What Is Synapsis
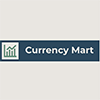
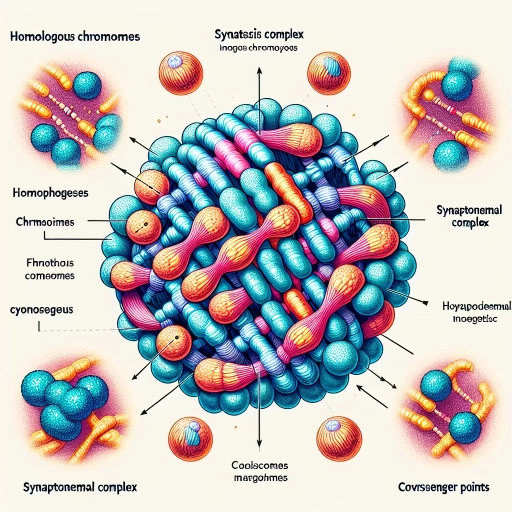
Synapsis, a fundamental concept in biology, plays a crucial role in the intricate processes of cell division and genetic inheritance. At its core, synapsis involves the precise alignment and pairing of homologous chromosomes during meiosis, a process essential for ensuring genetic diversity and the proper distribution of genetic material. This article delves into the multifaceted nature of synapsis, beginning with an exploration of its definition and context. We will examine the mechanisms and stages involved in the process of synapsis, highlighting the complex interactions that occur at the molecular level. Additionally, we will discuss the importance and implications of synapsis in biology, shedding light on its significance in maintaining genomic stability and facilitating evolutionary change. By understanding these aspects, we can gain a deeper appreciation for the critical role synapsis plays in the life cycle of organisms. To begin, let us first delve into the foundational concept: Understanding Synapsis: Definition and Context.
Understanding Synapsis: Definition and Context
Synapsis, a fundamental concept in biology, refers to the process where homologous chromosomes pair and align during meiosis, a critical phase in the reproductive cycle of organisms. This intricate biological mechanism is pivotal for ensuring genetic diversity and the proper distribution of genetic material. To fully grasp the significance of synapsis, it is essential to delve into its historical background, understanding how our knowledge of this process has evolved over time. Additionally, exploring the biological significance of synapsis reveals its crucial role in maintaining genomic integrity and facilitating recombination. Finally, familiarizing oneself with key terms and concepts related to synapsis provides a comprehensive framework for appreciating its complexities. By examining these aspects—historical background, biological significance, and relevant terminology—we can gain a deeper understanding of synapsis and its definition within the broader context of cellular biology. This article aims to provide a detailed exploration of these facets, ultimately leading to a thorough comprehension of synapsis: its definition and context.
Historical Background of Synapsis
The historical background of synapsis is deeply intertwined with the evolution of genetic and cytological studies, particularly in the late 19th and early 20th centuries. The term "synapsis" itself was coined by the American biologist Franklin Sumner in 1905, derived from the Greek word "synaptein," meaning "to clasp together." However, the concept predates this nomenclature. Early observations of meiotic processes were made by scientists such as Walther Flemming, who in 1882 described the condensation of chromosomes during cell division. This foundational work laid the groundwork for later researchers to delve into the specifics of chromosomal behavior. One pivotal figure in this narrative is Theodor Boveri, a German zoologist who in the early 1900s conducted extensive studies on sea urchin eggs. Boveri's work provided critical insights into the pairing and separation of chromosomes during meiosis, a process that would later be recognized as synapsis. His findings were complemented by those of E.B. Wilson and Nettie Stevens, who independently discovered sex chromosomes around the same period. These discoveries collectively illuminated the intricate mechanisms underlying genetic inheritance. The early 20th century saw a surge in research on meiosis and chromosomal behavior, with scientists like J.B.S. Haldane and R.A. Fisher contributing to the understanding of genetic recombination. The discovery of the synaptonemal complex by Don Fawcett in 1956 marked a significant milestone, as it provided a structural basis for understanding how chromosomes pair during meiosis. This complex, composed of proteins and nucleic acids, forms between homologous chromosomes and facilitates the exchange of genetic material through crossing over. The advent of molecular biology and advanced imaging techniques further elucidated the molecular mechanisms underlying synapsis. Studies using electron microscopy and later, super-resolution microscopy, have allowed researchers to visualize the synaptonemal complex in unprecedented detail. Additionally, genetic studies in model organisms such as yeast and mice have identified key proteins involved in synapsis, such as those forming the central element and lateral elements of the synaptonemal complex. Today, our understanding of synapsis is not only grounded in its historical context but also enriched by ongoing research into its molecular and functional aspects. This historical journey from early cytological observations to modern molecular insights underscores the dynamic nature of scientific inquiry and highlights the importance of synapsis in ensuring accurate genetic recombination during meiosis. As research continues to unravel the complexities of this process, it becomes increasingly clear that synapsis is a critical component of reproductive biology, essential for maintaining genetic diversity and ensuring the integrity of the genome.
