory of Matter remains a valuable tool in our scientific undertaking.
Components and Structure According to Particle Theory
Diving into the universe of atoms, the fundamental structures that constitute everything around us, opens up a whole new frontier of knowledge. Bringing clarity to the intricacies of Particle Theory is a task that involves understanding three significant concepts: Atoms and Molecules, Subatomic Particles: Protons, Neutrons, and Electrons, and Intermolecular Forces and Bonds. The idea of Atoms and Molecules refines the basic building blocks of all entities, detailing how different atomic combinations create diverse molecular structures that contribute to the unique properties of substances. Moving further, we delve into the heart of atoms, exploring the Subatomic Particles: Protons, Neutrons, and Electrons, which orchestrate the overall behavior and identity of atoms. Lastly, conceptualising Intermolecular Forces and Bonds helps in comprehending the interactions between molecules, influencing the physical characteristics of substances, from their phase of matter to their solubility. Embarking first on the journey into the realm of Atoms and Molecules, we shall observe the structure, composition, and the fundamental role they play in the material world.
Atoms and Molecules
The Particle Theory of Matter hypothesizes that all matter is made up of tiny, indestructible particles, uniquely identified as atoms and molecules. These elements, constantly in motion, provide the foundational structure of existence, and their complex interrelationships contribute to the manifold diversity of the material world. Atoms, which retain specific features that diversify them into more than one hundred known types or 'elements', are the most elementary particles according to classical physics. Constructed by sub-atomic particles, atoms comprise protons and neutrons housed in a dense nucleus, surrounded by a cloud of rapidly moving electrons. Every atom's identity hinges on its proton count, usually denoted as atomic number; this distinguishes one element from another. Having the same number of electrons and protons, the atom remains electrically neutral, but change to this delicate balance leads to ion formation, a crucial process in many chemical reactions. Atoms can link together to form molecules, complex structures that engender the vast diversity of matter on the planet. The number of atoms and the techniques they adopt to combine determine the molecule's identity. Molecules, compared with singular atoms, have qualities that seem unpredictable and unique due to these interactions. A great illustration of this is water - a molecule composed of two hydrogen atoms and one oxygen atom. Although hydrogen and oxygen individually are gases that support combustion, when combined into water, they have entirely different, and in some ways opposite, characteristics - they can extinguish fire. The constant motion of these atoms and molecules becomes significant when we consider the broader implications. This movement affects the pressure that gases and fluids exert as their molecules collide with each other and their surroundings. It can influence bonding between particles, leading to change in phase from solid to liquid, liquid to gas, and vice versa. Understanding these kinetic features of atoms and molecules offer insights into macroscopic phenomena like diffusion and osmosis, which find importance in areas as diverse as baking bread and delivering drugs to specific cells in the human body. In a nutshell, Particle Theory transcends beyond a rudimentary teaching tool in science classes. The comprehensive understanding of atoms and molecules as fundamental components of matter, their structure, and their behavior, opens doorways to profound scientific applications. It serves as a basis for not only chemistry and physics but also for related disciplines like biology and engineering, bound together by this fundamental concept. The diverse structure of atoms and molecules coupled with their unique interactions, provides an endless source of scientific exploration, where discoveries continue to be made that stretch our current understanding of reality.
Subatomic Particles: Protons, Neutrons, and Electrons
In understanding the Particle Theory of Matter, it's essential to examine the primary building blocks of matter: protons, neutrons, and electrons, collectively referred to as subatomic particles. Under Particle Theory, matter is composed of tiny, naturally indivisible particles, and these subatomic entities are the smallest conceivable units of matter that retain their distinct properties. Protons, situated in the nucleus, shape the identity of an atom as they have a positive charge, and their number in a single atom defines the atomic number, which consequently ascertains the type of element. A singular characteristic of protons is their ability to transform into neutrons through beta decay, reflecting the underlying interconnectedness of subatomic particles. Neutrons, like protons, are housed in the atom's nucleus and play a critical role in the interaction and stability of the atom. They bear no electrical charge, a feature that enables them to act as a buffer between protons, mitigating the repulsive forces between these similarly charged particles. In a nuclide, if the number of neutrons changes while the protons remain constant, a different isotope of a chemical element is created. This highlights not only the structural but also the transformative role of neutrons in atomic composition. Electrons, in contrast, are negatively charged particles that orbit the nucleus in electron shells. Electron behaviour is a major determinant of an atom's chemical reactivity, given that they inhabit the outermost shells and partake in bond formation with other atoms. Consequently, electrons dictate the dynamism of an atom, in contrast to protons and neutrons, which primarily confer stability. Subatomic particles, in the context of Particle Theory, affirm the granular and convertible nature of matter. The properties of these particles and their interactions present a foundational microcosm in understanding the broader behaviour of matter. The proton's capacity to morph into a neutron illustrates this notion, reinforcing the concept of transmutable matter at a subatomic level. Moreover, the electron's role in bonding and reactions underscores the mutable interactions of matter in practical and observable phenomena. Understanding these particles is thus quintessential in grasping the Particle Theory's proposals about the innate character, structure, and transformative potential of matter.