Biological Significance of Synapsis
Synapsis, the process by which homologous chromosomes pair and align during meiosis, holds profound biological significance that underpins the very fabric of genetic diversity and reproductive success. At its core, synapsis ensures that each gamete (sperm or egg) receives a complete and balanced set of chromosomes, thereby maintaining the integrity of the genome across generations. This precise alignment is crucial for the proper segregation of chromosomes during meiosis, preventing aneuploidy—a condition where cells have an abnormal number of chromosomes—which can lead to developmental abnormalities, miscarriages, or birth defects. During synapsis, the formation of the synaptonemal complex—a protein structure that bridges the paired chromosomes—facilitates the exchange of genetic material through crossing over. This recombination process introduces genetic diversity by shuffling alleles between homologous chromosomes, thereby increasing the variability of offspring. This increased genetic diversity is vital for the adaptation and evolution of species, as it provides a broader range of traits that can be selected for or against by environmental pressures. Moreover, synapsis plays a critical role in ensuring that genetic material is accurately replicated and distributed. The pairing of homologous chromosomes allows for the detection and repair of DNA damage through mechanisms such as homologous recombination repair. This ensures that any errors in DNA replication are corrected before the chromosomes are separated, thus maintaining genomic stability. In addition to its role in meiosis, synapsis has implications for understanding various biological phenomena. For instance, studies on synapsis have provided insights into the mechanisms underlying fertility issues and certain genetic disorders. Understanding how synapsis fails can help in diagnosing conditions like infertility or recurrent miscarriages, which may be linked to defects in chromosome pairing and segregation. From an evolutionary perspective, the ability to form synapses between homologous chromosomes is a conserved feature across many eukaryotic organisms, highlighting its fundamental importance in sexual reproduction. This conservation underscores the critical role that synapsis plays in ensuring the continuity and diversity of life. In summary, synapsis is not merely a mechanistic step in meiosis but a cornerstone of genetic integrity, diversity, and reproductive health. Its biological significance extends beyond the cellular level to impact the broader evolutionary landscape, making it a fascinating and essential area of study in genetics and biology.
Key Terms and Concepts Related to Synapsis
**Key Terms and Concepts Related to Synapsis** Understanding synapsis requires a grasp of several key terms and concepts that underpin this critical biological process. **Synapsis** itself refers to the physical pairing of homologous chromosomes during meiosis, a process essential for genetic recombination and the proper segregation of chromosomes into gametes. **Homologous chromosomes** are pairs of chromosomes that carry the same genes but may have different alleles, ensuring genetic diversity. **Meiosis**, the context in which synapsis occurs, is a specialized type of cell division that reduces the chromosome number by half, producing haploid gametes (sperm and eggs) from diploid parent cells. This process is divided into two main phases: **meiosis I** and **meiosis II**. Synapsis takes place during **prophase I** of meiosis I, where homologous chromosomes come together and form a **synaptonemal complex**, a protein structure that facilitates their alignment and recombination. **Recombination**, or crossing over, is another crucial concept related to synapsis. This process involves the exchange of genetic material between homologous chromosomes, increasing genetic diversity by shuffling alleles. The points where crossing over occurs are known as **chiasmata**, which are visible under a microscope as X-shaped structures. The **centromere**, a region on each chromosome where sister chromatids are joined, plays a significant role in ensuring proper chromosome segregation during meiosis. **Sister chromatids**, identical copies of DNA joined at the centromere, separate during meiosis II. **Bivalent** and **tetrad** are terms used to describe the paired chromosomes during synapsis. A **bivalent** consists of two homologous chromosomes paired together, while a **tetrad** refers to the four chromatids (two from each homologous chromosome) that are aligned in a specific order. Understanding these terms and concepts is essential for appreciating the intricate mechanisms of synapsis and its role in maintaining genetic integrity and promoting diversity. Synapsis is not just a mechanical process but a highly regulated and complex biological event that ensures the proper transmission of genetic information from one generation to the next. By grasping these key terms, one can better comprehend the significance of synapsis within the broader context of meiosis and its impact on heredity.
The Process of Synapsis: Mechanisms and Stages
Synapsis, a critical process in meiosis, involves the intricate alignment and pairing of homologous chromosomes, ensuring genetic diversity and proper segregation during cell division. This complex mechanism is pivotal for the accurate transmission of genetic material from one generation to the next. The process of synapsis can be dissected into several key stages, each with distinct mechanisms and biological significance. **Initiation and Recognition in Synapsis** marks the beginning, where chromosomes are brought together through specific molecular interactions. **Alignment and Pairing During Synapsis** follows, where homologous chromosomes are precisely matched and aligned along their lengths. Finally, **Resolution and Completion of Synapsis** ensures that the paired chromosomes are properly separated, ready for meiotic division. Understanding these stages is essential for grasping the broader context of synapsis. To fully appreciate the intricacies of this process, it is crucial to delve into the definition and context of synapsis, setting the stage for a comprehensive exploration of its mechanisms and stages. Transitioning to **Understanding Synapsis: Definition and Context**, we will explore the foundational aspects that underpin this vital biological process.
Initiation and Recognition in Synapsis
Initiation and recognition are crucial stages in the process of synapsis, a fundamental aspect of meiosis and mitosis where homologous chromosomes align and pair. **Initiation** begins with the formation of the synaptonemal complex (SC), a proteinaceous structure that facilitates chromosome pairing. This process is mediated by proteins such as Spo11, which introduces double-strand breaks in the DNA, and RecA-like proteins that facilitate the exchange of genetic material. The SC consists of two lateral elements and a central element, providing a scaffold for the alignment of chromosomes. **Recognition** involves the precise identification and matching of homologous chromosomes, ensuring that each chromosome pairs with its correct counterpart. This is achieved through a combination of genetic and epigenetic cues, including sequence homology and chromatin modifications. The recognition process is highly specific, allowing for the accurate pairing necessary for subsequent genetic recombination and segregation. During initiation, the SC starts to assemble along the lengths of the chromosomes, beginning at specific regions known as attachment sites or telomeres. This assembly is facilitated by proteins such as HOP2 and MND1 in yeast, which stabilize the initial interactions between chromosomes. As the SC extends, it brings homologous chromosomes into close proximity, enabling the recognition phase to proceed efficiently. In the recognition phase, various mechanisms ensure that only homologous chromosomes pair. For instance, in some organisms, specific DNA sequences or repetitive elements serve as recognition sites. Additionally, epigenetic marks such as histone modifications and DNA methylation patterns can influence chromosome pairing by creating favorable chromatin environments. The interplay between initiation and recognition stages is tightly regulated to ensure accurate and efficient synapsis. Dysregulation in these stages can lead to meiotic errors, resulting in aneuploidy or infertility. For example, mutations in genes involved in SC formation or chromosome recognition can disrupt proper pairing, leading to aberrant meiotic outcomes. Understanding the mechanisms of initiation and recognition in synapsis not only sheds light on the intricate processes of cell division but also has implications for human health, particularly in the context of reproductive biology and genetic disorders. In summary, the initiation and recognition stages of synapsis are critical for the precise alignment and pairing of homologous chromosomes. These stages involve complex molecular interactions and regulatory mechanisms that ensure accurate chromosome matching, which is essential for genetic recombination and proper chromosome segregation during meiosis. The precision and specificity of these processes underscore the intricate and highly regulated nature of synapsis, highlighting its importance in maintaining genomic integrity.