Intermolecular Forces and Bonds
Intermolecular Forces and Bonds play a crucial role in shaping the Components and Structure of Matter according to the Particle Theory. These forces represent the interactions between molecules that keep them together, forming the basis of physical properties of a compound such as its melting point, boiling point, viscosity, and surface tension. In essence, they serve as the glue that holds different particles together in a substance. Intermolecular forces are of three primary types: dipole-dipole interactions, hydrogen bonds, and London dispersion forces. Dipole-dipole interactions occur between polar molecules which have a positive and negative side, leading to a mutual attraction. Hydrogen bonding, a special type of dipole-dipole interaction, occurs when a hydrogen atom is bonded to a highly electronegative element like nitrogen, oxygen, or fluorine, resulting in a stronger attractive force. Lastly, London dispersion forces arise due to temporary fluctuations in the electron distribution around a molecule, creating temporary positive and negative charges. Furthermore, the Particle Theory of Matter explains that the particles are constantly moving and vibrating, causing them to interact with each other. The strength of the intermolecular forces holds significant importance here, influencing the molecule's freedom of movement. Stronger intermolecular forces tend to limit the movement of particles in a substance, keeping them closer and resulting in a solid or liquid form. On the other hand, weaker forces allow particles to move more freely, often resulting in a gaseous state. These bonds and forces not just impact the state of matter but also guide the chemical reactions. For instance, they determine how easily a substance can be broken down or combined with another substance. Hence, understanding intermolecular forces is crucial to predict both physical and chemical properties of a substance. Moreover, there are cases where intramolecular forces, the forces within a molecule, come into play. These forces include ionic, covalent, and metallic bonds and they are typically much stronger than the intermolecular forces. Covalent bonds occur when atoms share electrons, while ionic bonds form when an atom gives an electron to another atom. Metallic bonds, on the other hand, happen when electrons move freely within a network of metal atoms. In conclusion, the intermolecular forces and bonds essentially contribute to the structure and properties of matter as suggested by the Particle Theory. They determine the shape, state, and behavior of different molecules and substances, making them a central part of any study of matter. Understanding these forces and bonds enrich our knowledge of why substances behave the way they do, forming a key part of our understanding of the world at a molecular level.
Applications and Implications of Particle Theory
Understanding the applications and implications of Particle Theory can offer insights into a myriad of areas - from providing a fundamental understanding of chemical reactions and processes, to making sense of the physical properties of matter and fueling technological advancements. Firstly, the theory offers a basis to comprehend how atoms and molecules interact, leading to different chemical reactions and processes. It provides a cogent explanation for why certain substances react differently under varying conditions. Secondly, Particle Theory assists us in understanding the distinct physical properties of matter. It elucidates why substances exist in different states and how factors like temperature and pressure influence these states. Lastly, it has significant implications for technological advancements. As researchers gain more understanding of particle behavior, this knowledge could possibly lead to the development of innovative technologies. As we delve deeper underneath the surface, the profound nature of Particle Theory in unfolding the mysteries of our universe becomes undeniably clearer. So, let's begin our exploration with an in-depth look at how Particle Theory underpins our understanding of chemical reactions and processes.
Chemical Reactions and Processes
Chemical reactions and processes provide a vast panorama of fascinating instances where the particle theory of matter is applicable. This theory, stating that all matter is made up of tiny, indistinguishable particles that are in constant motion, plays a vital role in expounding the nature of chemical interactions and transformations. When chemical reactions occur, they involve the rearrangement, combination, or separation of these microscopic particles. One classic example of this principle in action is the reaction between hydrogen and oxygen to form water. The hydrogen and oxygen atoms (the 'particles' in this context) rearrange themselves to create molecules of water, conforming to the core tenet of the particle theory. Additionally, the particle theory helps demystify the processes of diffusion and osmosis. Diffusion refers to the passive movement of particles from a region of high concentration to an area of low concentration. In contrast, osmosis pertains to the movement of water molecules across a semi-permeable membrane, again from an area of high concentration to one of low concentration. Both these processes are quintessential examples of the particle theory's applicability in explicating real-world phenomena. Further, the particle theory also explicates the reasons behind changes in states of matter. For instance, heating a solid provides energy to the particles, increasing their motion and leading to a state change from solid to liquid (melting). Conversely, when a liquid is cooled, the particles lose energy, their movement reduces, resulting in a state change from liquid to solid (freezing). This principle also illuminates the understanding of pressure in gases. According to the particle theory, particles are always moving and colliding with each other and their container's walls. These collisions impart a force on the walls of the container, which is defined as pressure. Lastly, the theory explains how catalysts function to expedite reactions. Catalysts, by providing an alternative reaction pathway with a lower activation energy, increase the number of particles with energy equal to or greater than the activation energy, thereby accelerating the reaction. In conclusion, the particle theory of matter offers a fundamental framework for understanding an array of chemical reactions and processes. By considering the world at the particle level, we can gain a more profound understanding of the changes in matter we observe. The theory has far-reaching implications, making it a cornerstone of scientific inquiries into the nature and behavior of matter.