Alignment and Pairing During Synapsis
During the intricate process of synapsis, alignment and pairing are critical stages that ensure the precise matching of homologous chromosomes. This phase is a cornerstone of meiosis, the reproductive cell division process that results in the production of gametes with half the number of chromosomes as the parent cell. Alignment refers to the initial step where homologous chromosomes, each consisting of two sister chromatids joined at the centromere, begin to come together in a parallel fashion. This alignment is facilitated by the synaptonemal complex (SC), a proteinaceous structure that forms between the paired chromosomes and holds them together. Pairing, which follows alignment, involves the close association of homologous chromosomes along their entire length. The SC plays a pivotal role here as well, providing a scaffold for the chromosomes to adhere to each other. This close association is crucial for several reasons: it allows for genetic recombination through crossing over, which increases genetic diversity by shuffling genes between homologues; it ensures proper segregation of chromosomes during meiosis I; and it facilitates the repair of DNA damage by providing a template for homologous recombination. The precision of alignment and pairing is maintained by various molecular mechanisms. For instance, proteins such as HOP1 and ZIP1 in yeast, and their mammalian counterparts, are essential for stabilizing the SC and ensuring that only homologous chromosomes pair. Additionally, mismatch repair proteins can detect and correct any errors in pairing, ensuring that non-homologous chromosomes do not mistakenly pair. The alignment and pairing process is tightly regulated and involves a series of checkpoints to ensure accuracy. For example, in some organisms, the formation of the SC is dependent on the presence of double-strand breaks (DSBs) introduced by enzymes like Spo11. These DSBs serve as initiation sites for recombination and help drive the pairing process forward. In summary, the alignment and pairing stages during synapsis are meticulously orchestrated processes that are fundamental to the successful completion of meiosis. These stages not only ensure genetic diversity through recombination but also guarantee the accurate segregation of chromosomes, thereby maintaining genomic integrity across generations. The intricate molecular machinery involved underscores the complexity and importance of this phase in the broader context of synapsis and meiotic progression.
Resolution and Completion of Synapsis
**Resolution and Completion of Synapsis** The resolution and completion of synapsis are critical stages in the meiotic process, ensuring accurate chromosome segregation during gamete formation. Synapsis, the pairing of homologous chromosomes, is a complex process that involves the formation of the synaptonemal complex (SC), a proteinaceous structure that stabilizes the paired chromosomes. As synapsis progresses, the SC facilitates the alignment and recombination of homologous chromosomes, which is essential for genetic diversity and proper chromosome segregation. The resolution phase marks the disassembly of the SC, allowing the chromosomes to separate while maintaining the genetic exchanges made during recombination. This process is tightly regulated to prevent errors that could lead to aneuploidy or other chromosomal abnormalities. The dissolution of the SC is mediated by specific proteins that dismantle the transverse filaments and central elements, releasing the paired chromosomes. Following resolution, the completion of synapsis involves the stabilization of chiasmata, which are the visible manifestations of crossover events between homologous chromosomes. Chiasmata act as physical links that hold homologs together until the first meiotic division, ensuring that each gamete receives a complete set of chromosomes. The proper formation and resolution of chiasmata are crucial for maintaining genomic integrity and ensuring that meiosis proceeds correctly. During this final stage, additional mechanisms such as cohesion and condensation come into play. Cohesin proteins, which bind sister chromatids together, are essential for maintaining chromosome structure and ensuring that sister chromatids separate correctly during meiosis II. Chromosome condensation, facilitated by condensin complexes, further prepares the chromosomes for segregation by compacting them into visible structures. In summary, the resolution and completion of synapsis are intricate processes that involve the coordinated action of multiple proteins and structures to ensure accurate chromosome pairing, recombination, and segregation. These stages are pivotal in the meiotic cycle, as they set the stage for successful gamete formation and the transmission of genetic material from one generation to the next. Any disruptions in these processes can lead to meiotic errors, highlighting their importance in reproductive biology and genetic stability.
Importance and Implications of Synapsis in Biology
Synapsis, a critical process in meiosis, plays a pivotal role in the intricate dance of genetic recombination, chromosomal stability, and reproductive biology. At its core, synapsis involves the precise alignment and pairing of homologous chromosomes, a step essential for the proper segregation of genetic material during cell division. This complex mechanism not only facilitates genetic recombination, thereby enhancing genetic diversity, but also ensures chromosomal stability and integrity by preventing errors that could lead to chromosomal abnormalities. Furthermore, synapsis is crucial for reproductive biology and fertility, as it directly impacts the formation of viable gametes. Understanding the importance and implications of synapsis is vital for grasping its multifaceted roles in these biological processes. This article will delve into the role of synapsis in genetic recombination and diversity, its impact on chromosomal stability and integrity, and its relevance to reproductive biology and fertility. To fully appreciate these aspects, it is essential to begin with a clear understanding of what synapsis entails, which will be explored in the section "Understanding Synapsis: Definition and Context."
Role in Genetic Recombination and Diversity
Synapsis, the process by which homologous chromosomes pair and align during meiosis, plays a pivotal role in genetic recombination and diversity. This intricate mechanism is crucial for the shuffling of genetic material, ensuring that offspring inherit a unique combination of traits from their parents. During synapsis, the synaptonemal complex forms between the paired chromosomes, facilitating the exchange of genetic material through crossing over. This recombination event introduces new combinations of alleles, thereby increasing genetic diversity within a population. The precise alignment of chromosomes during synapsis ensures that crossing over occurs accurately, minimizing errors that could lead to genetic abnormalities. The significance of synapsis in genetic recombination cannot be overstated. By allowing for the exchange of segments between homologous chromosomes, synapsis enhances the mixing of genetic information, which is essential for adaptation and evolution. This process contributes to the creation of new genotypes, some of which may confer advantageous traits that enhance survival and reproductive success. Furthermore, genetic diversity generated through synapsis helps populations to better withstand environmental challenges and resist diseases, as a diverse gene pool provides a broader range of potential responses to changing conditions. In addition to its role in recombination, synapsis also ensures proper segregation of chromosomes during meiosis. The accurate pairing and alignment of chromosomes during synapsis are prerequisites for correct chromosomal segregation, which is vital for maintaining the integrity of the genome. Misalignment or failure in synapsis can lead to aneuploidy, a condition where cells have an abnormal number of chromosomes, often resulting in developmental abnormalities or infertility. The implications of synapsis extend beyond the individual organism to the population level. In ecosystems, genetic diversity fostered by synapsis can influence population dynamics and evolutionary trajectories. For instance, populations with higher genetic diversity are more likely to adapt to changing environments, thereby enhancing their resilience and long-term survival. This underscores the importance of synapsis as a fundamental biological process that underpins the health and adaptability of species. In conclusion, synapsis is a critical component of meiosis that drives genetic recombination and diversity. By facilitating the precise alignment and exchange of genetic material between homologous chromosomes, synapsis ensures the creation of new genotypes and enhances the adaptability of populations. Its role in maintaining genomic integrity and promoting evolutionary fitness makes synapsis an indispensable process in biology. Understanding the mechanisms and importance of synapsis provides valuable insights into the intricate processes that shape life on Earth.