Physical Properties of Matter
The universe, as we understand it, is primarily composed of matter which possesses physical properties; this is the essence of the Particle Theory of Matter. These physical properties range from mass to color, from state to temperature, and they have profound implications and applications for the world around us. Physical properties define what we see, what we feel, and how substances interact with each other, thereby forming the foundation of our observations and practical applications in various fields of science. In the realm of physics, for instance, the physical properties linked to particle theory are utilized in the development of advanced technologies such as in nuclear power plants which operate based on the principle of nuclear fission; a process that exploits the property of certain atomic particles to release enormous amounts of energy when split. Similarly, in the field of chemistry, the physical properties of matter are pivotal in predicting and explaining chemical reactions. Take the property of reactivity in some elements; this explains why certain substances interact more readily with others, thus aiding in the creation of countless compounds and materials essential for our everyday life. Furthermore, the physical properties of matter are integral to the functionality of everyday objects. For example, the rigidity or flexibility of an object depends on the arrangement and interaction of its constituent particles, which is in turn, governed by the particle theory of matter. Likewise, the functionality of life-saving equipment, such as an airplane’s black box, is highly dependent on the physical property of matter known as density. Specifically, the black box is designed in a way that it can survive intense crashes and high-pressure underwater conditions, since its density is higher than the sea water, allowing it to stay intact and not disintegrate or sink. In the technological realm, networking and computing functions are greatly influenced by the physical properties of particles. For instance, the high-speed internet that we enjoy today is an application of the principles of light (a particle-wave matter) propagation in fiber optics. Similarly, the storage and processing capacity of our computers and smartphones owe their efficiency to the particle properties of semi-conductive materials that they are built with. Lastly, the physical properties of matter play a crucial role in dealing with environmental and social issues. In dealing with climate change, the understanding of the properties of greenhouse gases, like methane and carbon dioxide, is key to combating global warming. Alternatively, the physical properties of particles also affect social issues, like world hunger. By comprehending how different types of soil (which is, essentially, comprised of various matter particles) affect plant growth, agricultural scientists can enhance food production. In conclusion, the physical properties of matter, derived from the particle theory, have far-reaching applications and pose significant implications in multifarious domains. Thus, understanding these properties is not just a scientific endeavor but, a fundamental approach to comprehend and interact with the world around us.
Technological Advancements Based on Particle Theory
In our exploration of the impact and utility of the particle theory of matter, we must delve into the most recent, groundbreaking achievements: the technological advancements based on this theory. Technological advancements based on particle theory are embodiments of the sheer extent to which our understanding of the microscopic universe of atoms and particles can shape and revolutionize our macroscopic world. With this grasp, we’ve built machines and algorithms replicating the interactions and behaviors of these particles, resulting in monumental leaps in technology. One of the most significant developments is quantum computing. Unlike traditional computers that operate on binary data (ones and zeros), quantum computers leverage the principles of superposition and entanglement, core tenets of quantum mechanics and particle theory, to store and manipulate 'qubits' that can exist in multiple states simultaneously. This allows quantum computers to perform complex calculations tremendously faster than conventional computers. Another noteworthy advancement is the nanoparticle-based future of medicine. Particle theory plays a central role in the development of nanotechnology supporting diagnostic and therapeutic applications. Controlled drug delivery, targeted cancer therapies, and highly sensitive imaging techniques are just a few implications of applying the particle theory to medicine. Particle accelerators are yet another technological marvel dependent on particle theory. These machines explore the world of particles at extremely high energies, recreating conditions that were present during the birth of the universe and offering crucial insights about its building blocks. They are integral to vast fields such as astrophysics, cosmology, and materials science. By no means does this exclude the development of new materials based on particle theory. Comprehension of atomic and molecular behavior has birthed a wave of materials with bespoke properties and precision enhancements. Examples range from novel semi-conductors leading to faster and smaller electronics, to sophisticated materials like graphene, famed for its remarkable strength and flexibility. Moreover, some intriguing research explores particle theory for the next generation of information storage. The future could potentially see data stored in the state of a single atom or even its arrangement in a molecule— a concept unfathomable without a proper grasp on particle theory. These advancements demonstrate the profound practical application of particle theory and its implications on our society. Embracing our understanding of the particle universe unravels a world of monumental technological advancements that have the potential to revolutionize sectors spanning across healthcare, computing, materials science, and information storage. As we persist in unearthing mysteries of the particle world, our technological capabilities expand, offering limitless possibilities for a future enriched by these profound scientific insights.