Impact on Chromosomal Stability and Integrity
The impact on chromosomal stability and integrity is a critical aspect of synapsis, highlighting its pivotal role in ensuring accurate genetic transmission during meiosis. Synapsis, the process by which homologous chromosomes pair and form a synaptonemal complex, is essential for maintaining chromosomal stability. This intricate structure facilitates the precise alignment of chromosomes, enabling the exchange of genetic material through recombination and ensuring that each gamete receives a complete and balanced set of chromosomes. Without synapsis, chromosomes would not pair correctly, leading to errors in meiotic segregation. Such errors can result in aneuploidy, a condition where cells have an abnormal number of chromosomes, which is associated with various developmental disorders and diseases, such as Down syndrome and certain types of cancer. Moreover, synapsis plays a crucial role in the repair of DNA double-strand breaks, which are potentially lethal lesions that can arise during meiosis. The synaptonemal complex provides a scaffold for the recruitment of repair proteins, facilitating homologous recombination and ensuring that these breaks are repaired accurately. This process not only maintains genomic integrity but also contributes to genetic diversity by introducing new combinations of alleles. The absence or failure of synapsis can lead to increased levels of unrepaired DNA damage, which may trigger cell cycle checkpoints and apoptosis, thereby protecting the organism from the propagation of genetically unstable cells. In addition to its role in meiosis, the principles underlying synapsis have broader implications for our understanding of chromosomal stability in somatic cells. Research on synapsis has provided insights into the mechanisms of chromosome pairing and recombination, which are relevant to understanding genomic instability in cancer cells. For instance, defects in the machinery that mediates chromosome pairing and recombination can lead to genomic rearrangements and mutations that drive tumorigenesis. Therefore, understanding the molecular mechanisms of synapsis is not only crucial for elucidating the intricacies of meiotic processes but also for developing therapeutic strategies to combat diseases associated with chromosomal instability. In summary, the impact of synapsis on chromosomal stability and integrity underscores its importance in maintaining accurate genetic transmission and preventing genomic disorders. By ensuring proper chromosome pairing and facilitating accurate recombination, synapsis safeguards the integrity of the genome, thereby contributing to the health and fertility of organisms. This fundamental biological process has far-reaching implications for our understanding of genetic diseases and the development of novel therapeutic approaches to address them.
Relevance to Reproductive Biology and Fertility
Synapsis, the process by which homologous chromosomes pair and align during meiosis, holds profound relevance to reproductive biology and fertility. This intricate mechanism is crucial for ensuring genetic diversity and the proper segregation of chromosomes during gamete formation. In the context of reproductive biology, synapsis facilitates the exchange of genetic material through crossing over, which enhances genetic variability and contributes to the adaptability of offspring. This genetic recombination is essential for maintaining the health and resilience of populations by introducing new combinations of alleles that can confer advantageous traits. Moreover, synapsis plays a pivotal role in fertility by ensuring that each gamete receives a complete and balanced set of chromosomes. Any disruptions in this process can lead to aneuploidy, a condition where gametes have an abnormal number of chromosomes, which is a common cause of infertility and miscarriages. For instance, errors in synapsis can result in nondisjunction events, where chromosomes fail to separate properly, leading to conditions such as Down syndrome or Turner syndrome. Therefore, understanding the mechanisms and importance of synapsis is vital for diagnosing and treating fertility issues related to chromosomal abnormalities. Additionally, research into synapsis has significant implications for assisted reproductive technologies (ART). By elucidating the molecular pathways involved in chromosome pairing and recombination, scientists can develop more effective strategies for improving the success rates of in vitro fertilization (IVF) and other fertility treatments. For example, insights into how synapsis is regulated could help in the development of novel diagnostic tools to assess the quality of oocytes and spermatozoa before they are used in ART procedures. In summary, synapsis is a cornerstone of reproductive biology, underpinning both the genetic diversity and the chromosomal integrity necessary for successful reproduction. Its importance extends beyond basic biological processes to have direct implications for human fertility and the advancement of reproductive technologies. Understanding and optimizing synapsis can thus contribute significantly to improving reproductive health outcomes and enhancing our ability to support individuals facing fertility challenges.